Final report for GNC17-248
Project Information
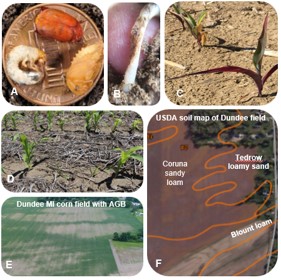
C) As few as a single grub can cause a corn seedling to wilt and discolor. (A. Pekarcik, Ohio State). D) Seedlings fed on by AGB are often severely stunted which can significantly reduce yield (Bruce MacKellar, Michigan State). E) Infestations in field crops are often restricted to E) sandy areas of fields as seen in an early season corn field in Dundee, MI (Ricardo Costa-Silva, Michigan State).
F) USDA soil survey map outlining limits of soil types which overlap with AGB damage from Dundee, MI field.
Over the past decade grubs of the Asiatic garden beetle (Fig. 1A), Maladera castanea Arrow (Coleoptera: Scarabaeidae: Melolonthinae), which will be referred to as AGB for the rest of this report, have emerged as an early season subterranean root feeding pest of corn in Indiana (Krupke et al. 2007), Michigan (DiFonzo 2007), Ohio (Hammond 2013), and to a lesser extent Virginia (Tiwari et al. 2008). AGB was initially introduced to the United States in New Jersey in 1921 and would eventually cause sporadic economic damage to turf and ornamentals (Hallock 1936). AGB has since spread to at least 24 states and 2 Canadian provinces (Eckman 2015). The first report of AGB in Ohio occurred around 1960 (CABI 1961), and by 2012 the grubs were first observed heavily infesting corn (Figs. 1B-E) in sandy soils (Fig. 1F) in the northwestern counties (Hammond 2013). There are currently no successful management tactics or rescue treatments available for management of AGB in corn (Richer and Michel 2014), often leaving farmers to replant (at great expense) should infestations become too severe.
Entomopathogenic (insect-killing) nematodes (EPNs) are naturally occurring in the environment and could be a sustainable control agent for use against AGB in corn (Rasmann et al. 2005; Kergunteuil et al. 2016); commercial nematodes are successful for AGB management in turfgrass (Tashiro 1987; Morales-Rodriguez et al. 2010). Recent advancements in rearing techniques and easy-to-use formulations have lowered production costs which has made it feasible to work with locally isolated nematodes (Testa and Shields 2017) instead of commercially purchased one that are accustomed to laboratory conditions and not environmental extremes. Benefits of local nematodes compared to commercially available nematodes are that they are better adapted to local environmental extremes, can persist longer in the absence of insect hosts (Shields et al. 2009), and are cheaper to mass produce and apply from modified spray equipment (Lacey and Georgis 2012).
The goal of this research was to assess AGB susceptibility to locally isolated EPNs in Ohio field corn and understand the spatial distribution of EPN in agroecosystems. To accomplish this, EPN were isolated from the soil samples collected at farms affected by AGB in northern Ohio during 2018 and 2019 and they were evaluated in the greenhouse for efficacy against AGB grubs. Isolated nematodes were subsequently be mass reared “in vivo” using economical and low input technologies. Local AGB populations will be collected from local farms and evaluated for susceptibility to these nematode species in greenhouse and field trials. Field sampling data were analyzed to identify trends in spatial distribution over time. The results from this research will help develop a sustainable management plant for AGB in corn using EPN.
This proposal includes an outreach component to educate farmers about the safety and persistence of these nematode species, and to provide demonstrations regarding rearing nematodes at home and applying them with modified spray equipment. Findings from this research have been presented at annual AGB meetings held in Wauseon, OH in March 2019 and February 2020, and an extension handout that educates readers on the life cycle and utility of EPN with respect the AGB, how to isolate, extract, mass-rear, and apply EPN for AGB control, and other important pieces of information. Contingency plans have been devised for the research portion of this study, while extension events including talks and workshops will be evaluated pre- and post-workshop surveys.
Additional EPN assessments beyond the grant period will be conducted in 2020 and 2021. EPN isolated from 2018 and 2019 will be evaluated for efficacy against AGB in sand and loam soils placed in microplots in the field at the Ohio Agricultural Research and Development Center’s Snyder Farm in Wooster, OH. Additionally, efforts to DNA barcode isolated EPN species from 2018 and 2019 are ongoing and will be used to confirm identification of the collected species and produce a phylogenetic estimation to assess population structure in northern Ohio farms. These findings will be included in a comprehensive peer-reviewed manuscript to be published in the Journal of Nematology.
_________________________________________________
References Cited
(CABI) CAB International. 1961. Maladera castanea. Distribution maps of plant pests, Map 121. (http://www.cabi.org/dmpp/default.aspx?site=164&page=1060).
