Final report for FNE22-001
Project Information
There are myriad options for farmers to amend soil to support crops and soil ecosystems. In this study the soil amendment Indigenous Microorganisms (IMO), a recipe for which is given in Korean Natural Farming, is compared to hot compost, municipal compost, and the mycorrhizal inoculant MycoGrow® Soluble (Fungi Perfecti, LLC). Chemical nutrient analysis, soil food web microscopy, and identification of plated bacteria and fungi cultures provide a general overview of each amendment’s properties and conditions. These properties are also compared at three stages of the IMO production process to better understand its biological dynamics. Labor and costs for each of the three amendments are also compared to assist farmer decision-making. Wheat bran-derived IMO is compared to the traditional use of rice bran in order to adapt the Korean methodology to the Northeastern US. Results show poor nitrogen and organic matter but comparable phosphorus, potassium, and salt levels in IMO relative to composts. Food web microscopy and laboratory identification of bacteria and fungi reveal IMO’s anaerobic properties and potential pathogens (oomycetes), properties at least partially attributable to excessive moisture called for in the IMO recipe. IMO proved to be more expensive and less labor intensive than hot compost, though farmers may choose less labor intensive methods of composting. MycoGrow® was shown to possess negligible nutrition and fewer fungal propagules than advertised. It is suggested that further research alter the IMO recipe and/or look more thoroughly into the biology of the IMO process in order to cultivate more beneficial organisms. Finally, the similarity between wheat bran-derived and rice bran-derived IMO warrants optimism about the adaptation of Korean Natural Farming to other climates. Results and commentary were shared via direct education of farm interns, two online publications, a podcast interview, and a hub website created for all these materials.
This project seeks to:
- Analyze chemical nutrient content and microbiological community dynamics throughout the stages of Indigenous Microorganism (IMO) production.
- Compare chemical nutrients and microbiology of top-dressing ready IMO to farm-produced and municipal compost, and the commercial mycorrhizal fungi inoculant MycoGrow® Soluble (Fungi Perfecti, LLC).
- Track and compare cost and labor for each of the amendment methods.
- Compare rice bran-derived IMO with wheat bran-derived IMO, which is more locally appropriate to the Northeast.
- Share theory, methods, results and recommendations in multiple agricultural audiences via direct education, written articles, and a podcast.
The use of microbial inoculants could reduce the need for fertilizers since soil microorganisms act to make nutrients available to plants, moderate delivery of nutrients to roots, and recycle decaying organic matter. Microbe-plant relationships can also increase drought tolerance, reduce disease and pest pressure, and attenuate plant stress (Lowenfels and Lewis, 2010; Bellows et al., 2020, Jacoby et al. 2017). The global agricultural inoculants market, which was worth $868 million in 2019, is projected to rise to $1.94 billion by 2027 (Fortune Business Insights, 2020). There are an increasing number of products available to the farmer, but their success depends on factors such as target soil conditions and competition with resident microbes (Bellows et al., 2020). This problem may not exist for organisms adapted (or “indigenous”) to a farm’s unique conditions.
Korean Natural Farming (KNF), a curriculum pioneered by Dr. Cho Han-Kyu, includes a technique to gather and inoculate indigenous microorganisms (IMOs) in under a month. The goals of KNF include improving soil quality, enhancing fertility, and boosting plant health all while using locally available materials (Reddy 2011). However, evidence for the effectiveness of IMO is still largely anecdotal, and studies attempting to correlate IMO with improved plant outcomes have variable results (Liao et al. 2019; Llamelo et al. 2016; Sakimin et al. 2017; Wang et al. 2012; Zuraihah et al. 2012). A study in Hawai’i showed that plant growth outcomes and the abundance of plant growth-promoting bacteria (Bacillus subtilis and B. licheniformis) in IMO-treated soils depended on the initial collection site of the IMO (Keli’ikuli 2018). SARE-funded research (Sustainable Agriculture Research and Education) attributed wheat growth benefits to the IMO compost carrier rather than the IMO microbes themselves (Englander 2013). Another SARE study observed no appreciable effects of IMO on plant yield despite an increased resistance to basil downy mildew with IMO treatment (Wright 2019). A third study observed increased leaf nutrient content for lettuce in IMO-inoculated soils, though differences in the soil microbiome were not detected with phospholipid fatty acid analysis (Roberts 2021).
More information is needed to optimize the IMO method for the Northeastern US and help farmers evaluate investments of time and money. One goal of this study was to trial the production of IMOs using wheat bran, which is cheaper and more locally accessible in the northeast than the rice bran of the original IMO recipe. Furthermore, although the potential benefits, scalability, and supposedly cheap cost of IMOs are enticing, composts and commercial inoculants represent other options, the costs and labor of which have yet to be compared to IMO directly. This study will compare chemical (nutrient) and biological properties of rice bran-produced IMO, wheat bran-produced IMO, municipal compost, farm-produced compost, and a commercial mycorrhizal fungi inoculant product (MycoGrow® Soluble, Fungi Perfecti LLC) while also tracking labor and financial costs of each method. Rather than studying effects on plant growth, which can vary with crop type and underlying soil properties, the focus was instead to provide basic information on each material. Specifically, this study will analyze physio-chemical composition using standardized compost analysis, microbial population dynamics with Soil Food Web microbiome assessments, and identify key fungi and bacteria species using plate cultures from swabs. A comprehensive comparison of these properties should inform future research as well as farmers evaluating soil amendment options.
Founded by millennial first-generation farmers who met through the international WWOOF network, Unadilla Community Farm is an off-grid fruit & vegetable farm and non-profit permaculture education center, situated on 12 acres of field and forest in West Edmeston, in central New York State. Our mission is to provide a space for the teaching and practice of sustainable skills. Our work centers on providing education and training in sustainable agriculture and natural building, and providing access to fresh produce for low-income and low-access communities. Founded in 2014, Unadilla Community Farm Education Center Inc became incorporated as a 501(c)3 non-profit organization in 2020.
We are showcasing a range of cutting-edge sustainable agriculture practices. Our off-grid center grows 200+ varieties of annual and perennial cold-hardy fruits, vegetables, mushrooms, and herbs. We are regenerating the land after decades of conventional monocropping, transforming an abandoned corn field into an edible food forest. As a center for sustainable education and a member of USDA’s Conservation Stewardship Program and New York State’s Climate Resilient Farming Program, we showcase a diversity of USDA NRCS recommended conservation practices, such as rainwater collection, multi-story and alley cropping, no-till management, wildlife habitat planting, heavy mulching, on-site composting, crop rotation, and high tunnels.
The farm’s primary 2 programs are its veggie box delivery program and beginning farmer training program.
This season, we produced 2,443 lbs of veggies, 53% of which was donated to food pantries in Otsego, Oneida, and Herkimer counties. In addition to delivering to food pantries, we also delivered weekly veggie boxes from June-October directly to families in the Edmeston area — a USDA low-income, low-access food desert, meaning an area with high poverty rates where the nearest supermarket is over 10 miles away and a significant number of households don’t have a vehicle. Boxes for families are priced on a sliding scale — starting at $0, no questions asked — delivered directly to families’ doorsteps at no cost.
2022 was our 9th season welcoming interns for our accredited beginning farmer training program, teaching skills related to no-till organic farming, regenerative agroforestry, permaculture design, natural building, food preservation, plant-based cooking, and more. We offer an immersive residential program with a minimum stay of 1 month, focused on hands-on experiential learning. This season, we hosted 27 interns from across the country and around the world, including from the countries of France & Lebanon. At least 4 interns received academic credit at the undergraduate or graduate level through academic institutions, such as Bowdoin College in Maine, Kalamazoo College in Michigan, and École d'ingénieurs de Purpan in France, for completing our program.
Matt Bedeaux is Unadilla Community Farm's 2022 Research Fellow. Matt is a 2021 alumnus of the farm’s internship. Throughout the 2022 season, Matt trained interns in Unadilla Community Farm’s Beginning Farmer Training Program and engaged them in the on-farm research project studying indigenous microorganisms.
Cooperators
- - Technical Advisor (Educator and Researcher)
Research
IMO and compost were produced at Unadilla Community Farm in West Edmeston, NY during July and August 2022. One-half inch screened municipal compost was purchased and delivered in April 2022 from Onondaga County Resource Recovery Agency in Jamesville, NY. Wood chips used in the farm-produced compost were purchased and delivered in May 2022 from Clifton Recycling in Syracuse, NY. Water used to moisturize IMO and compost was collected from a creek that runs through the farm. Creek water was not characterized. For monitoring culture conditions, a Lostronaut® compost thermometer and an Inspect USA® soil moisture meter were used. The soil moisture meter provides live, 0.1%-precision moisture readings but only measures up to 50%, beyond which the monitor displays an error. For the experiments described below, confirmation of >50% moisture was satisfactory. Materials used to produce IMO are listed in detail in Table 1 at the end of this section.
The commercial inoculant selected for this study is MycoGrow® Soluble Mycorrhizae (Fungi Perfecti, LLC), 1 lb of which was purchased. This product was selected due to its popularity and since IMO was hypothesized to contain beneficial fungi. According to the product’s website, MycoGrow® Soluble Mycorrhizae (henceforth: MycoGrow) contains 92,000 propagules of endomycorrhizal fungi per pound of the product, including Glomus intraradices, G. mosseae, G. aggregatum, G. etunicatum (34 prop/g each); and Glomus deserticola, G. monosporum, G. clarum, Paraglomus brasilianum and Gigaspora margarita (13 prop/g each). The product is also advertised to contain 1.2 billion propagules of ectomycorrhizal fungi per pound, including Rhizopogon villosulus, R. luteolus, R. amylopogon, R. fulvigleba (208,750 prop/g each); Pisolithus tinctorius (1,250,000 prop/g); Suillus granulatus (260,000 prop/g), Laccaria bicolor and L. laccata (83,500 prop/g each); and Scleroderma cepa and S. citrinum (41,750 prop/g each, Fungi Perfecti 2022). Identification of fungal propagules was not performed in this study since mycorrhizae need root masses to grow, and other identification experiments were performed on plates. However, verification of the total endo- and ectomycorrhizal propagule counts was attempted using microscopy as described in Analysis and Sampling.
