Final report for GNC20-315
Project Information
Wetland creation and restoration are an important part of nutrient management strategies in watersheds with intensive row-crop agriculture, because these systems are effective sinks for nitrogen, phosphorus and carbon sequestration. Many of the same environmental conditions that support the capacity of wetlands to capture nutrients also stimulate the release of methane (CH4) and nitrous oxide (N2O), which are potent greenhouse gases (GHGs) that drive climate change. Climate change is driving increased frequency and severity of intense rainfall events, including an increasing level of rain on snow and rain on snow. This threatens to increase the delivery of nutrients to downstream wetlands, creating a positive feedback. Thus, identifying wetland strategies that minimize releases of GHGs while maintaining water quality benefits. This project, “Design and Management of On-Farm Wetlands for Water Quality and Climate Regulation,” will address this demand through research and outreach efforts.
Two main factors of wetland design and management are vegetation and hydrology. Variability in nutrient retention, carbon sequestration, and GHG release have been linked to vegetation and water table management. Yet, there is considerable uncertainty about the mechanisms that drive these differences. Further, data on individual plant species, which could be used by managers to optimize plantings, are not available. This research addressed the uncertainties related to how wetland plant species influence microbial functions using twelve experimental mesocosms planted with one of three wetland species and a novel integration of biogeochemical methods. The effects of plant species were assessed using CH4, CO2, and N2O flux estimates and nutrient retention in the experimental mesocosms. The mechanisms that explain differences across treatments were identified by measuring soil and plant characteristics and rates of denitrification, methanogenesis, and N2O production. Multivariate analyses will be used to identify characteristics that explain the response of wetland functions to differences in plant species. A major outcome of this research will be the development of actionable guidelines for plant selection of wetlands that effectively treat agricultural drainage and runoff while reducing GHG emissions. The achievement of research and outreach outcomes will support progress toward reaching nutrient reduction goals while limiting contributions to climate change.
The primary learning outcome is to increase the actionable knowledge of how wetland plant species can be leveraged to maximize water quality and climate regulation benefits, which can inform the restoration efforts of land managers and wetland practitioners. These wetland practitioners may be employed by state and local government, NRCS, environmental consulting firms, and non-profit agencies that invest in wetland restoration, such as Ducks Unlimited and The Nature Conservancy. NRCS engineers and other wetland practitioners are the primary interface between county agencies, farmers, and non-profits who might be proponents of wetland adoption. A secondary learning outcome is to increase the knowledge and awareness of K-12 students of the role of wetlands in sustainable agriculture.
If the learning outcomes are effectively met, we anticipate the following actions: wetland practitioners will incorporate this guidance into future wetland designs to make planned wetlands more sustainable, farmers will adapt management of existing wetlands (e.g. planting or removing certain plant species) based on management guidance to make wetlands more sustainable, and more K-12 students will consider pursuing careers in sustainable agriculture and environmental science. Although these action outcomes are anticipated, tracking these changes in behavior is beyond the proposed scope of the project.
Cooperators
- (Researcher)
- (Researcher)
- (Researcher)
Research
Due to COVID-19 and personnel changes, progress on this project was considerably delayed. Data collection began in Fall 2022 and resumed data collection during the growing season in 2023.
Mesocosms were placed outdoors in gravel-lined depressions at Purdue Wildlife Area (Figure 1). Placing mesocosms in the ground insulates them, reducing temperature extremes and keeping mesocosm temperatures in line with natural wetlands. These mesocosms are just outside of a new Purdue University laboratory facility built at Purdue Wildlife Area. The well for this facility is the source of water for the experiment.
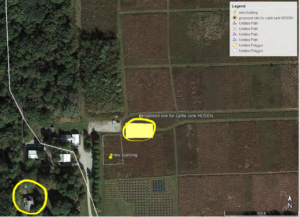
Soil from the depressional wetland at Purdue Wildlife Area was split across twelve mesocosms (Figure 2). Due to the project's delays, the scope was narrowed from what was first proposed to only focus on the impacts of plant species, rather than concurrently assessing the impacts of water table management. Water levels in the mesocosms were kept at a fixed depth outside of the growing season, which is representative of the hydrologic zones where the selected plants tend to thrive. This water depth was maintained during the growing season. Mesocosms were planted with one of three vegetation types (Reed Canary Grass, Rice Cutgrass, and Broadleaf Arrowhead) or no vegetation (control) in Spring 2023 (Figure 3).