DiFonzo, C. 2007. Asiatic garden beetle in southern Michigan. Michigan State University Extension. Accessed March 20, 2017. http://msue.anr.msu.edu/news/asiatic_garden_beetle_in_southern_michigan
Eckman, L. 2015. Host plant feeding preferences of the adult Asiatic garden beetle, Maladera castanea Arrow (Coleoptera: Scarabaeidae). MS thesis, UConn. 1-95.
Hallock, H.C. 1936. Life history and control of the Asiatic garden beetle. USDA Circ. 246: 1-20.
Hammond, R. 2013. Asiatic garden beetle could be cause for concern for northern Ohio corn. About Us: News. OSU, Department of Entomology. Accessed on March 20, 2017. https://cfaes.osu.edu/news/articles/asiatic-garden-beetle-could-be-cause-for-concern-for-northern-ohio-corn
Kanzaki N., K. Futai. 2002. A PCR primer set for determination of phylogenetic relationships of Bursaphelenchus species within the xylophilus group. Nematology. 4(1): 35-41.
Kaya, H.K., T.M. Burlando, G.S. Thurston. 1993. Two EPN Species with Different Search Strategies for Insect Suppression. Environ. Entomol. 22(4): 859-864.
Kergunteuil, A., M. Bakhtiari, L. Formenti, Z. Xiao, E. Defossez, S. Rasmann. 2016. Biological control beneath the feet: A review of crop protection against insect root herbivores. Insects. 7(4): DOI:10.3390/insects7040070
Krupke, C., J. Obermeyer, L. Bledsoe. 2007. A new field crops pest for Indiana: Asiatic garden beetle. Purdue Cooperative Extension Service. Accessed March 20, 2017. https://extension.entm.purdue.edu/pestcrop/2007/issue11/
Lacey, L.A., R. Georgis. 2012. EPN for control of insect pests above and below ground with comments on commercial production. J. Nematol. 44(2): 218-225.
Morales-Rodriguez, A., A. Ospina, D.C. Peck. 2010. Variation in the laboratory susceptibility of turf infesting white grubs (Coleoptera: Scarabaeidae) to biological, biorational and chemical control products. Pest Manage. Sci., 66: 90-99.
Powers, T.O., E.C. Bernard, T. Harris, R. Higgins, M. Olson, M. Lodema, P. Mullin, L. Sutton, K.S. Powers. 2014. COI haplotype groups in Mesocriconema (Nematoda: Criconematidae) and their morphospecies associations. Zootaxa. 3827(2): 101-146. http://dx.doi.org/10.11646/zootaxa.3827.2.1.
Rasmann, S., T.G. Kollnwe, J. Degenhardt, I. Hiltpold, S. Toepfer, U. Kuhlmann, J. Gershenzon, T.C.J. Turlings. 2005. Recruitment of entompathogenic nematodes by insect-damaged maize roots. Nature. 434: 732-737.
Richer, E.A., A. Michel. 2014. Product efficacy on Asiatic garden beetles in field corn. OSU Extension. Accessed March 20, 2017. https://agcrops.osu.edu/sites/agcrops/files/ofr_reports/2014%2520Fulton%2520AGBproducts.pdf
Shields, E.J., A. Testa, G. Neumann, K.L. Flanders, P.C. Schroeder. 2009. Biological control of Alfalfa snout beetle with a multi-species application of locally adapted persistent EPN: The first success. Amer. Entomol. 55(4): 250-257.
Tashiro, H. 1987. Turfgrass insects of the United States and Canada. Cornell University Press, Ithaca, NY. 156-192.
Testa, A.M., E.J. Shields. 2017. Low labor “in vivo” mass rearing method for EPN. Biol. Contr. 106: 77-82.
Tiwari, S., C.A. Laub, R.R. Youngman. 2008. Asiatic garden beetle in field corn. Virginia Cooperative Extension. Accessed March 20, 2017. https://pubs.ext.vt.edu/444/444-108/444-108_pdf.pdf
The learning outcomes stemming from this project are that farmers will be able to:
1) understand how EPN provide a sustainable, low input, and
economical management tool with minimal off-target effects
2) learn which EPN AGB is susceptible to
3) identify nematodes based on cadaver color and texture
4) learn how to rear and applying EPN at home for personal use
The action outcomes from this study are that farmers interested in utilizing EPN for management of AGB in corn will be able to:
1) rear their own EPN for application on their personal farms
2) use local EPN for management of AGB in corn
3) minimize insecticide use for management of AGB in corn.