IMO Overview
After several troubleshooting trials, one batch of rice bran IMO and one batch of wheat bran IMO were produced using the following methodology adapted from Reddy 2011. The IMO recipe occurs in four successive stages. IMO1 is made by placing a wooden box filled with cooked white rice in the wilderness for several days. Once the rice is completely covered with white mold, it is combined with an equivalent volume of brown sugar and allowed to incubate in a glass or ceramic jar for no more than three days. This mixture, which is called IMO2, enters the IMO3 stage by being diluted with water and KNF-derived fermented plant juice (FPJ) and used to inoculate bran which is allowed to sit for approximately 1 week, or until the IMO3 is completely colonized with white mold. The final phase of IMO production, IMO4, involves mixing the cultured bran with an equivalent amount of soil and allowing another two days. At this point the IMO4 may be applied as top dressing or via foliar spray (O’Hara 2017; Zuraihah et al. 2012). Amendment was not performed in this study.
IMO Production
IMO1. A box was constructed with 0.75 inch thickness hemlock whose internal dimensions were 12 inch x 8 inch x 4.5 inch. The lid was made to be a metal screen. White rice was prepared to be slightly dry (4:7 rice to water ratio). The wooden box was filled three quarters with rice. During the initial investigations of this project, it was discovered that cheesecloth being placed directly on the rice, rather than over the exterior of the box, led to more prolific molding and protected the cheesecloth from animals. The IMO collection site in a nearby forest (deciduous stage) was chosen with attention to plant diversity, tree age, moisture, and fruiting fungi. Soil samples were collected for analysis immediately before placing the box. The box was then half-buried at the collection site and soil debris was placed on top. A small plastic tarp was used to protect from rain. During initial investigations it was found that the IMO1 is fully colonized by white mold, with early signs of black mold, in 3 to 6 days. The experimental trial IMO1 was removed after four days.
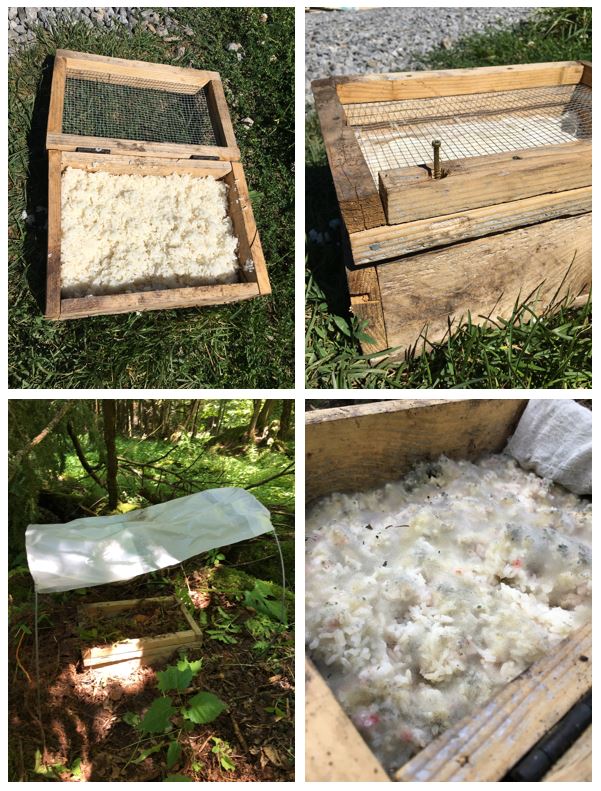
IMO2. The rice box was removed from the collection site and the rice was then mixed 1:1 by volume with organic brown sugar in a glass jar. Large clumps of rice were broken up with a spoon and the mixture was stirred well before being covered with a towel and tied with twine. The jar was then allowed to sit outdoors in dry, partial shade for 2 days.
FPJ. Fermented plant juice (FPJ) is an important ingredient used in the IMO3 mixing recipe. To prepare FPJ, in sunny weather during the late morning dandelions were pulled from soft, heavily mulched soil. Care was taken to pull dandelions from shady areas and to keep the collected plants in the shade while processing. Some soil was shaken off, though the material was not washed as surface microorganisms are desired. Plant material was chopped into 1-2 inch sections, with roots being chopped in 0.5-1 inch sections. Chopped dandelions (5.0 oz) were mixed 2:1 by mass with brown sugar. Two tbsp creek water was added to improve brown sugar distribution on the leaf surfaces. The bowl was allowed to sit under a towel for 2 hours in the shade before being transferred to a clean quart-sized glass jar. A stone was placed on top of the mixture to weigh it down, the top was tied with cheesecloth and the jar was stored in the shade for eight days before use in the IMO mixing process. The stone was removed from the jar on the fifth day to allow more air infiltration. The fermentation was timed to be ready at the IMO3 mixing stage, when FPJ is needed.
IMO3. After two days in the jar, IMO2 was added to a ~1:1000 dilution of FPJ in creek water (5 tsp in 5 gal). IMO2 was then diluted 1:500 in the FPJ/creek water mixture (10 tsp in 5 gal). Then, 40 lbs of rice bran were poured onto the IMO3 culturing bed and 5 gallons of the IMO2-FPJ dilution was added to the pile and mixed with the bran. The moisture content was tested to be >50%, and the initial temperature was 22 °C. The bran was piled to 2-5 inch tall on bare soil in a shady area at a forest edge. The same methodology was repeated for wheat bran. That is, 5 gallons of the same IMO2-FPJ mixture was added to 40 lbs of bulk wheat bran which was cultured adjacent to the rice bran. The pitchfork used to stir was wiped to avoid contamination between bran types. The wheat bran’s initial moisture and temperature were >50% and 23 °C. The piles were covered with plain, black-ink-only cardboard and weighed with rocks. Temperature and moisture were measured twice a day for 7 days by uncovering the cardboard and inserting the probe into different locations in the pile. It is desired that the moisture content of the piles is such that the bran clumps when pressed and loosens when shaken. It was observed that the rice bran IMO was initially too wet (mushy consistency). Thus the rice bran IMO was left uncovered for the first two days. For future experiments, it is advised to add only as much water as is needed to create the clumps/loosens consistency, rather than the arbitrary five gallons used in this study. The extra moisture in the rice bran IMO, and being uncovered for two days, led to a slower progression of temperature than the simultaneous wheat bran IMO whose moisture content was satisfactory since the wheat bran is more absorbent. The IMO piles were turned and saltwater (30 parts per thousand mineralized sea salt in creek water) was added after 3 days to mix and re-moisturize them. In accordance with Dr. Cho’s recipe, saltwater was used instead of fresh water to increase mineral nutrient content. One gallon of saltwater was added evenly to the rice bran IMO, and 2 gallons were added to the wheat bran IMO. Only one saltwater addition was needed to keep the moisture content >50% for both batches as desired. After seven days, the rice bran IMO finished at 35 °C, whereas the wheat bran IMO finished at 42 °C.
IMO4. Each IMO3 batch was mixed 1:1 by volume with topsoil (screened to 1 inch). The moisture content after mixing was measured to be 11%-22%. Therefore, 2 gallons of saltwater (30 ppt) were added after the IMO was thoroughly mixed. Moisture and temperature continued to be measured twice a day for another two days before the IMO4 was sampled.
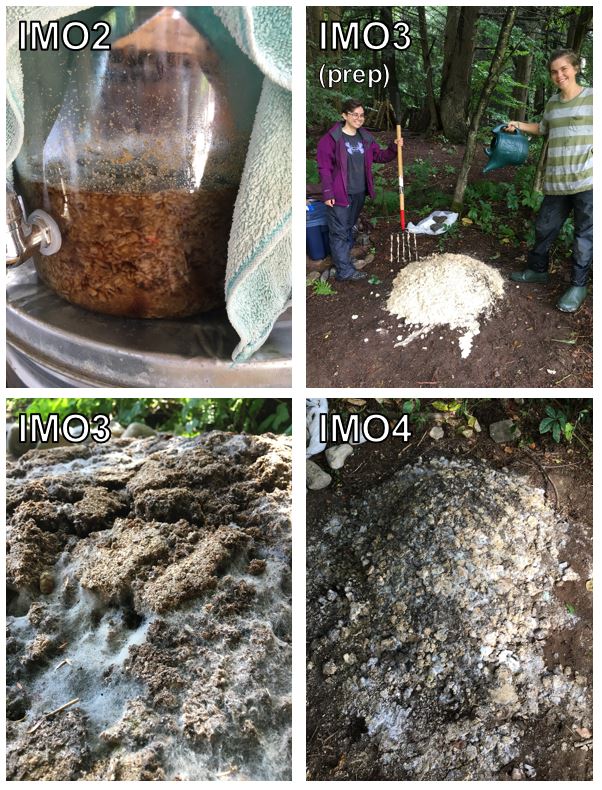
Compost
The ‘Berkeley’ hot composting method was selected so that the compost would finish in the same timeframe as IMO. The method is advertised to work within 18 days (Eliades 2010). This method utilizes a low carbon to nitrogen ratio, high water content, and involves turning the pile every other day after the initial, static four days. Green material consisted of yard clippings from the farm and brown material consisted of commercial wood chips. Grass and weed clippings were collected during two consecutive days via mowing. Full-length hand-pulled weeds and root masses were collected and chopped into 6-8 inch sections during the same time. Based on an initial compost trial in which the temperature exceeded the desired range of 55 °C-65 °C, it was surmised that the C:N ratio of the grass clippings was approximately 20:1. Therefore, 50 gallons of wood chips were combined with 1 cubic yard of grass clippings over the course of the first five days to attempt to reach a C:N ratio between 25:1 and 30:1, the ideal range for this method. The temperature and moisture content of the compost were measured at least twice a day for the first 10 days, then once a day thereafter. Moisture was measured in three locations at a depth of ~1 ft. When moisture content was measured to be lower than 50% in any location, 5 gallons of fresh water were added to the compost within 24 hours. Since the pile was in full shade, moisture stayed relatively constant. The pile was not turned for the first four days, then it was turned every other day until finished. The compost was also screened every four days to separate clumps, though no materials were removed during the screening process. This “rough screening” made a finer consistency. During the first five days of composting, the pile reached temperatures greater than 70 °C, indicating a low C:N ratio. Despite additions of wood chips and turning, the equilibrium temperature was above 70 °C for the first seven days. This likely indicates that the grass clippings had a lower C:N ratio than presumed. The compost did not cool to below 55 °C until day 13. The pile then slowly cooled to a final temperature of 33 °C on day 24, at which time the compost was nearly totally decomposed and sampled for analysis. Municipal compost was also sampled and analyzed to provide data on a lower temperature, less labor-intensive composting process.