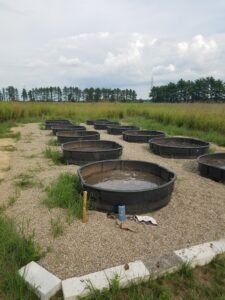
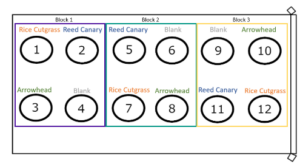
Sample Collection: A fertilizer runoff event was simulated by stirring in a concentrated solution of sodium nitrate (NaNO3) so that the final water column concentration in all mesocosms was 10 mg NO3-N/L. Soil samples were collected before planting, three weeks before the simulated runoff event (6/26/2023), the day after the event (7/17/2023), and two weeks after the event (7/31/2023). Sample times were chosen to evaluate baseline performance, performance under NO3-enriched conditions, and system recovery after a nutrient influx. Soil samples were collected to a depth of 30 cm from five random spots in each mesocosm using a stainless steel soil probe with a 2-cm inner diameter. For each sampling event, soils from the same mesocosm were homogenized and stored in a cooler with ice while transported to the laboratory for analysis.
Assessment of Denitrification Potential: Denitrification potential was characterized using acetylene-block denitrification enzyme assays (DEA) (Groffman et al., 1999; Tiedje et al., 1989). Optimal conditions for denitrification were created under short-term laboratory conditions by incubating soils under anaerobic conditions and adding NO3- (47.2 mg NaNO3/L) and labile organic carbon (60.7 mg C6H12O6/L) to alleviate rate limitations imposed by substrate availability. Chloramphenicol was added to each bottle to prevent additional enzymes from being synthesized in response to substrate additions (Bernot et al., 2003). Acetylene gas (C2H2) was introduced such that headspace was initially 10% C2H2 and 90% N2, which inhibits the final step of denitrification, resulting in the buildup of nitrous oxide (N2O), the product of incomplete denitrification. The buildup of N2O was measured by taking headspace samples through a septum in the bottle lid hourly for 5 hours and analyzing N2O concentration on a Shimadzu Gas Chromatograph (GC) (GC-2014, Shimadzu Corporation, Kyoto, JP). Ideal Gas Law and Henry’s Law were used to estimate the total N2O-N mass in the headspace and slurry based on headspace concentration measurements. Linear models were then used to estimate potential denitrification rates for each bottle. Finally, each rate was normalized to the oven-dry soil mass of each bottle.
Assessment of System GHG Flux: GHG flux was determined by taking gas samples from floating flux chambers. GHG flux sampling took place on the same dates as soil sampling for DEAs, with flux measurements being made before soil collections so that any soil disturbance caused by sample collection would not impact flux measurements. The flux chambers were constructed from 5-gallon high-density polyethylene buckets with a 3-way stopcock affixed to the top of the unit for sampling via a syringe. When placed on the water, these chambers have a headspace volume of 13.55 L and cover 660 cm2 of the water surface. Flux chambers were placed over vegetation in the mesocosms. The stopcock was left open during placement to allow air pressure to equilibrate. Once equilibrated, an initial baseline gas sample was taken, and the stopcock was left closed, except when pulling gas samples. Subsequent samples were collected every 15 minutes for 1 hour. Samples were analyzed for CO2, CH4, and N2O concentrations on the same GC used for the DEAs. Results are reported as milligrams of C- CO2, C-CH4, and N-N2O respectively, per hour per square meter.
Potential explanatory soil and plant properties: Different soil and plant properties were characterized to investigate potential drivers of differences in water quality improvement and GHG emissions between plant types. Soil properties included soil organic carbon concentration (cold-water extraction), organic matter content (gravimetrically via loss-on-ignition), concentrations of exchangeable soil NO3- and ammonium (NH4+) (potassium chloride extraction), carbon and nitrogen contents (elemental determination via combustion). Water-extractable soil organic carbon was further characterized by collecting fluorescence excitation-emission matrices (EEMs) with a spectrofluorometer (HORIBA Aqualog, HORIBA Scientific, Edison, NJ, USA). Dried plant biomass samples were analyzed for carbon, nitrogen, lignin, cellulose, and hemicellulose.