Cooperators
- (Educator)
- (Educator)
- (Educator)
- (Educator)
Research
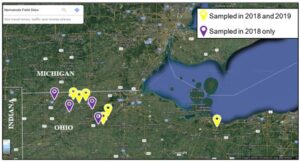
EPN were first isolated from soil samples collected at farms affected by AGB in northern Ohio. Isolated nematodes were subsequently mass reared “in vivo” using economical and low input technologies. Local AGB populations were collected from local farms and evaluated for susceptibility to these nematode species in preliminary greenhouse trials. AGB grubs were sampled in the same plots that nematodes were sampled from; investigations into the relationship between the presence of nematodes and AGB grubs were conducted. Field trials evaluating EPN efficacy against AGB grubs will occur in 2020 in a microplot study to be conducted at Wooster, OH that will monitor the efficacy against AGB and persistence of isolated nematode species over the course of 2 years. The results from this research will help develop a sustainable management plant for AGB in corn using EPN.
Objective 1. Screen local soils for presence of naturally occurring EPNs
Experimental Design
I worked closely with Ohio State University Extension Educators Eric Richer (Fulton and Lucas Counties), John Schoenhals (Williams Co.), Garth Ruff (Henry Co.) and Mike Gastier (Erie Co.) in April of 2018 to meet, discuss plans with, and obtain permission from 9 local farmers across 5 Ohio Counties to sample for nematodes and AGB on their land for a total of 10 corn-soybean rotated farms (5 fields from Fulton County, 2 from Henry, and 1 from Erie, Lucas, and Williams) (Fig. 2). Several of these farmers are fervent supporters of on-field extension research conducted previously by Ohio State University research staff. In addition, land at the OSU - Ohio Agricultural Research and Development Center in Wayne County, Ohio will be used for a field trial to evaluate nematode persistence and efficacy throughout 2021 and 2021. Although we did not reach our goal of 40 fields due to time constraints, we decided to continue resampling 6 of the 10 fields sampled in October 2018 with high AGB infestations during June and October 2019. In 2018, bait stations or soil cores (3 cm diameter x 20 cm long) were placed or taken, respectively, within 20 plots arranged in a 5×4 grid across a 4-5-acre area (Fig. 3); these plots were already being used for an AGB spatio-temporal sampling network and will further compliment the research being conducted in that study.
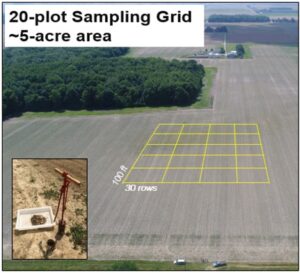
EPNs were initially sampled using bait stations and soil cores. First, bait stations were set out during the week of September 23, 2018 to attract and isolate EPN in the field. Each bait station consisted of a wire mesh tube-shaped enclosure (2.54 cm diameter x 7.64 cm long) filled with 10 waxworms and closed with a condiment cup lid then buried under soil near the surface and left for one week. After one-week bait stations were collected and brought back to the lab. Discolored, dead waxworms (e.g. infected by nematodes or other microbials) were then placed on a White trap (see Objective 2 for description) and surrounded by distilled water to promote emergence of nematodes from the waxworm cadavers into water. White traps were maintained at room temperature (~23OC) in the dark (under cardboard on a counter) for as long as it took for nematodes to emerge (~3-7 days). Emerged nematodes were identified by a yellowish and cloudy appearance of the water, and confirmation under a dissecting microscope.
Unfortunately, a combination of raccoons, ants, and parasitic fly species decimated the waxworms from a significant number of the bait stations from several locations and we could not conduct statistical analyses on them, so for the remainder of this study we isolated nematodes from soil cores. A total of 60 soil cores (2.54 cm diameter x 20 cm long) were randomly sampled from each field (3 per plot) with each core being split among two containers based on soil depth as different nematode species are known to persist at different depths; Steinernema spp. generally will sit and wait for an insect host near the soil surface to move past them, while Heterorhabditis spp. actively search for insect hosts lower in the soil profile (Kaya et al. 1993). For this study the top 5 cm and bottom 15 cm of each soil core were placed into labeled 12-oz and 32-oz deli containers, respectively. Containers were then brought to the lab and 5 or 10 greater wax moth, Galleria mellonella (L.), larvae were added to the small and large deli containers, respectively, as they are highly susceptible to EPN infections (Testa and Shields 2017). Containers were ventilated by poking holes through the lid. Each container was flipped upside down to encourage contact between nematodes and waxworms. After 72 hours, G. mellonella mortality rates and cadaver discoloration were recorded for each core subsample along with the number of samples resulting in a positive infection.
Statistical analyses. The number of soil samples containing each entomopathogen were compared from the upper 5 cm and lower 15 cm of soils cores over time using a two-way ANOVA with PROC GLM (SAS 9.4), and the number of positive samples with any entomopathogen were compared among evaluation dates for the upper 5 cm and lower 15 cm using two-way ANOVA with PROC GLM (SAS 9.4). The changes in entomopathogen populations over time was assessed with separate paired t-tests for each entomopathogen using PROC TTEST (SAS 9.4). Furthermore, multiple linear regression analysis was used to assess how evaluation date, site, crop, percent sand, percent silt, percent clay, and presence of each isolated entomopathogen using PROC REG (SAS 9.4); initial analysis indicated that silt, clay, and Ma should be removed as they behaved colinearly with other variables. Robust regression (PROC ROBUSTREG, SAS 9.4) was also used, but did not strengthen the analysis. Principal component analysis was used to assess the relatedness of sampling sites based on a combination of site components including entomopathogen species, sand, silt and clay content, evaluation period and crop were using PROC PRINCOMP (SAS 9.4).