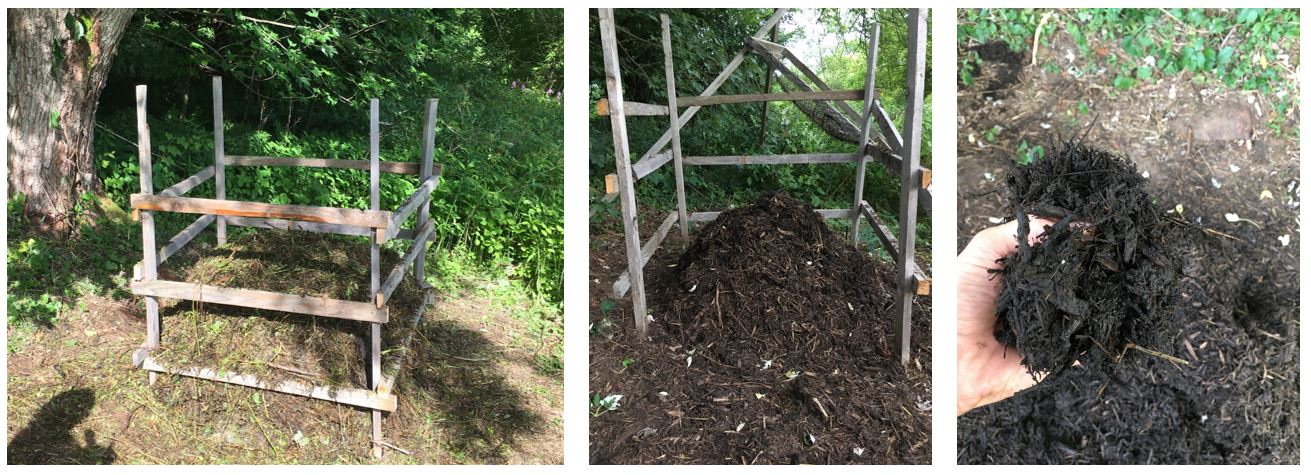
Analysis and Sampling
Samples were collected throughout the IMO production process and at the topdressing-ready stage for composts and MycoGrow. Samples were collected in plastic bags with a stainless steel corer and/or a hand trowel which were cleaned using soap and water and sanitized with isopropyl alcohol between each sample collected. Samples were shipped on ice to respective labs for analysis. Nutrient analyses were provided by Pennsylvania State University’s Agricultural Analytical Services Laboratory (AASL). Nutrient analyses were performed using the lab’s “Compost 1B” test, which includes percent solids (SM 2540 G, American Public Health Assoc 1992), organic matter (TMECC 5.07-A, USDA and US Composting Council 2002), pH (TMECC 4.11-A), soluble salts (TMECC 4.10-A), total nitrogen (TMECC 4.02-D), total carbon (TMECC 4.02-D), Carbon:Nitrogen ratio, ammonium nitrogen (TMECC 4.02-C), phosphorus (TMECC 4.03-A) and potassium (TMECC 4.04-A). One sample was additionally submitted for AASL’s Soil Fertility Test, which includes aqueous pH (Eckert and Sims 2011), phosphorus (Wolf and Beegle 2011), potassium (Wolf and Beegle 2011), magnesium (Wolf and Beegle 2011), calcium (Wolf and Beegle 2011) by the Mehlich 3 (ICP) test, as well as nitrate content (Griffin et al. 2011), total nitrogen content (Bremner 1996), and soluble salts (Gartley 2011). Microbiome assessments were provided by Web of Life Regenerative Landcare in Ithaca, NY. The microbiome assessment utilizes a 1 ml (~1 g) sample of soil, which is diluted 1:10 with water, 1 drop from which is placed under a 18mm square cover slip and examined using a shade microscope at 400x magnification. Fifteen to 25 fields of view are then examined, organisms from which are categorized and counted. The counts are averaged and scaled using dilution parameters to provide estimates and standard deviations of total bacterial biomass, fungal biomass, actinobacterial biomass, counts of flagellates, amoebae, ciliates, and oomycetes. The total number of bactivorous, fungivorous, predatory, and root-feeding nematodes in the droplet are counted and thus this measurement has no associated variability. Bacteria and fungi identification analyses were provided by EMSL Analytical, Inc. in Cinnaminson, NJ. Swabs provided by EMSL were collected and returned to EMSL for analysis. Samples were then cultured for two weeks and the 3 most prominent bacteria cultures were identified and enumerated to the species level. Similarly, fungi are cultured, identified, and enumerated at the genus level from submitted swabs. No reference is provided for these identification methodologies as EMSL’s experiments are proprietary.
The forest soil was sampled at the site of IMO1 collection, which was sent to Penn State for the Compost 1B and the Soil Fertility tests described above. No other samples were submitted for the Soil Fertility test. For each of the samples collected at the IMO1 stage, five to ten 3 inch cores were taken variously within a 10 ft radius surrounding the location in which the rice box was placed. The same box of rice (IMO1) was used, and the same batch of rice-brown sugar mixture (IMO2) was used to inoculate rice bran and wheat bran which went on to be separate and simultaneously produced IMO4’s. Therefore, only one sample was needed at the IMO1 stage and the IMO2 stage. IMO2 was sampled after inoculating both the rice bran IMO3 and wheat bran IMO3. The IMO2 mixture, which consisted of liquified brown sugar and white rice, was stirred and poured into sampling containers. The next samples were collected at the end of the IMO4 stage, in which five full-depth cores were collected directly into the sampling bag. For samples of the municipal compost, five 3 ft holes were dug with a hand trowel at different areas of the large pile, material from which was mixed into a five gallon bucket, from which samples (approx. 1 quart each) were collected. For samples of the farm-produced compost, five trowel scoops were collected at various depths and sides of the pile and directly placed into sampling bags. MycoGrow was sampled by pouring the product directly into a sampling bag. MycoGrow was submitted for nutrient analysis and microbiome assessment, but not for identification. All swab samples were collected from the same locations from which material was removed for other samples. Sterile swabs and containers were provided by EMSL Analytical, Inc.. For all samples, bags were filled approximately halfway to permit aeration during travel. They were kept in shade and put on ice within an hour of being collected. Samples were sent in well-padded cardboard boxes to their respective labs for analysis. Samples from the IMO1 site (referred to as “forest soil”) were sent via two day shipping, whereas all others were sent overnight.
Materials, Costs, and Labor
Table 1 shows the relevant materials used for producing IMO and compost on site. Analysis of costs and labor was performed for a fixed top-dressing application area of 100 square feet at 1 inch depth. To perform this comparison, the total volume of 1 batch of IMO and 1 batch of farm-produced compost were measured to be approximately 6 cubic feet each. Material was compacted into 5-gallon buckets by filling the bucket partially, dropping the bucket onto flat ground ten times, and refilling and dropping ten times repeatedly until the bucket was full. When compacted, each batch was measured to be 10 gallons. The resulting calculated pore space of 78% was used for all IMO and compost materials. MycoGrow application density is equated to the manufacturer’s recommended rate of 1 lb per 4000 square feet. Bulk density is also used to compare weights of materials and components. Bulk density was calculated by weighing 5 gallons of compacted material. Analysis of cost, labor, and key chemical and biological properties per 100 square feet is presented at the end of the results and discussion section.
Material costs were tracked via receipts. Calculations do not include the cost of ordinary materials such as scrap wood. Cost also does not include the one-time startup $20.33 for IMO to purchase steel wire and plastic covering for rain protection. There were no startup costs for composts and MycoGrow. Cost for delivery of topsoil, wood chips, and municipal compost are excluded as these vary with mileage. Labor is calculated from records of hours worked each day, with multiplication for hours worked simultaneously by multiple people. Time for research tasks was subtracted. The labor figure does not include the time required to apply the amendment. Labor does not include time for startup tasks such as building the compost frame, building the IMO1 box, or preparing the IMO3 culturing site.
Amendment
|
Material | Amount needed per batch | Source Brand |
IMO | White rice | 8 cups dry | Dougets ® |
Brown sugar | 2 lbs | Field Day ® | |
Rice bran (Max-E-Glo horse supplement) | 40 lbs | Manna Pro ® | |
Wheat bran | 40 lbs | Star of The West ® | |
Mineralized sea salt | 1 lb | Champions Choice ® | |
Topsoil | 3 cu ft | Epic Landscapes ® | |
9-ga galv. steel wire | 10 ft | Farmgard ® | |
Plastic covering | 5 ft | Poly-Cover ® | |
Plain cardboard | 40 sq ft | n/a | |
Dandelions | 0.5 lb | Collected on farm | |
Farm-produced compost | Grass clippings, weeds, kitchen scraps | 1 cu yd | Collected on farm |
Wood chips | 50 gal | Clifton Recycling ® |
Nutrient Analysis
A farm’s nutrient needs vary with crop, soil type, growth stage, climate and weather, and other factors. Thus, no general advice may be given on any of the presented amendments, though comparison of nutrient amounts will deepen understanding of the IMO production process and its utility compared to other amendments. Amounts in Table 2 estimate the total mass of each nutrient via AASL’s Compost 1B tests. This test quantifies total nutrient amounts whether or not they are bioavailable. Mehlich 3 soil fertility analysis instead measures nutrient bioavailability, and reported 3.4% and 5.0% of the phosphorus and potassium amounts for the IMO1 collection site shown in Table 2 respectively, indicating the likelihood that small fractions of the masses reported in Table 2 are bioavailable. No other samples were submitted for Mehlich 3 analysis.