Statistical Analyses: A linear mixed-effects model was used to assess the impact of plant type on potential denitrification rates (i.e., DEA). This model included plant species, nitrate conditions (i.e., before the spike, one day after the spike, or two weeks after the spike), and phase of the plant life cycle (i.e., measurements taken during the growing season or while the plants were senescent) as fixed effects. Mesocosm location was incorporated as a random effect by nesting the mesocosm block and unique identification number. Pairwise comparisons were made using a Tukey test to test for differences in the rates between levels of each factor. Pairwise comparisons of denitrification rates between plant types were performed for each nitrate condition, although future pairwise analyses will be performed using estimated marginal means. The mesocosm location did not have a significant effect on the variance of GHG flux rates, so linear mixed with only the fixed effects were fitted for N2O, CO2, and CH4 fluxes. A significance level (α) of 0.05 was applied for all analyses.
Collectively, plant type, seasonal status, and NO3- enrichment level explained 49% of the variance in potential denitrification rates (conditional R2). Soils planted with reed canary grass consistently supported the highest mean potential denitrification rate across NO3- enrichment level and seasonal status, followed by arrowhead, rice cutgrass, and the unplanted mesocosms. Across NO3- enrichment levels and seasonal status, reed canary grass consistently had significantly higher potential denitrification rates than unplanted soils. Differences in potential denitrification across plant types were generally limited during the growing season, although reed canary grass supported significantly higher rates than arrowhead within two weeks of the simulated runoff event. Differences in denitrification between plant types were more evident during the senescent phase.
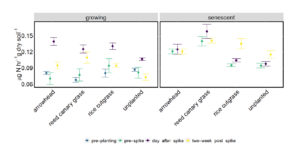
CO2, CH4, and N2O emissions were typically highest in mesocosms planted with rice cutgrass, while the unplanted mesocosms had the lowest emissions for each GHG. Rice cutgrass supported significantly higher CH4 emissions than the unplanted mesocosm, although no other significant differences were found among plant types. All planted mesocosms had significantly higher CO2 emissions than the unplanted treatment, but differences between plant types were limited. No significant differences in N2O emissions were observed between plant treatments.
Figure 5: Impact of plant type on GHG fluxes from wetlands soils
Wetlands have an exceptional capacity to intercept and treat excess N from agricultural production, yet the anoxic conditions and organic carbon-enriched soils of wetlands lead to microbial production of GHGs. Wetlands in agricultural watersheds should be designed and managed to maximize water quality improvement while minimizing impacts on climate. These preliminary findings indicate that plant selection may be a key design choice in engineering agricultural wetlands to maximize N removal but limit GHG emissions. Arrowhead seems ideal for planting in wetlands with high NO3- loading, because mesocosms planted with arrowhead generally supported comparable potential denitrification as other wetland plant species but had lower GHG emissions than both rice cutgrass and reed canary grass in response to a simulated runoff event. By contrast, rice cutgrass often had similar potential denitrification as other plant types but supported the highest CH4, CO2, and N2O emissions among plant types.
Contrasting denitrification and GHG emissions of planted and unplanted mesocosms further highlights the role of wetland plants in regulating tradeoffs between N removal and GHG emissions. Denitrification was consistently higher in planted mesocosms. Despite wetlands being important ecosystems for carbon sequestration, denitrification in wetlands in agricultural watersheds has been reported to be limited by the availability of labile organic carbon in some cases (Kayranli et al., 2010; Grebliunas and Perry, 2016). Increased availability of labile organic carbon via root exudation and litterfall may stimulate higher denitrification in planted mesocosms (Hang et al., 2016). Several studies have also reported lower CH4 emissions from unplanted wetland soils, although these lower rates have been attributed to shunting of CH4 to the atmosphere through vascular tissues in plants (e.g., Li et al., 2018). Wang and Reid (2020) similarly demonstrated that root-mediated gas transport accounts for a notable portion of N2O emissions from wetland soils. Arrowhead supported the lowest GHG emissions in this experiment, although both broadleaf arrowhead (e.g., Smith & Luna, 2013; Wang & Reid, 2020) and reed canary grass (e.g., Zedler & Kercher, 2004) have been cited as having higher potential for plant-mediated transport than other wetland species. Next steps for this project will include identifying plant properties that help explain differences in GHG emissions and denitrification across plant types by using multivariate analyses to link plant and soil properties with GHG emissions and potential denitrification. With an increased understanding of the mechanistic drivers of differences in environmental quality outcomes across plant types, wetland managers and designers will ideally be able to select for certain plant properties to promote denitrification while constraining GHG emissions.