Objective 2. Rear isolated EPN species using established “in-vivo” techniques
For each location, isolated nematode species were pooled by cadaver color and reared separately following Testa and Shields’ (2017) protocol. This process is detailed in picture in an EPN handout that was produced for this research. Emerging nematodes were isolated from G. mellonella cadavers using White traps which consisted of a small plaster of Paris disk (~5 cm) placed inside a larger petri dish which is then topped with a filter paper and ten infected cadavers per bait station and soil core sample. Distilled water was added to each petri dish to surround the cadavers and facilitate natural emergence of nematodes away from the waxworm host. The emerged nematodes, cadavers, and traps were rinsed with approximately 25 ml distilled water into a beaker. Standard serial dilution was used to attain a concentration of approximately 15,000-30,000 nematodes per 25 ml. The 25 ml of nematodes was added to plastic containers (~450 ml) filled ¾ full of sawdust and 250 G. mellonella larvae, closed with a vented lid, and monitored daily for nematode emergence. Each container was then rinsed with distilled water through two stacked mesh sieves (Nos. 40, 20) for 2-3 minutes. The flow through was collected and standardized to 15,000-30,000 nematodes per 25 ml. Autoclaved soil was inoculated with 25 ml of nematode stock and stored in the dark at room temperature for long-term reservoirs up to 3 months, although several attempts were unsuccessful.
EPN species were also obtained from Dr. Elson Shields at Cornell University and added to the stocks of our nematode species to increase genetic diversity. All nematode species were augmented in isolated areas on the OARDC campus to maintain long-term natural populations that could be used at any time.
Objective 3. Evaluate AGB susceptibility to these nematodes in corn
V2 organic corn plants were grown in the greenhouse at a temperature of 20oC ± 3. Individual corn seeds were sown into 32 oz deli containers; this size container is nearly identical to the volume of soil we use to sample for AGB in the field. Soil was either Millgrove loam or Haskins fine sandy loam, both collected from the same field in Henry County, OH to identify whether soil type influenced nematode infection rates. AGB 2nd and 3rd instar grubs were collected from an infested field in Henry County, OH on October 8 and stored in a refrigerator in soil at 10oC prior to experimentation. Prior to efficacy tests, AGB grubs were placed at room temperature for 24 hours, after which 2 of either 2nd or 3rd instar grubs were added to each replicate and allowed to acclimate for 24 hours. On October 11, 2018, one of the five treatments (water control, Hb, Hm, Sc, and Hb + Hm) was then applied to the appropriate containers at a conservative rate of approximately 1000 IJs per 25 ml water for a total of 12 plant replicates per treatment. This rate was chosen as it is often used to evaluated EPN infection potential against insects in the lab. After 7 days, grubs were evaluated for EPN induced mortality, and plant shoots and roots were separated, dried, and weighed. Grub mortality, root, shoot, and total plant weights were compared for EPN treatments in separate one-way ANOVAs with Lsmeans ≤ 0.05 and Tukey-Kramer post-hoc tests.
Objective 1. Screen local soils for presence of naturally occurring EPNs
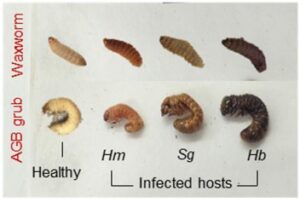
Identification of isolated EPNs. Based on the resulting waxworm and AGB grub cadaver colors (Fig. 4) and confirmation of the presence of EPNs under the microscope with a basic assessment of relative size and body plan, three species, in addition a fungus, Metarhizium anisopliae (image not shown), were successfully isolated from every field sampled. The nematode species include (Fig. 4): Heterorhabditis bacteriophora (Hb), H. megidis (Hm), and Steinernema glaseri (Sg). M. anisolpliae (Ma) infections are observed when the insect host turns a greenish-gray color and white structures form on top of it that secrete droplets. Efforts are currently being made to confirm the identification of these species using the following protocol that was developed by Camila Hofman et al. (in press) to sequence the mitochondrial cytochrome oxidase subunit I (COI) gene.