|
IMO1 Site (aka “Forest Soil”) | IMO2 | Rice bran IMO4 (RIMO4) | Wheat bran IMO4 (WIMO4) | Municipal Compost | Farm- | MycoGrow |
produced Compost | |||||||
pH | 6.8 | 5.9 | 5.8 | 6.5 | 8.1 | 7.9 | 6 |
Soluble salts (mmhos/cm) | 0.04 | 0.53 | 3.51 | 4.42 | 1.91 | 3.93 | 22.11 |
Est. soluble salts (ppm)* | <50 | 300 | 2650 | 3500 | 1450 | 3050 | >10,000 |
Solids (%) | 63.6 | 67.5 | 78.7 | 76.2 | 46.8 | 42.2 | 92 |
Moisture (%) | 36.4 | 32.5 | 21.3 | 23.8 | 53.2 | 57.8 | 8 |
Organic matter (%) | 12.3 | 99.9 | 13.5 | 9.8 | 41.5 | 78.7 | 51.6 |
Total nitrogen (%) | 0.481 | 0.25 | 0.433 | 0.429 | 1.338 | 2.024 | 0.827 |
Organic nitrogen (%) | 0.481 | 0.247 | 0.421 | 0.382 | 1.335 | 2.019 | 0.808 |
Ammonium (%) | 0.0002 | 0.0022 | 0.0126 | 0.0467 | 0.0015 | 0.0038 | 0.02 |
Carbon (%) | 6.9 | 39 | 7.5 | 6.3 | 25 | 26.1 | 30.8 |
C:N Ratio | 14.3 | 155 | 17.4 | 14.6 | 18.7 | 12.8 | 37.2 |
Phosphorus** (ppm) | 1,200 | 260 | 4,100 | 2,600 | 1,600 | 3,000 | 570 |
Potassium** (ppm) | 1,700 | 741 | 3,900 | 3,300 | 4,800 | 14,000 | 53,000 |
*determined using Scherer and Meehan 2019. ** P and K are given on an elemental (P and K, not P2O5 and K2O) basis. |
Measurements of pH in both IMO4 preparations were acidic, more so in the case of rice bran-prepared IMO4 (RIMO4), which agrees with the anaerobic odor observed when working with IMO and the especially moist conditions of RIMO4. Both municipal and farm compost have pH near 8, which is within the range of 6-8 expected for mature composts (Cornell 1996). Soluble salts, which is a general estimate of the total plant-accessible, inorganic nutrients, are lower in RIMO4 than in WIMO4 by approximately 1 part per thousand. This is expected since 3 gallons of saltwater were used in the RIMO production, whereas 4 gallons were used in the WIMO production. Soluble salts in both IMO4’s exceed that of municipal compost and are relatively comparable to the farm-produced compost.
Assuming IMO would be applied at the same density as compost, both IMO4 preparations have lower organic matter and carbon content relative to the two finished composts. This is likely due to finished IMO4’s containing approximately 50% inorganic topsoil. Both IMO4’s also have nitrogen amounts slightly under 0.5%, which is the low end of the optimal range for finished compost (AASL, 2020) and lower than either compost tested in this study. The IMO4’s have higher phosphorus than the municipal compost and comparable phosphorus to the farm-produced compost, and they have higher potassium than municipal compost and lower potassium than the farm-produced compost. In summary, lower organic material and somewhat low nitrogen make IMO less desirable as a replacement for compost, though soluble salts, phosphorus and potassium levels are comparable to the compost materials studied. If more nitrogen and/or organic matter is desired in IMO, I suggest using nutrient-rich soil in the IMO4 stage rather than the largely inorganic material used in this study.
Beyond the comparison of IMO to traditional compost methods, Table 2 also shows that the farm-produced compost has higher organic matter, lower C:N ratio, and more phosphorus, potassium, and salts than the municipal compost. These results are expected due to its relative freshness. It is also clear that IMO2 would be relatively ineffective as a nutrient amendment. It also appears that nutrients from the forest soil are not transmitted to the IMO2 stage, and the larger amounts of phosphorus and potassium in the IMO4 preparations than the IMO2 indicate that nutrient properties of IMO4 are primarily affected in the IMO3 and IMO4 stages. Interestingly, carbon, total nitrogen and organic matter amounts in the forest soil sample are similar to that of the IMO4’s. This poses questions about whether biological conditions in IMO4 resemble that of the original forest soil. This will be addressed in later sections.
Nutrient content of MycoGrow was analyzed using the same methodology as the other amendments. Results are presented for completeness. However, since MycoGrow is primarily a biological inoculant, its nutrient properties cannot be compared directly to the other amendment methods which serve to add nutrients as well as organisms. The comparison of MycoGrow is complicated further by the fact that it is diluted prior to application. The manufacturer recommends spraying MycoGrow diluted at 1 ounce per 12 gallons, and reports that a full pound of the product can be used to treat 4000 square feet (Fungi Perfecti 2022). However, studies of commercial inoculants highlight the complex relationship between application rate and degree of colonization for arbuscular mycorrhizal fungi inoculants (Benami et al. 2020, Tarbel and Koske 2007). The rate of spray or injection and its correspondence to successful colonization is beyond the scope of this study, but it is clear that a farm’s unique conditions will necessitate experimentation to determine an effective application rate.
Microbiome Assessments
Table 3 shows the results of the Microbiome Assessments. As is typical for the Soil Food Web methodology, large standard deviations were produced, some of which were greater than the corresponding mean. This occurs since organisms appear at variable concentrations across microscopic fields of view. Bacteria are typically evenly distributed across multiple fields of view, whereas fungi, protozoa and oomycetes may be concentrated in particular areas and absent from others. Since nematodes are counted in total, there is no associated variability (standard deviation) for these counts. The units of #/g are converted to ug/g for bacteria, fungi, and oomycetes using the Soil Food Web’s proprietary algorithm. In general, standard deviations that are less than 50% of their corresponding mean are assumed to be reasonably precise, whereas measurements with standard deviations greater than 70% of the mean are removed from discussion. While this method provides a glimpse into IMO biology, future studies may consider more detailed methods such as DNA sequencing.
|
Rec. soil range | Rec. compost range | Forest soil | IMO2 | RIMO4 | WIMO4 | Mun. Compost | Farm compost |
Fungal biomass (ug/g) | 675 - 9000 | 101 - 1012 | 2,794 ± 1,362 | 0 | 78 ± 173 | 243 ± 509 | 18 ± 28 | 764 ± 547 |
Bacterial biomass (ug/g) | 135 - 900 | 135 - 1350 | 6,656 ± 1,564 | 2420 ± 548 | 17,137 ± 2,598 | 21,693 ± 4,342 | 9,720 ± 2,813 | 5,089 ± 1,632 |
Actinobacteria (ug/g) | 4-Jan | 16-Jan | 1.86 ± 2.14 | 0.07 ± 0.16 | 1.88 ± 2.85 | 0 | 1.10 ± 1.34 | 11.33 ± 4.28 |
F: B ratio | 5 - 100 | 0.6 - 0.9 | 0.42 | 0 | 0.0046 | 0.011 | 0.0019 | 0.15 |
Total protozoa (#/g) | >10,000 | >50,000 | 220,000 ± 200,000 | 0 | 0 | 0 | 65,216 ± 145,827 | 3,187,432 ± 871,331 |
Flagellates (#/g) | see total | see total | 220,000 ± 200,000 | 0 | 0 | 0 | 0 | 588,982 ± 289,869 |
Amoebae (#/g) | see total | see total | 0 | 0 | 0 | >0* | 65,216 ± 145,827 | 2,598,450 ± 612,461 |
Ciliates (#/g) | see total | see total | >0* | 0 | 0 | 0 | 0 | 0 |
Bactivorous nematodes (#/g) | ~300 | ~200 | 0 | 0 | 0 | 320 | 160 | 1,870 |
Fungivorous nematodes (#/g) | ~300 | ~100 | 0 | 0 | 0 | 0 | 0 | 170 |
Predatory nematodes (#/g) | ~200 | >0 | 0 | 0 | 0 | 0 | 0 | 0 |
Oomycetes (ug/g) | 0 | 0 | 0 | 772 ± 694 | 22,484 ± 3,967 | 2,235 ± 3,002 | 0 | 394 ± 413 |
Root-feeding nematodes (#/g) | 0 | 0 | 0 | 0 | 0 | 0 | 0 | 0 |
*a single organism of this type was observed. |
In general, fungi and bacteria provide an array of benefits to the soil ecosystem including nutrient mineralization and cycling, recycling and decomposing organic matter, increasing drought tolerance, reducing pest pressure, and attenuating plant stress (Lowenfels and Lewis, 2010; Bellows et al., 2020, Jacoby et al. 2017). Although assessment of these benefits is not possible from Table 3, total biomass measurements provide a simple indication of fungal and bacterial activity, where too much or too little is known to create imbalances in soil ecosystems. There appear to be nonzero amounts of fungal biomass in every sample except IMO2, although sporangia were observed in this otherwise dormant mixture. The imprecision of the values in the cases of the RIMO4, WIMO4 and municipal compost samples prevents confident discussion. In the case of the forest soil and farm compost samples, it may be said with some confidence that fungal biomass values are within the recommended ranges for soil and compost respectively. Interestingly, all samples submitted exceed recommended ranges for bacterial biomass, resulting in low fungi:bacteria ratios across the board. The farm-produced compost is expected to be bacterially dominant given its high temperature and frequent turning. Low fungi:bacteria ratios in municipal compost and IMOs may additionally be explained by the short culturing duration of 1 week as well as their lack of woody material (a preferred food of fungi).
Actinobacteria deserve distinct attention due to special benefits some of them provide, including nitrogen-fixation and production of pest- and disease-deterring antibiotics (Zhang et al. 2019, Miao and Davies 2010). Though at least some actinobacterial activity was observed in all samples, the precision is adequate only in the case of the farm compost, which contains a large amount of actinobacteria, in agreement with its high amount of bacteria in general.
Protozoa are distinguished by their size and motility mechanism, from smallest to largest: flagellates, amoebae, and ciliates. They regulate bacterial populations and at certain ratios may stimulate bacterial growth via ‘grazing.’ Protozoa also release excess nitrogen from consuming bacteria in the form of plant-available ammonium (Ekelund and Ronn 1993). Appreciable protozoa were observed in the forest soil, municipal compost, and farm compost, only the last of which was a precise measurement. The single amoeba observed in WIMO4, and the single ciliate in the forest soil, suggest negligible protozoan activity. The size and diversity of protozoa populations in the farm compost sample provide important benefits given its high bacterial biomass. On the other hand, the absence of protozoa in IMO preparations may prolong bacterial dominance.