References:
Grebliunas, B. D., & Perry, W. L. (2016). Carbon limitation of sediment bacterial production and denitrification in high nitrate low carbon systems. Environmental Earth Sciences, 75(8), 662. https://doi.org/10.1007/s12665-016-5464-1
Hang, Q., Wang, H., Chu, Z., Ye, B., Li, C., & Hou, Z. (2016). Application of plant carbon source for denitrification by constructed wetland and bioreactor: Review of recent development. Environmental Science and Pollution Research International, 23(9), 8260–8274. https://doi.org/10.1007/s11356-016-6324-y
Kayranli, B., Scholz, M., Mustafa, A., & Hedmark, Å. (2010). Carbon Storage and Fluxes within Freshwater Wetlands: A Critical Review. Wetlands (Wilmington, N.C.), 30(1), 111–124. https://doi.org/10.1007/s13157-009-0003-4
Li, Y., Wang, D., Chen, Z., & Hu, H. (2018). Comprehensive effects of a sedge plant on CH4 and N2O emissions in an estuarine marsh. Estuarine, Coastal and Shelf Science, 204, 202–211. https://doi.org/10.1016/j.ecss.2018.03.008
Smith, K. E., & Luna, T. O. (2013). Radial Oxygen Loss in Wetland Plants: Potential Impacts on Remediation of Contaminated Sediments: Natural Treatment Systems. Journal of Environmental Engineering (New York, N.Y.), 139(4), 496–501.
Wang, S., & Reid, M. C. (2020). Kinetics of nitrous oxide mass transfer from porewater into root aerenchyma of wetland plants. Journal of Environmental Quality, 49(6), 1717–1729. https://doi.org/10.1002/jeq2.20162
Zedler, J. B., & Kercher, S. (2004). Causes and Consequences of Invasive Plants in Wetlands: Opportunities, Opportunists, and Outcomes. Critical Reviews in Plant Sciences, 23(5), 431–452. https://doi.org/10.1080/07352680490514673
Educational & Outreach Activities
Participation Summary:
We introduced K-12 students throughout the United States and Canada to water quality challenges related to agriculture and conservation practices that reduce the water quality impacts of agriculture, particularly on-farm wetlands, through volunteering with Skype-A-Scientist, Pathful Connect (previously Nepris), and Illinois-Indiana Sea Grant's Students Ask Scientists program and working with Purdue University Extension 4-H Youth Development. We also presented the role of wetlands in water quality and the findings of this project to practitioners and other researchers. The details of these virtual presentations are presented below:
- Discussed careers in ecological engineering and presented my plans for investigating on-farm wetlands with an AP Physics class at Parkland High School in Winston-Salem, North Carolina (October 15, 2020) [presentation]
- Discussed water quality benefits from agricultural wetlands and tradeoffs in agricultural wetlands, specifically greenhouse gas production and losses of legacy phosphorus, and outlined my upcoming research on on-farm wetlands with the IB Environmental Systems and Societies class at Parkland High School in Winston-Salem, North Carolina (October 28, 2020) [presentation]
- Presented strategies for nutrient reduction in agricultural watersheds, including on-farm wetlands, to 7 classes at Indian River High School in Philadelphia, New York, through Students Ask Scientists. I also consulted with this class on their research project that investigated why excess nutrients were present in a local reservoir (2 classes on April 22, 2021; 3 classes on April 23, 2021, and 2 classes on April 27, 2021) [7 presentations]
- Hosted a hands-on Earth Day activity virtually for kindergarteners and 1st graders for a chapter of Girl Guides of Canada in Vancouver, Canada through Skype-A-Scientist. This activity taught the girls about forms of pollution that come up from agriculture, industry, and urban areas (May 13, 2021) [presentation]
- Worked with 7th and 8th grade students on their design projects (The Science of Sustainability in Building an Earth Friendly Theme Park) and discussed my research into sustainability, including on-farm wetlands, through Skype-A-Scientist (May 27, 2021) [presentation]
- Virtually visited a 5th grade class in Edmonton, Alberta who just finished studying wetlands and discussed wetlands and other conservation practices in urban and agricultural settings (June 23, 2021) [presentation]
- Presented information on the use and investigations of wetlands to treat pollution with an undergraduate researcher at Worcester Polytechnic Institute in a one-on-one session through Skype-A-Scientist (December 1, 2021) [presentation]
- Served as a technical expert to answer questions about more than 90 9th-grade students' projects related to sustainable agriculture in the Nepris session Global STEM Challenges Program: Food Production/Waste Reduction. I also gave a presentation on different nutrient loss reduction strategies in agriculture from in-field practices, such as precision fertilizer application and cover cropping, to edge-of-field practices, such as riparian buffers and on-farm wetlands, to downstream practices, such as riverine floodplain restoration (February 4, 2022) [presentation]