Nematode Identification: mtCOI DNA barcoding. Single nematodes for each cadaver color were placed in 1.5 ml microcentrifuge tubes, then onto glass coverslips in 18 μl of sterile water. DNA extractions followed a modified protocol (Powers et al. 2014) where nematodes were sliced into pieces using a razor blade under a dissecting microscope. Mashed nematodes were stored in molecular grade water in individual 0.25 ml PCR reaction tubes until PCR reactions were performed. The primer set used to amplify the mitochondrial cytochrome oxidase subunit I (COI) gene consisted of COI-F1KF (29bp, 5’- CCTACTATGATTGGTGGTTTTGGTAATTG-3’) and COI-R2KF (23bp, 5’- GTAGCAGCAGTAAAATAAGCACG-3’) (Kanzaki and Futai 2002). Used. PCR amplification reactions will consist of 6.4 ml of ddH2O, 1.8 ml of each 20 mM primer, 15 ml of 2XJumpStart RED Taq ReadyMix (Sigma-Aldrich, Inc. St. Louis, MO) RED Taq ReadyMix (Sigma-Aldrich, Inc. St. Louis, MO) and 5 ml DNA template from the macerated nematode, for a total reaction volume of 30 ml. PCR cycling protocol will consist of a hot-start and an initial denaturation of 5 minutes at 94°C followed by 45 cycles of 15 seconds at 94°C (denaturation), 15 seconds at 55°C (annealing), 60 seconds at 72°C (extension) and a final extension step of 5 minutes at 72°C. All PCR will be conducted in a thermal cycler at the Ohio State University Department of Entomology. To confirm successful amplifications, 3 ml of PCR products will be loaded into 1% agarose gels stained with GelRed Nucleic Acid Gel Stain (Biotium, Hayward, CA, USA) in 1× Tris-acetate-EDTA (TAE) buffer. Gels will be placed into electrophoresis with 0.5X Tris-Borate-EDTA (TBE) running buffer for 35 minutes at 155V. DNA templates will be sequenced via bidirection Sanger sequencing at Functional Biosciences (Madison, WI), then edited and aligned on Geneious (Biomatters Inc., Newark, NJ). Excluding the primers, amplification products should yield 658-bp for resulting sequences.
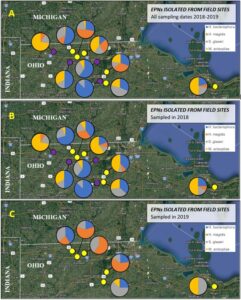
Initial attempts at Sanger sequencing rendered a majority of bases as ambiguous and ultimately useless. Attempts will be made to clean up the PCR products and resequence them at the Ohio State University Molecular and Cellular Imaging Center in Wooster, OH. If that does not work, then individual nematodes will be macerated following the Powers et al. (2014) protocol verbatim and re-sequenced at the MCIC. Resulting sequences will be uploaded into Geneious Prime software and the consensus sequences formed, then be submitted to NCBI GenBank following publication of the resulting manuscript from this research. The sequences will be used to create a maximum likelihood phylogenetic estimation using MEGA X software following models selected by Bayesian Information Criterion scores. These results will increase our understanding of the genetic makeup of naturally occurring nematode populations which could give us insight to how similar they are; should species from nearby fields be genetically distinct, then a more targeted approach might be necessary when isolating local EPNs to mass rear and apply back into the system to ensure compatibility.
EPN spatio-temporal dynamics. The overall species composition of entomopathogens overall (Fig. 5A) and in 2018 (Fig. 5B) and 2019 (Fig. 5C). Three of the four sites sampled only in 2018, all of which had the lowest grub densities had at least 50% of positive samples contain Sg and no sampled containing Hm. The other sites were much more varied in species composition. All species were isolated from both the upper 5 cm and lower 15 cm of each soil core. Kaya et al. (1993) observed that Steinernema spp. occur near the soil surface and Heterorhabditis spp. are lower in the soil profile (Kaya et al. 1993). It is possible that these results are conflicting because the nematodes are responding to the presence of AGB grubs. In June, the grubs are found lower underground as they begin to enter pupation, while grubs in the fall are closer to the soil surface following harvest (personal observation).
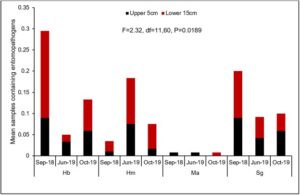
Overall there were significant differences in the number of samples containing each entomopathogen over the three evaluation periods (Fig. 7). In general, populations for Hb (Fig. 8A) and Ma (Fig. 9B) declined from September 2018 to June 2019, while Hm (Fig. 8B) increased and Sg (Fig. 9A) remained consistent. Northwest Ohio saw unprecedented rainfall events in Spring and early summer 2019 which left many fields inundated for days which extensively delayed and even prevented plantings for many farmers. Wauseon, OH saw at least 5” more rain in 2019 than the annual precipitation rate. During this time, the AGB adult population was only 2/3 that of what was observed in 2018. The excessive rains continued through the summer with July seeing more than 7” of rain. Ma (Fig. 9D) and Sg (Fig. 9C) populations remained constant through October 2019 while Hb (Fig. 8C) populations increased slightly and Hm (Fig. 8D) decreased. Hb (Fig. 8E) and Ma (Fig. 9F) were the only two entomopathogens to significantly decline from September 2018 to October 2019; the presence of Hm (Fig. 8F) and Sg (Fig. 9E) did not vary over time. These findings suggest that Hm does successful when soil moisture is high relative to the other entomopathogens, while Hb and Ma are highly susceptible to excessive soil moisture. Although soil moisture was not directly evaluated in this study, fields were observed to be oversaturated from May to July, with many fields supporting large ponds of water. If these environmental extremes become more routine, it may be necessary to select for species adapted to oversaturation, or drought, to ensure maximum efficacy and control.