Nematodes may be bacterivorous, fungivorous, predatory (to protozoa and other nematodes), omnivorous, or root-feeding . With the exception of the root-feeding types which make up a minority of documented species, nematodes are highly beneficial, providing decomposition and nutrient (i.e. nitrogen) mineralization (Ingham n/d). Some bacterivorous nematodes were observed in WIMO4, municipal compost, and farm compost. Such organisms will provide bacteria population control and nitrogen mineralization in these bacteria-dominated systems. The farm compost also contained fungivorous nematodes which provide similar benefits. The absence of root-feeding nematodes in all samples bodes well for plant vitality. In the case of RIMO4, the absence of nematodes may contribute to bacterial dominance.
Oomycetes are fungi-like eukaryotes, many of which are plant pathogens such as seedling blights, damping-off, root rots, foliar blights and downy mildews (Fawke et al. 2015, Fry and Gruenwald 2010). Oomycete detection was appreciable but imprecise in the case of IMO2, WIMO4, and farm compost samples, and significant and precise in the case of RIMO4. Interestingly, municipal compost did not show oomycete activity. This is the only material to which water was not added. This suggests that controlling for >50% moisture content in IMO and farm-produced compost may have been excessive. The presence of oomycetes is not itself an indicator that the material will negatively impact crops, though the success of pathogens, if they are present, will be more probable if the amendment does not also support a diverse food web. The significant presence of oomycetes in the RIMO4 sample aligns with other results which indicate the anaerobic state of RIMO4 such as the sample’s odor, bacterial dominance, and lack of predators. All of these effects may be the result of the erroneously excessive moisture used in the RIMO preparation. Additionally, that each sample had low fungal biomass suggests that the IMO’s characteristic moldiness may possibly be attributable to oomycetes. Figure 4 shows organisms identified as oomycetes in RIMO4 and WIMO4 samples. In summary, all the methods studied produce bacteria-dominant amendments. IMO preparations contain few fungi, and few bacterial predators, as well as significant amounts of potential pathogens. Forest soil conditions were not re-established within the IMO preparations. The farm compost contained the most prolific and balanced microbiome, whereas the municipal compost was lacking in fungi and bacterial predators.
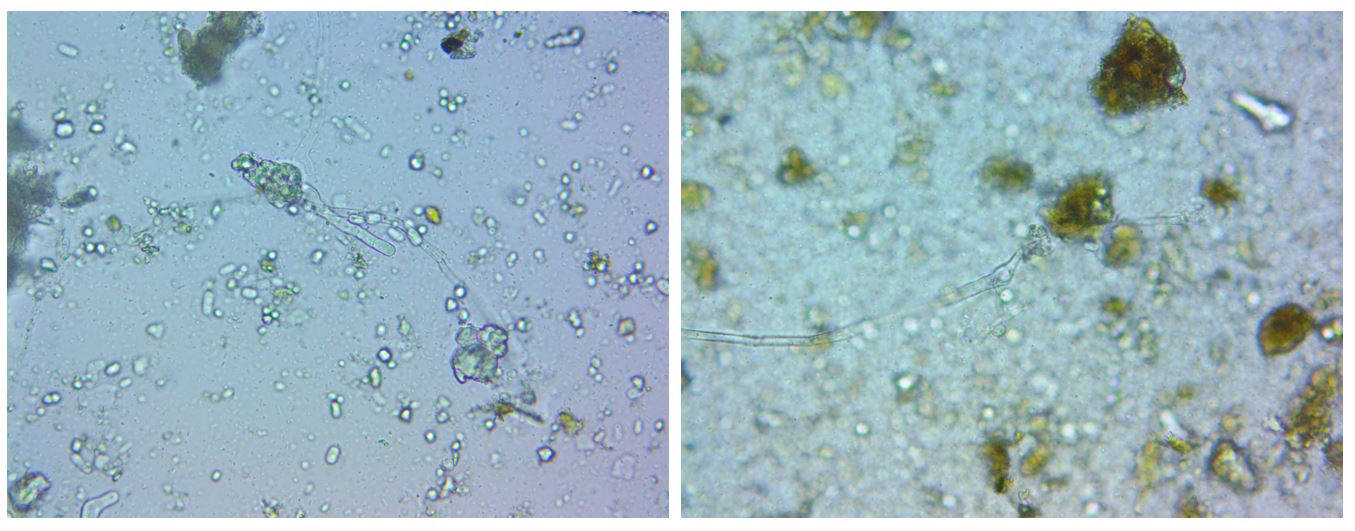
Upon performing the microbiome assessment of MycoGrow the only relevant materials discovered were fungal spores. That no other activity was observed is expected since the product is both dry and shelf stable. Fungi Perfecti’s claim of 1.2 billion propagules of ectomycorrhizal fungi per pound of MycoGrow was verified through observation of 6-13 um diameter spores. However, their claim of 92,000 endomycorrhizal spores per pound, which would be expected to have much larger diameter, could not be verified. Again, the comparison of soil amendment methods is complicated by the distinct application method of MycoGrow (i.e., inoculation of propagules rather than direct addition of organisms). However, noting the lack of fungi present in other amendments, targeted application of MycoGrow may still provide useful benefits given its low-labor accessibility.
Fungi and Bacteria Identification
Tables 4 and 5 show organisms identified in each sample. Information on the organisms’ roles in soil ecosystems is provided. The relative abundance of taxa cultured and identified under laboratory conditions may not resemble that of the field (Davis et al. 2005), as most soil microbes elude efforts to isolate them in the lab As such, it is difficult to gauge the prominence of the taxa presented in Tables 4 and 5 at their sampling site, and these tables most likely underestimate the full microbial diversity in these samples that may have been revealed with other methods like DNA sequencing. Nonetheless, the listed microbes were certainly present in their respective sample at some level, and the distribution of percentages yields at least basic information about each organism’s relative abundance.
Penicillium was identified in the forest soil sample as well as both IMO4 preparations. Yeast, while identified in forest soil, IMO2, and WIMO4, was not identified in RIMO4. No other fungi genera from the forest soil were identified in later stages of the IMO. In the limited scope of this analysis described above this test appears to show higher diversity and more even distribution of fungal genera in the forest soil than in the IMO preparations. This result would be expected due to the distinct food sources and media in the IMO production (i.e. rice, brown sugar, and bran) compared to the forest soil, as well as the short (1 week) culturing duration. This result confirms the hypothesis suggested by the microbiome assessments that IMO poorly reproduces wilderness conditions. The dominance of yeast in lab cultures from IMO2 agrees with the little or no fungal biomass observed in the microbiome assessment. The presence of Fusarium and Penicillium fungi as well as the bacterium Proteus mirabillis in both IMO4 preparations (see Table 5) aligns with their single IMO2 origin batch, as well as their close physical proximity during culturing. Yet, although the farm-produced compost cured somewhat close to the IMO production area, organisms identified in the compost were distinct. This can be explained by the distinct media and conditions of compost versus IMO. In this test’s context, farm-produced compost has higher apparent fungal diversity than the municipal compost. MycoGrow was not submitted for identification. A list of advertised species is included in the Methodology section. Mycorrhizae would not be expected to survive the experiment since no root mass is provided during the culturing process.
Table 5 shows facultative anaerobes in the IMO4’s succeeding an obligate aerobe in the forest soil. This yet again confirms the poor propagation of wilderness organisms by IMO. Bacteria identified in IMO2 appear neither in the forest soil nor the IMO4’s. This result is explained by the low bacterial biomass and relatively dormant state of IMO2. The appearance of acid-tolerant Leuconostoc mesenteroides in IMO2 agrees with its pH of 5.9. Klebsiella pneumoniae in RIMO4 is an interesting discovery, since this organism has been used as an inoculant to boost nitrogen availability (Riggs et al. 2011). Observation of facultative anaerobes in the IMO4 preparations is consistent with their observed anaerobic odor. Identification of two facultative anaerobes in the farm-produced compost is consistent with high moisture curing conditions, though the additional identification of the plant-growth promoting obligate aerobe Pseudomonas fluorescens indicates a range of oxygen availability.
Sample
|
Fungus Identified | Colony Percentage | Information and reference |
Forest soil | Penicillium sp. | 50% | Saprophytic. Globally distributed. Found in soil, decaying organics, seeds and grains (Pitt 1994). |
Cladosporium sp. | 17% | Mainly saprophytic. Found in soil, water, and air. Typically green or black mold (Ogorek 2012). | |
Yeast | 17% | Wide variety of ecosystem functions and habitats. | |
Mortierella sp. | 8% | Saprophytic. Plant growth-promoting. Some extremophiles. Improve access to P and Fe. Protect plants from pathogens (Ozimek and Hanaka 2020) | |
Trichoderma sp. | 8% | Endophytic (Bae et al. 2011). Common component of commercial inoculants. Plant symbionts (Harman et al. 2004). | |
IMO2 | Yeast | 80% | See above. |
Scedosporium sp. | 20% | Genus contains two species. Only clinical studies are available (Castellani and Chalmers 2019). | |
RIMO4 | Penicillium sp. | 78% | See above. |
Fusarium sp. | 11% | Saprophytic or parasitic. Some disperse aerially. Some plant pathogens (Nelson et al. 1994). | |
Rhizopus sp. | 11% | Saprophytic. Only eight species. Assist in fighting pathogenic bacteria and fungi and making nutrients bioavailable (Endrawati and Kusumaningtyas 2017). | |
WIMO4 | Yeast | 56% | See above. |
Penicillium sp. | 28% | See above. | |
Fusarium sp. | 11% | See above. | |
Mucor sp. | 6% | Often the first saprophytic colonizers. Digest a wide variety of carbon food sources (Botha and du Preez 1999). | |
Mun. compost | Cladosporium sp. | 68% | See above. |
Mucor sp. | 32% | See above. | |
Farm compost | Yeast | 36% | See above. |
Aspergillus sp. | 32% | Saprophytic. Found in soil, decaying matter, seeds and grains (Pitt 1994). Many aerobic species. Some grow in nutrient-deprived places (Raper and Fennel 1965). | |
Mucor sp. | 14% | See above. | |
Rhodotorula sp. | 14% | A genus of yeast. Found in the human biome, soil and marine water (Galan-Sanchez et al. 1999). | |
Fusarium sp. | 5% | See above. |
Sample
|
Bacterium Identified | Colony Percentage | Information and reference |
Forest soil | Delftia acidovorans | 95% | Strictly aerobic, nonfermentative and chemo-organotrophic. Found in soil, sediment, activated sludge, crude oil, oil brine, water and humans (Wen et al. 1999). |
Gram negative rod | 5% | N/A | |
IMO2 | Serratia liquefaciens | 63% | When colonizing surfaces, cultures ‘swarm.’ That is, they form biofilms with motility-differentiated subcultures. Resistant to antibiotics. Commonly infects insects and cold-blooded vertebrates (Eberl et al. 1999). |
Rahnella aquatilis | 33% | Nitrogen fixer, utilizes various carbon food sources, and solubilizes mineral phosphates (Kim et al. 2006). | |
Leuconostoc mesenteroides | 4% | Lactic acid bacterium, indicative of early stages of fermentation, (McDonald et al. 1990). | |
RIMO4 | Gram negative rod | 51% | N/A |
Klebsiella pneumoniae | 47% | Facultatively anaerobic (Ochuba and von Riesen 1980). Endophytic diazotroph (Fouts et al. 2008). Inoculants have boosted maize yield (Riggs et al. 2001). | |
Proteus mirabilis | 2% | Facultatively anaerobic (Stewart 1988). Capable of swarming (Rather 2005). | |
WIMO4 | Gram negative rod | 98% | N/A |
Proteus mirabilis | 2% | See above. | |
Mun. compost | Arthrobacter globiformis | 40% | Pleomorphic. Commonly found in soil (Germina and Casida 1980). |
Gram positive rod | 33% | N/A | |
Pseudomonas mendocina | 27% | Pollutant remediator (Kao et al. 2005), increases iron availability (Kohler et al. 2006). | |
Farm compost | Enterobacter sp. | 49% | Facultatively anaerobic. Closely related to the Klebsiella genus. Predominantly discovered in human and other animal intestines, though is also found in plants (Rogers 2020). |
Raoultella ornithinolytica | 39% | Facultatively anaerobic. Has been discovered in soil and water (Drancourt et al. 2001). | |
Pseudomonas fluorescens | 13% | Obligate aerobic. Plant growth-promoting rhizobacterium (David et al. 2018). |
Costs, labor, and key properties of soil amendment methods
Table 6 shows the calculated costs, labor, and results converted from Tables 2 and 3 (nutrients and microbiomes) using the bulk density of each soil amendment. As described in the Methodology, the pore space for compost and IMO materials was calculated to be 78%. At this density it was determined that 1.4 batches of the size produced in this study would cover 100 square feet at 1 inch depth. Thus, a scaling factor of 1.4 was applied to values in Table 6. Values in Table 6 are not scalable to large application areas since costs and labor would not increase linearly.
The high monetary cost and somewhat low labor of IMO are concurrent with lower nutrient levels and beneficial biological activity than composts. As stated previously, nutrient quality of IMO could be improved by mixing with nutrient-rich soil at the IMO4 stage. Whereas nutrient analysis indicated lower amounts in municipal compost than farm-produced compost, the difference in these materials’ densities diminishes their gap in nutrient quality in Table 6. Importantly, if compost were applied based on nutrient needs of the farm rather than a set volume, nutrient effectiveness of farm-produced compost would be improved. The cost of the 50 gallons of wood chips used in the farm-produced compost is higher than the total amount of municipal compost in 100 square feet at 1 inch depth. Cheaper sources of carbon-rich material would further incentivize on-farm composting. Farm-produced compost also requires the most labor by far, though less labor-intensive (i.e. slower) methods of composting could be used to make compost of similar quality. While MycoGrow has the lowest cost and labor, nutrients are negligible compared to IMO or compost, and the subsequent successful growth of fungi will depend on site conditions.
|
RIMO4 | WIMO4 | Municipal compost | Farm-produced compost | MycoGrow* |
Cost ($/100ft2) | $122.12 | $46.02 | $4.94 | $9.78 | $3.63 |
Labor (hrs/100ft2) | 5.5 | 5.5 | 0 | 41.5 | 0 |
Total mass (lb/100ft2) | 169.7 | 163 | 97.2 | 65.8 | 0.025 |
Organic matter (lb/100ft2) | 22.9 | 16 | 40.3 | 51.7 | 0.0129 |
Carbon (lb/100ft2) | 12.7 | 10.3 | 24.3 | 17.1 | 0.00769 |
Nitrogen (lb/100ft2) | 0.7 | 0.7 | 1.3 | 1.3 | 0.00021 |
C:N ratio | 17.4 | 14.6 | 18.7 | 12.8 | 37.2 |
Phosphorus (lb/100ft2) | 0.7 | 0.4 | 0.2 | 0.2 | 1.4E-05 |
Potassium (lb/100ft2) | 0.7 | 0.5 | 0.5 | 1 | 0.00132 |
Fungal biomass (lb/100ft2) | >0 | >0 | >0 | >0 | 0** |
Bacterial biomass (lb/100ft2) | 2.9 ± 0.4 | 3.5 ± 0.7 | 0.9 ± 0.3 | 0.3 ± 0.1 | 0 |
Oomycete biomass (lb/100ft2) | 3.8 ± 0.7 | >0 | >0 | >0 | 0 |
Protozoa count (#/100ft2) | 0 | 0 | 0 | 3.87E7 ± 1.91E7 | 0 |
Nematode count (#/100ft2) | 0 | 5.22E+04 | 1.55E+04 | 1.34E+05 | 0 |
*utilizes manufacturer’s recommended application rate of 1lb/4000 ft2 **fungal growth upon inoculation is beyond the scope of this study |
References
AASL. “Compost Sample Report.” Pennsylvania State University, 2020, https://agsci.psu.edu/aasl/compost-testing.
American Public Health Association. Standard Methods for the Examination of Water and Wastewater. 18th ed., 1992.
Bae, Hanhong, et al. “Endophytic Trichoderm Isolates from Tropical Environments Delay Disease Onset and Induce Resistance Against Phytophthora Capsici in Hot Pepper Using Multiple Mechanisms.” Molecular Plant-Microbe Interactions®, vol. 24, no. 3, Mar. 2011, pp. 336–51. DOI.org (Crossref), https://doi.org/10.1094/MPMI-09-10-0221.
Bellows, Barbara, et al. Microbial Inoculants. ATTRA Sustainable Agriculture, Sept. 2020. Zotero, https://attra.ncat.org/publication/microbial-inoculants/.
Benami, Maya, et al. “The Economic Potential of Arbuscular Mycorrhizal Fungi in Agriculture.” Grand Challenges in Fungal Biotechnology, edited by Helena Nevalainen, Springer International Publishing, 2020, pp. 239–79. DOI.org (Crossref), https://doi.org/10.1007/978-3-030-29541-7_9.
Botha, A., and J. C. du Preez. “Mucor.” Encyclopedia of Food Microbiology, 1999.
Bremner, J. M. “Nitrogen-Total.” Methods of Soil Analysis, Part 3. Chemical Methods., American Society of Agronomy, 1996.
Castellani, Saccardo, and Chalmers. Scedosporium Species. 2019, https://drfungus.org/knowledge-base/scedosporium-species/.
Cornell Waste Management Institute. “Compost Chemistry, Monitoring Compost PH.” Cornell Composting, 1996, http://compost.css.cornell.edu/chemistry.html#:~:text=To%20provide%20optimal%20amounts%20of,each%20part%20nitrogen%20by%20weight, http://compost.css.cornell.edu/monitor/monitorph.html.
David, Baliah V., et al. “Pseudomonas Fluorescens : A Plant-Growth-Promoting Rhizobacterium (PGPR) With Potential Role in Biocontrol of Pests of Crops.” Crop Improvement Through Microbial Biotechnology, Elsevier, 2018, pp. 221–43. DOI.org (Crossref), https://doi.org/10.1016/B978-0-444-63987-5.00010-4.
Davis, Kathryn E. R., et al. “Effects of Growth Medium, Inoculum Size, and Incubation Time on Culturability and Isolation of Soil Bacteria.” Applied and Environmental Microbiology, vol. 71, no. 2, Feb. 2005, pp. 826–34. DOI.org (Crossref), https://doi.org/10.1128/AEM.71.2.826-834.2005.
Drancourt, M., et al. “Phylogenetic Analyses of Klebsiella Species Delineate Klebsiella and Raoultella Gen. Nov., with Description of Raoultella Ornithinolytica Comb. Nov., Raoultella Terrigena Comb. Nov. and Raoultella Planticola Comb. Nov.” International Journal of Systematic and Evolutionary Microbiology, vol. 51, no. 3, May 2001, pp. 925–32. DOI.org (Crossref), https://doi.org/10.1099/00207713-51-3-925.
Eberl, Leo, et al. “Surface Motility of Serratia Liquefaciens MG1.” Journal of Bacteriology, vol. 181, no. 6, Mar. 1999, pp. 1703–12. DOI.org (Crossref), https://doi.org/10.1128/JB.181.6.1703-1712.1999.
Eckert, Donald, and J. Thomas Sims. “Recommended Soil PH and Lime Requirement Tests.” Recommended Soil Testing Procedures for the Northeastern United States, 3rd ed., University of Delaware, 2011, pp. 19–25.
Ekelund, Flemming, and Regin Ronn. “Notes on Protozoa in Agricultural Soil with Emphasis on Heterotrophic Flagellates and Naked Amoebae and Their Ecology.” FEMS Microbiology Reviews, vol. 15, no. 4, Dec. 1994, pp. 321–53. DOI.org (Crossref), https://doi.org/10.1111/j.1574-6976.1994.tb00144.x.
Eliades, Angelo. “How to Make Compost in 18 Days Using the Berkeley Hot Composting Method.” Deep Green Permaculture, 8 May 2010, https://deepgreenpermaculture.com/2010/05/08/hot-compost-composting-in-18-days/.
Endrawati, Dwi, and Eni Kusumaningtyas. “Several Functions of Rhizopus Sp on Increasing Nutritional Value of Feed Ingredient.” Indonesian Bulletin of Animal and Veterinary Sciences, vol. 27, no. 2, May 2017.
Englander, Aaron. Farm-Grown Microbial Soil Inoculants: Effects on Bread Wheat Yield and Quality. GNE11-016, SARE, 2013, https://projects.sare.org/sare_project/gne11-016/.