The combination of variables assessed in the multiple regression analysis elicited a significant and slightly positive relationship (R2=0.054) between these variables, particularly site (t=0.11, df=1, P=0.0204), evaluation period (t=-2.89, df=1, P=0.0041), and crop either being soybean or corn (t=2.34, df=1, P=0.0196), with the average number of grubs per plot (F=2.89, df=7,352, P=0.0060). There were no significant relationships between the number of grubs and presence of each different entomopathogen species (P>0.05). Ma, which was not included in the analysis since it behaved colinearly with other variables, declined so much following the excessive rains of 2019 that it was barely isolated from samples in June and October 2019.
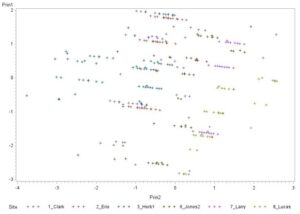
Principal component analysis (Fig. 10) was used to determine whether there were any consistencies among plots with varying numbers of grubs, or site location site were structured by a combination of the presence of different entomopathogen species, sand, silt and clay content, evaluation period, and crop. Since silt, clay, and Meterhizium anisopliae were colinear with other variables in the multiple regression analysis, they were discarded. Prin1, or principle component 1, was heavily weighted by evaluation period and crop. Prin2, or principle component 2, is weighted most by sampling location and the presence of sand, and to a lesser extent by the presence of Heterorhabditis bacteriophora. In general, the sites on this figure show some homogeneity as they overlap to a certain extent, although some sites lean further in one direction or the other.
Future directions. The resulting sequences will provide positive controls for us to confirm additional isolated nematode species. We will continue to sample each field for entomopathogens by taking 20 composite samples per field in June 2020 and Fall 2020. I am currently learning how to use GIS to accompany these analyses. A manuscript will be prepared from these results and submitted to PLOS ONE following completion of this work.
Objective 2. Rear isolated EPN species using established “in-vivo” techniques
Each species was successfully reared following the protocol established by Testa and Shields (2017). Each species is currently being reared/preserved in several ways: populations were established in vegetative areas around the OARDC in Wooster, OH. Autoclaved soil was inoculated with each species and stored in vented containers in the dark at room temperature. Nematode colonies are being maintained in waxworms in the lab. In order to maintain genetic variation and field-like attributes, nematodes will be sampled in the spring and augmented with those previously collected as recommended by Dr. Elson Shields. These species will be mass reared using waxworm cups prior to field trials in the spring.
Objective 3. Evaluate AGB susceptibility to these nematodes in corn
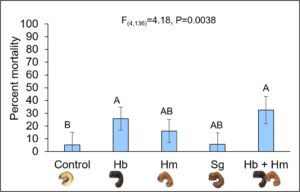
Using a conservative rate of ~11,824 nematodes per ft2, all four nematode treatments successfully infected and killed AGB, albeit at different rates (Fig. 11). (It is important to note that the number of grubs used in this study was a limitation as we are unable to rear the species in a controlled setting and we must drive 2-3 hours to collect grubs from the field. In addition, grubs are approximately the size of sand grains in the summer months, so we had to wait until mid-Fall to collect grubs that were a reasonable size to work with as our bait stations failed due to other animal species, which occurred around the time grubs were turning into adults). Hb and Hb + Hm treatments caused the highest mortality around 40% and were significantly different from the control which received no nematodes. Sg and Hm alone killed approximately 10% and 20% of grubs, respectively, but did not vary from the water control. Despite mortality rates below 50%, the rate used was only a fraction of what would be applied in a production setting, suggesting that actual field mortality rates may be much higher. Additional factors that may have impacted nematode infection rates (e.g. soil type and grub instar) were compared in separate two-way ANOVAs using proc reg in SAS 9.4; grub infection rates did not vary for sandy and loamy soils or among 2nd and 3rd instar grubs (P>0.05). These results were largely consistent with those evaluating commercially available nematodes against AGB in turf grass systems. Unlike these studies, our study isolated nematodes from the local environment as these species will be better adapted to local environmental extremes such as drought and temperature extremes and should not require annual augmentation into the field; previous studies in upstate New York showed that locally isolated EPN can reduce pest populations in field cropping systems and persist for over 7 years after initial application.