Fawke, Stuart, et al. “Oomycete Interactions with Plants: Infection Strategies and Resistance Principles.” Microbiology and Molecular Biology Reviews, vol. 79, no. 3, Sept. 2015, pp. 263–80. DOI.org (Crossref), https://doi.org/10.1128/MMBR.00010-15.
Fortune Business Insights. Agricultural Inoculants Market to Reach $1,939.9 Million by 2027. Fortune Business Insights, 8 Feb. 2021, https://www.fortunebusinessinsights.com/press-release/global-agricultural-inoculants-market-10510.
Fouts, Derrick E., et al. “Complete Genome Sequence of the N2-Fixing Broad Host Range Endophyte Klebsiella Pneumoniae 342 and Virulence Predictions Verified in Mice.” PLoS Genetics, edited by David S. Guttman, vol. 4, no. 7, July 2008, p. e1000141. DOI.org (Crossref), https://doi.org/10.1371/journal.pgen.1000141.
Fry, William, and Niklaus Gruenwald. “Introduction to Oomycetes.” The Plant Health Instructor, 2010, https://doi.org/10.1094/PHI-I-2010-1207-01.
Fungi Perfecti. MycoGrow® Soluble. 2022, https://fungi.com/products/mycogrow_soluble.
Galan-Sanchez, Fatima, et al. Microbiological Characteristics and Susceptibility Patterns of Strains of Rhodotorula Isolated from Clinical Samples. p. 4.
Gartley, K. “Recommended Soluble Salts Tests.” Recommended Soil Testing Procedures for the Northeastern United States, 3rd ed., University of Delaware, 2011, pp. 87–94.
Germida, J. J., and L. E. Casida. “Myceloid Growth of Arthrobacter Globiformis and Other Arthrobacter Species.” Journal of Bacteriology, vol. 144, no. 3, Dec. 1980, pp. 1152–58. DOI.org (Crossref), https://doi.org/10.1128/jb.144.3.1152-1158.1980.
Griffin, G., et al. “Recommended Soil Nitrate-N Tests.” Recommended Soil Testing Procedures for the Northeastern United States, 3rd ed., University of Delaware, 2011, pp. 27–38.
Harman, Gary E., et al. “Trichoderma Species — Opportunistic, Avirulent Plant Symbionts.” Nature Reviews Microbiology, vol. 2, no. 1, Jan. 2004, pp. 43–56. DOI.org (Crossref), https://doi.org/10.1038/nrmicro797.
Ingham, Elaine. “Soil Nematodes.” Natural Resources Conservation Service: Soils.
Jacoby, Richard, et al. “The Role of Soil Microorganisms in Plant Mineral Nutrition—Current Knowledge and Future Directions.” Frontiers in Plant Science, vol. 8, Sept. 2017. DOI.org (Crossref), https://doi.org/10.3389/fpls.2017.01617.
Kao, C. M., et al. “Factors Affecting the Biodegradation of PCP by Pseudomonas Mendocina NSYSU.” Journal of Hazardous Materials, vol. 124, no. 1–3, Sept. 2005, pp. 68–73. DOI.org (Crossref), https://doi.org/10.1016/j.jhazmat.2005.03.051.
Keli’ikuli, Ana. Unraveling The Mystery Of The Natural Farming System (Korean): Isolation Of Bacteria And Determining The Effects On Growth. University of Hawai’i, July 2018.
Kim, Kil Yong, et al. “Rahnella Aquatilis, a Bacterium Isolated from Soybean Rhizosphere, Can Solubilize Hydroxyapatite1.” FEMS Microbiology Letters, vol. 153, no. 2, Jan. 2006, pp. 273–77. DOI.org (Crossref), https://doi.org/10.1111/j.1574-6968.1997.tb12585.x.
Kohler, J., et al. “Contribution of Pseudomonas Mendocina and Glomus Intraradices to Aggregate Stabilization and Promotion of Biological Fertility in Rhizosphere Soil of Lettuce Plants under Field Conditions.” Soil Use and Management, vol. 22, no. 3, 2006, pp. 298–304.
Liao, Jianli, et al. “Natural Farming Improves Soil Quality and Alters Microbial Diversity in a Cabbage Field in Japan.” Sustainability, vol. 11, no. 11, June 2019. DOI.org (Crossref), https://doi.org/10.3390/su11113131.
Llamelo, Nathaniel, et al. Effect of Different Bio-Fertilizers Applied as Supplemental Foliar Spray on the Growth and Yield Ff Corn (Zea Mays L.). no. 4, 2016.
Lowenfels, Jeff, and Wayne Lewis. Teaming With Microbes: A Gardener’s Guide to the Soil Food Web. 2nd ed., Timber Press, 2010.
McDonald, L. C., et al. “Acid Tolerance of Leuconostoc Mesenteroides and Lactobacillus Plantarum.” Applied and Environmental Microbiology, vol. 56, no. 7, July 1990, pp. 2120–24. DOI.org (Crossref), https://doi.org/10.1128/aem.56.7.2120-2124.1990.
Miao, Vivian, and Julian Davies. “Actinobacteria: The Good, the Bad, and the Ugly.” Antonie van Leeuwenhoek, vol. 98, no. 2, Aug. 2010, pp. 143–50. DOI.org (Crossref), https://doi.org/10.1007/s10482-010-9440-6.
Nelson, P. E., et al. “Taxonomy, Biology, and Clinical Aspects of Fusarium Species.” CLIN. MICROBIOL. REV., vol. 7, 1994, p. 26.
Ochuba, G. U., and V. L. von Riesen. “Fermentation of Polysaccharides by Klebsielleae and Other Facultative Bacilli.” Applied and Environmental Microbiology, vol. 39, no. 5, May 1980, pp. 988–92. DOI.org (Crossref), https://doi.org/10.1128/aem.39.5.988-992.1980.
Ogórek, Rafał, et al. Characteristics and Taxonomy of Cladosporium Fungi. p. 7.
O’Hara, Bryan. “Biological Inoculants for Soil Health.” Acres USA, Sept. 2017, https://www.ecofarmingdaily.com/build-soil/biological-inoculants-for-soil-health/.
Ozimek, Ewa, and Agnieszka Hanaka. “Mortierella Species as the Plant Growth-Promoting Fungi Present in the Agricultural Soils.” Agriculture, vol. 11, no. 1, Dec. 2020, p. 7. DOI.org (Crossref), https://doi.org/10.3390/agriculture11010007.
Pitt, J. I. “The Current Role of Aspergillus and Penicillium in Human and Animal Health.” Medical Mycology, vol. 32, no. s1, Jan. 1994, pp. 17–32. DOI.org (Crossref), https://doi.org/10.1080/02681219480000701.
Raper, Kenneth, and Dorothy Fennell. The Genus Aspergillus. Williams & Wilkins Co., 1965, https://www.cabdirect.org/cabdirect/abstract/19661301466.
Rather, Philip N. “Swarmer Cell Differentiation in Proteus Mirabilis.” Environmental Microbiology, vol. 7, no. 8, Aug. 2005, pp. 1065–73. DOI.org (Crossref), https://doi.org/10.1111/j.1462-2920.2005.00806.x.
Reddy, Rohini. Cho’s Global Natural Farming. South Asia Rural Reconstruction Association, 2011.
Riggs, Patrick, et al. “Enhanced Maize Productivity by Inoculation with Diazotrophic Bacteria.” Functional Plant Biology, vol. 28, no. 9, 2001, pp. 829–36.
Roberts, Rebecca. Effect of an Indigenous Soil Microbial Inoculant on Soil, Soil Microbial Community and Leaf Nutrient Density. Progress Report, FNE19-943, SARE, 2021.
Rogers, Kara. “Enterobacter.” Britannica.
Sakimin, Siti Zaharah, et al. “Effects Of Indigenous Microorganism And System Of Rice Intensification Formulation On Growth, Physiology, Nutrient Uptake And Rice Yield.” Bangladesh J. Bot., vol. 46, no. 1, Mar. 2017.
Stewart, V. “Nitrate Respiration in Relation to Facultative Metabolism in Enterobacteria.” MICROBIOL. REV., vol. 52, 1988, p. 43.
Tarbell, T. J., and R. E. Koske. “Evaluation of Commercial Arbuscular Mycorrhizal Inocula in a Sand/Peat Medium.” Mycorrhiza, vol. 18, no. 1, Dec. 2007, pp. 51–56. DOI.org (Crossref), https://doi.org/10.1007/s00572-007-0152-3.
USDA and US Composting Council. Test Methods for the Examination of Composting and Compost. 2002.
Wang, Koon-Hui, et al. Evaluating the Benefits of Korean Natural Farming Practice on Tropical Vegetable Crop Production in Hawaii. 69-9251-11–912, NRCS, May 2012, p. 11.
Wen, Aimin, et al. “Phylogenetic Relationships among Members of the Comamonadaceae, and Description of Delftia Acidovorans (Den Dooren de Jong 1926 and Tarnaoka Etal. 1987) Gen. Nov., Comb. Now.” International Journal of Systematic Bacteriology, p. 10.
Wolf, A. M., and D. B. Beegle. “Recommended Soil Tests for Macronutrients.” Recommended Soil Testing Procedures for the Northeastern United States, 3rd ed., University of Delaware, 2011, pp. 39–47.
Wright, Melody. Comparison of Indigenous Microorganism and Commercial Soil Inoculant on Crop Yields and Basil Downy Mildew Disease Resistance. FNE17-883, SARE, 2019.
Zhang, Binglin, et al. “Variation in Actinobacterial Community Composition and Potential Function in Different Soil Ecosystems Belonging to the Arid Heihe River Basin of Northwest China.” Frontiers in Microbiology, vol. 10, Sept. 2019, p. 2209. DOI.org (Crossref), https://doi.org/10.3389/fmicb.2019.02209.
Zuraihah, I. Illani, et al. “Effects of IMO and EM Application on Soil Nutrients, Microbial Population and Crop Yield.” J. Trop. Agric. and Fd. Sc., vol. 40, no. 2, 2012, pp. 257–63.
The IMO production process shows distinct biology at each stage, and nutrient quality of IMO is strongly affected by the nutrition of the soil used in the IMO4 stage. There are some similarities between nutrient levels and fungal genera at the IMO1 collection site and the final IMO4 product, though overall biological conditions at the collection site are not reproduced by IMO4. Benefits of IMO are anecdotally attributed to bacteria and fungi, but this study showed dominance of bacteria and negligible bacterial predation, presence of oomycetes, and lack of fungi. Future research could evaluate whether distinct conditions and media at each stage in the IMO production interrupt the progression of biological communities. This may assist in modifying conditions within the IMO production to host more beneficial organisms. Furthermore, lowering the requirement for >50% moisture may improve access to oxygen, and nutrient quality of IMO can be improved by the use of nutrient-rich soil at the IMO4 stage. That the use of wheat bran produced IMO of similar quality to rice bran suggests that cost-effectiveness may be further improved by the use of local alternatives for brown sugar and topsoil.
Anaerobic conditions in the IMO production led to a less balanced ecosystem than that of farm-produced compost. IMO also had lower nutrient quality and higher financial cost than both farm-produced and municipal composts. Farm-produced compost was more nutrient dense than municipal compost. However, due to the composts’ difference in mass density, access to nutrient benefits depends on how farmers apply the material. In any case, farm-produced compost hosted the most balanced soil ecosystem of all materials studied, which would provide greater benefit than other materials regardless of differences in mass density. Additionally, cheaper sources of carbon and less labor-intensive composting methods could create compost of similar quality to that produced in this study.
Low fungal biomass in IMO and compost is attributable to the short (<1 month) timescales used. This underscores the utility of fungal inoculant products such as MycoGrow as cheap, low-labor inoculation methods. Although MycoGrow provides negligible nutrients and endomycorrhizal spores were not observed in this study, the use of MycoGrow in combination with short timescale amendments may provide unique economic advantages. Nonetheless, finding an effective application rate for any fungal inoculant necessitates experimentation by the farmer (Benami et al. 2020, Tarbel and Koske 2007), and the success of non-indigenous fungi depends significantly on site conditions (Bellows et al. 2020). Readers interested in methods of producing “indigenous” mycorrhizal fungal inoculants on site are referred to Englander 2013.
Though IMO appears to be a lackluster alternative to compost, insights presented in this study highlight the potential for adaptation of conditions and materials to improve the quality and sustainability of IMO. As investigations continue into cost-effective and locally appropriate methods of supporting soil life, we suggest composting in the meantime.
Education & Outreach Activities and Participation Summary
Participation Summary:
Throughout this project, 17 interns in Unadilla Community Farm’s Beginning Farmer Training Program participated in the on-farm production and preparation of lab samples of hot compost and IMO. Through an on-farm class, the interns were also provided with an in-depth seminar on the motivations, methods, and results of the project. In addition, 1 farm employee and 2 farm managers led the project, conducted the research, and prepared educational materials.
The methodologies of hot composting and IMO production developed through this project, as well as the research results, are being used to inform soil amendment decisions at Unadilla Community Farm.
The educational products of this project, including a comprehensive technical report, published articles, and podcast, have been compiled into a landing page on Unadilla Community Farm’s website for further promoting the results of this research. This landing page can be found at unadillacommunityfarm.org/IMO.
An article was written for Garden Culture Magazine, whose readership is estimated to be 47000 readers per month. The article examines the challenges with IMO encountered in this study and was accepted by the editor on December 6th, 2022 for publication in the magazine’s February edition.
An article was written for the Permaculture Research Institute. This article summarizes the project’s results in less technical language than the comprehensive report, making it more accessible for a general audience. This article was published on December 12th, 2022.
Permaculture Research Institute Article
An interview was completed for the Plant Cunning Podcast, which consistently receives approximately 1000 listeners per episode. The interview summarizes the project’s main findings in the theme of this podcast.
https://www.youtube.com/watch?v=drqe321WSYo
Learning Outcomes
Nutrient management is an essential aspect of agriculture, to which this study adds commentary on the economic and environmental sustainability of three amendment options. Our recommendation to farmers considering IMO, compost, and commercial inoculants is threefold: compost appears to have the best short term advantages in terms of cost, nutrients, and biology; commercial inoculants may not include advertised material and will necessitate experimentation to find an effective application rate; and the recipe we used for IMO resulted in potential pathogens and anaerobic properties, in part due to the excessive moisture content used. Recommendations for future research to adapt the IMO recipe are given below.
When disseminating results, it is important to begin with the economic and environmental motivations for using IMO or other locally produced soil amendments to provide context for this study. It is also useful to discuss new knowledge of soil microorganisms as they relate to plant health. The most common question received throughout our education is: “What are the results that you want?” Our answer is that no results in particular are desired, that this project is a comparison of chemical and biological properties as they are. We have no agenda for or against the use of IMO. In general we are trying to provide a glimpse at potential benefits of IMO and commercial products relative to a well-understood method of composting. Time-conscious farmers will find concise summaries of the project’s main results and the challenges we encountered with IMO in the Permaculture Research Institute and Garden Culture Magazine articles, respectively. Otherwise, ordinary audiences of the Permaculture Research Institute, Garden Culture Magazine, and Plant Cunning Podcast will encounter this project’s results.
Project Outcomes
This study has provided a unique, in-depth analysis to help Northeast farmers make informed nutrient management decisions. As a result of this study, we have decided not to implement IMO on our farm until further studies produce an improved methodology with reduced costs and proven benefits for Northeast farmers. In the meantime, we will continue to explore best practices regarding the use of commercial inoculants as a supplemental microbiology source applied alongside either farm-produced or municipal compost. We are sure that other farmers who read this project’s materials will walk away with valuable knowledge to inform their own decisions.
This project has deepened my personal understanding of soil ecology, soil amendment chemistry, and farm economics, and has brought much of that knowledge to others. Myself and those taught have improved skills such as composting, IMO production, and agricultural research methods. I also feel a stronger workplace confidence and improved communication and management skills.
Keys to success. This project was overall successful. Previous research experience allowed me to estimate and pursue an appropriate scope with the given resources. The keys to success include extensive planning, setting reasonable goals, and having clear expectations for responsibility of project participants. These actions minimized the challenges encountered, though we did encounter several issues.
Challenges and recommendations.
- Most prominently, an erroneously high water content was used in the production of IMO. Rather than following the recipe’s recommendation of adding just enough water to make the bran “clump when pressed and loosen when shaken,” an arbitrary amount (5 gallons) of the diluted IMO2 was added to both the rice bran and wheat bran. This led to an excessive water content that may have accentuated the anaerobic qualities of IMO discussed in the report, especially in the case of the rice bran-IMO which was much moister than the wheat bran-IMO. Though drying the rice bran-IMO was attempted by leaving it uncovered for two days, the moisture content remained excessive throughout the IMO production. This issue and remedy are also explained in the ‘Materials and Methods’ section of the report, since this directly interfered with ideal experimental conditions.
- We were not able to meet our initial objective of anecdotally observing the effects of IMO on plant growth. This is because the brans were not certified organic, and Unadilla Community Farm’s farming practices prohibit the application of such materials on fields used for commercial production. Seeking organic brans may have resolved this issue, though meeting the objective would trade off with the added expenses and difficulty of obtaining these materials.
- The comparison of the commercial mycorrhizal inoculant MycoGrow® Soluble was limited by our experimental design. When designing the experiment it was assumed that nutrient analysis and microbiome assessment of MycoGrow® would provide more useful information. However, we overlooked the fact that the biology of MycoGrow® consists of propagules and that of compost and IMO consist of living organism communities. This oversight could have been avoided by instead choosing a commercial product which contains live organisms or abandoning the commercial product comparison. Studies wishing to compare inoculant products would require plant growth trials, which brings up the uncertainty of effective application rates. These questions are beyond the scope of this project.
- Fourth, the compost exceeded the desired temperature range, which is most likely attributable to an underestimation of the nitrogen content in grass clippings. This could have been resolved by more extensive preliminary research during the planning phase.
- A fifth challenge encountered is the variability of the microbiome assessment (soil food web microscopy) method. This interfered with many accurate comparisons, but still allowed some informative discussion. This is a common occurrence with the Soil Food Web test, but the issue was also unknown to the author when the experiment was being designed. Knowing that some results would carry large variability would have assisted in having adequate expectations of accuracy and thus designing more effective experiments. It is possible we would have pursued additional or separate characterization methods if we had proper expectations.
- Finally, this study’s use of one experimental trial significantly limits its scope and rigor. With multiple trials, the study could have achieved sufficient precision to seek publication in academic journals, which would increase its audience.
Meeting project objectives. Besides the lack of anecdotal plant growth trials and comprehensive comparison of MycoGrow®, this project effectively met all of its other objectives. We provide insight into previously unobserved properties of IMO and compare such properties to compost. We also provide at least some information about MycoGrow® and compare it as best we can.
Promoting the practice. As indicated in the conclusion of the report, this study highlights many challenges with IMO. We do not singlehandedly disprove the benefits of IMO, and indeed this project’s limited scope may be challenged by future studies. However, we do add a dissenting perspective to the growing praise for IMO. Importantly, we attempt not to dissuade others from working with IMO on small scales and recommend further trials to improve IMO’s effectiveness and sustainability in the Northeast. We hope that our study contributes to these goals.
Needs for additional work. Future work can attempt to adapt the IMO recipe to cultivate more beneficial organisms, for example by lowering the water content and using more porous covering while observing outcomes on IMO biology. Additionally, the traditional recipe uses white rice, brown sugar, and rice bran, whereas little scientific work exists on IMO produced using other grains, sugars, and brans that may be more appropriate to certain localities.
Our knowledge’s benefit. Our study is suited to teach anyone who has heard of IMO and its benefits. The largest benefit will be had by Northeast farmers and gardeners who are considering using IMO as an alternative or additional soil amendment. We provide essential commentary on the economics of IMO compared to compost and commercial inoculants, while assessing IMO in terms of its potential effects on plants and soil.
Information Products
- Permaculture Research Institute Article (Article/Newsletter/Blog)
- Plant Cunning Podcast, Ep.101: MattyB on Indigenous Microorganism (IMO) Research (Video)
- Project Summary Website / "Landing Page" (Website)
- Comparison of Farm-produced and Commercial Microorganism Inoculants: Cost, Nutrients, and Biological Constituents (Article/Newsletter/Blog)