Figure 12. Average percent of grubs that died from EPN treatments including a water control, 1000 IJs of either Hb, Hm, or Sg, or 500 IJs of Hb and Hm, respectively. This experiment was conducted in the greenhouse with V2 organic corn plants grown in 32 oz deli containers. Letters denote significant differences among treatments from multiple comparisons tests.
Plant growth characteristics (root, shoot and total plant weights) were similar for plants of all treatments (Fig. 12). Interestingly, plant weights were numerically the smallest for Hb, one of the treatments that caused the highest AGB mortality. When looking at the roots and shoots of plants from this treatment, it was apparent that the grubs fed on the main roots closest to the kernel at a higher rate for this treatment than the others. This suggests there is likely a very low economic threshold level for AGB grubs in corn, thus necessitating the need for novel management tools like EPN, especially since insecticide seed treatments appear to be ineffective against this pest. These results were presented at the Entomological Society of America’s 2018 Annual Meeting in Vancouver, BC on November 12, 2018. I was recently invited by Paul Georgen from Pioneer Seed in Indiana to present these results to local farmers in north central Indiana on January 24, 2019. In addition, I will hold a workshop in 2021 to educate farmers in NW Ohio about EPN, how to mass produce them, and how to apply them using their own equipment. This workshop will accompany an annual Asiatic garden beetle meeting that I have held the past 2 years.
Although initially planned for the 2018 field season, the field evaluations will begin in 2020 using a microplot trial outdoors at the OARDC in Wooster, OH (Fig. 13). A grant extension was obtained due to a brief teaching appointment during Fall 2017 when this research was proposed to begin. In 2019, excessive rains drowned nearly 2/3 of the Asiatic garden beetle populations and prevented collaborators from planting until after the grubs pupated. The persistence and efficacy of EPN against AGB grubs (and other soil dwelling pests) will be evaluated in a microplot study in which microplots consisting of 12” tall PVC pipes with a 12” diameter placed in the ground will contain either sand or loam soil and receive a treatment of water, Hb, Hm, or Hb and Hm. A randomized complete block design with four replicates per treatment will be used for this study. Two organic corn seeds will be planted beginning of May and thinned following germination. Weeds and other pests will be managed per commercial recommendations. EPNs will be mass produced in the lab using containers of waxworms. They will be applied using a backpack sprayer that has the nozzle screens removed after plant. Depending on availability, at least 5 grubs and 5 waxworms will be placed in separate EPN bait stations in each microplot. The bait stations will be collected after 72 hours and any discolored grubs or waxworms will be placed on a White trap to isolate the EPNs for identification. Additionally, 3 soil cores will be taken and combined to create one composite sample per microplot in parallel with the baiting technique (Fig. 14). Every 3 months through Fall 2021 grubs (if able to collect) and waxworms will be placed in each microplot, and one composite sample taken, to monitor EPN persistence and efficacy over time. These results will be compiled into a manuscript in combination with the greenhouse trial to be submitted to the Journal of Economic Entomology following the completion of all work.
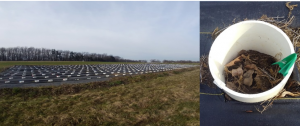
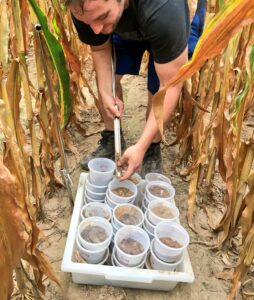
Educational & Outreach Activities
Participation Summary:
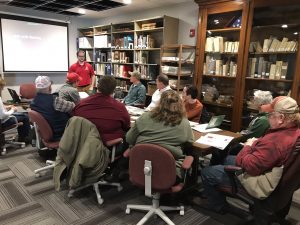
Prior to conducting the on-field nematode sampling I met and consulted with 9 individual farmers in 5 Ohio counties about my plans for research and answered any questions they had. I have disseminated my results from this research to extension educators, university researchers and local stakeholders in Wauseon, Ohio in 2019 and 2020 during an annual Asiatic garden beetle meeting. I have also presented my findings thus far at the Entomological Society of America’s annual meeting in Vancouver, BC in 2018. I was awarded 2nd place in the President’s Prize competition for this talk. I plan to present additional findings with respect to entomopathogen spatial and temporal distribution in agroecosystems at the 2020 Entomological Society of America North Central Branch – Southwest Branch joint meeting in Oklahoma City, OK in March.
In 2021, I plan to set up a workshop to teach interested farmers in the benefits and utility of EPN and how they can isolate, rear, and augment their own nematodes into their fields for biological control. This will be part of a larger Asiatic garden beetle meeting that I will be hosting in northwest Ohio for researchers, extension educators, and local stakeholders and growers from Ohio, Michigan and Indiana. At these events, I will have handouts prepared to answer basic questions about EPN in field crops. I also plan to write articles for Ohio State extension (C.O.R.N. newsletter, and eFields on-farm trial reports) about our findings which will be made available to the public.
Professional Presentations
Pekarcik, A., and K. Tilmon. March 2020. Seasonal relationships between naturally occurring EPN and Asiatic garden beetle, Maladera castanea, in Ohio agroecosystems. Entomological Society of America – North Central Branch & Southwest Branch Joint Meeting, Oklahoma City, OK.
*Pekarcik, A. and K. Tilmon. November 2018. Susceptibility of the Asiatic garden beetle, Maladera castanea, to locally isolated EPN. Entomological Society of America – Entomological Society of Canada Joint Annual Meeting, Vancouver, BC. *Received 2nd place for President’s Prize in IPM 3 section
Extension Publications
Pekarcik, A., E. Richer, and K. Tilmon. February 2020. EPN for control of the Asiatic garden beetle in field crops. Ohio State University Extension. 1-4. https://aginsects.osu.edu/sites/aginsects/files/imce/AGB_EPN_Handout_Feb2020.pdf
Project Outcomes
Due to excessive rains in 2019 which critically delayed planting until far past ideal planting dates and the hesitancy of farmers to apply entomopathogenic nematodes to their fields given their previous experiences with plant parasitic nematodes, we have not yet been able to implement these nematodes on a commercial scale for establishment of nematode populations and potentially subsequent control of Asiatic garden beetle and other pests. As a result, I have produced an extension bulletin that educates readers about entomopathogenic nematodes and how they can be isolated from a field and used on agricultural fields for an added management benefit. I also am conducting a microplot field trial in Wooster to assess the nematodes in a realistic setting to help convince farmers that these nematodes are beneficial and can potentially control their Asiatic garden beetle infestations. Farmers who attended the annual Asiatic garden beetle meetings held in 2019 and 2020 were given updates on the research conducted for this grant and any questions were answered and comments discussed. The results from this field trial will be presented at the 2021 Asiatic garden beetle meeting which will be open to extension educators, university researchers and staff, local stake holders, crop consultants, and industry reps.
I have gained much insight with respect to understanding farmers concerns and viewpoints, and the field of nematology. Farmers often deal with plant parasitic nematodes, which unlike entomopathogenic nematodes, can cause crippling crop losses and the innoculum can persist for years on end; the word nematode alone can cause farmers a lot of anxiety. The negative connotation of nematodes that stems from plant parasitic nematodes like soybean cyst nematode has caused us to reassess how we go about evaluating entomopathogenic nematodes, which do not cause any harm to plants, for Asiatic garden beetle grub control in field crops. As a result, I have produced an attractive bulletin that briefly discusses what entomopathogenic nematodes are and how they rely on insects, in addition to information regarding how to isolate nematodes from the field, mass rear them and apply them with standard spray equipment. I also will be conducting a preliminary field trial to assess efficacy and persistence of nematode treatments from 2020 to 2021 to reinforce to the farmers that these nematodes are truly beneficial and can help them manage Asiatic garden beetle in the long term. The cumulative results from these trials will be presented to farmers in 2021 at the annual Asiatic garden beetle meeting I host for extension educators, university researchers, and local stakeholders in Ohio, Michigan and Indiana.
The field of nematology is slowly dying as new faculty hires are forced to be multidisciplinary. I was fortunate to be able to visit Dr. Fred Warner at Michigan State University who is a nematologist who focuses on identification. He provided plenty of advice on the various ways to sample for and identify entomopathogenic nematodes and he recommended resources that have helped me along the way. He also provided feedback on my plans for this grant from the perspective of a nematologist. I have also communicated results with Dr. Elson Shields at Cornell University who has successfully used these techniques for the long term establishment of entomopathogenic nematodes in the field, and subsequent control of the Alfalfa snout weevil. He has provided knowledge on rearing the nematodes and maintaining genetic diversity in the populations to avoid the negative effects that stem from populations reared under laboratory conditions. From these mentors I have obtained critical skills that will enable me to contribute to nematology research; I have successfully isolated nematodes from the field using bait stations (albeit with a few issues with wildlife) and soil cores, then reared them in the lab and evaluated them against Asiatic garden beetle grubs in the greenhouse. I have begun working on the identification of the nematodes isolated from this research and have been looking into molecular barcoding to confirm the preliminary identifications.
Information Products
- Entomopathogenic nematodes for control of the Asiatic garden beetle in field crops (Bulletin)
- Susceptibility of the Asiatic garden beetle to locally isolated entomopathogenic nematodes (Conference/Presentation Material)