- Virtually visited a 7th grade class at Sashabaw Middle School for their career day via Nepris. I discussed career opportunities in conservation and conservation practices in agricultural settings (March 23rd, 2022) [presentation]
- Shared information on how plants and soils treat pollutants with hands-on activities at Purdue University's 2022 Spring Fest. Spring Fest is an annual festival for local students and their families. I also presented a poster on wetlands (What are wetlands?) to hundreds of visitors. [other educational activities]
Hundreds of children and their families learned how plants and soils treat and trap pollution in wetlands at 2022 Spring Fest at Purdue University. - Worked with the School of Agricultural and Biological Engineering to host three Career Day sessions during 4-H Round-Up. We led middle school students through a hands-on activity of testing water samples and discussed agricultural conservation practices, including wetlands (June 27, 2022) [other educational activities]
- Led a design challenge for INSPIRE engineering summer camp students (ages 9-11) where students were tasked with developing a filter prototype for treatment wetlands (July 14th, 2022) [workshop]
INSPIRE summer camp students learn about water quality and treatment wetlands before designing a filter prototype - Presented information about the water quality benefits of restorations during the Indiana Wetlands Educational Outreach Workshop, which was hosted by the Silver Jackets. The Indiana Silver Jackets is a partnership of federal, state, and local organizations that focus on the mitigation of flooding and other natural hazards (July 18th, 2022) [workshop]
Silver Jacket workshop attendees learn about wetland functions and restorations - Led a design challenge for the Algebra before 7th Grade program where students were tasked with developing a filter prototype for treatment wetlands (October 29th, 2022) [workshop]
- Used wetland mesocosm studies as an example to teach experimental design in a sophomore-level course in agricultural and biological engineering (ABE 205: Computations for Engineering Systems). I presented the class with the research problem that this proposal addresses and asked them to design an experiment to determine the effect of plant type on wetland function (Fall 2022) [other educational activities]
- Used the mesocosm design for this project to teach about randomization and controlling extraneous variables during experiments in a sophomore-level course in agricultural and biological engineering (ABE 205: Computations for Engineering Systems) (Fall 2022) [other educational activities]
- Led a student team in analyzing and presenting data from a meta-analysis of water quality treatment in agricultural wetlands for their course project in ABE 205, Computations for Engineering Systems. This team presented their findings as a poster in the ABE Sophomore Showcase (Fall 2022) [other educational activities]
- Created and distributed a lesson plan for more than 100 4-H educators in Indiana on how wetlands work as sponges in the landscape to store water and trap/treat pollutants in the landscape. This lesson plan included additional activities to learn more about experimental design and a modification to use the activity with Enviroscape Ecosystem Restoration kits (February 2023) [education tool]
- Worked with the Department of Agricultural and Biological Engineering to host an educational activity using the Enviroscape Ecosystem Restoration model to demonstrate the impacts of wetlands on water quality and flooding and to share other ecosystem services of wetlands through an educational poster (What are wetlands?), filtering activity, and plant uptake model with hundreds of visitors during Purdue University's 2023 Spring Fest (April 15, 2023) [other educational activities]
- Team representative presented the sampling plan and hypotheses at the 2023 meeting of the American Ecological Engineering Society in Tampa, FL, as a poster - Evaluating the influence of plant community composition on methane and denitrification rates in wetland mesocosms (June 6-9, 2023) [presentation]
- Facilitated a field tour with hands-on activities to explore design features of ecosystem restoration and practices for monitoring restored ecosystems for the Engineering the Environment workshop during 4-H Academy at Purdue University (June 8, 2024) [workshop]
- Demonstrated the impact of wetland restoration on water quality and flood mitigation with the Enviroscape Ecosystem Restoration model in the Purdue Extension building for Indiana State Fair attendees (August 16, 2023) [other educational activities]
- Visited Eastern Greene Elementary School to watch and provide feedback on capstone presentations for the Conservation and the Human Impact of the Water Cycle program, which is supported by the Greene County 4-H program and addresses the importance of wetlands (December 12, 2023) [other educational activities]
- Collaborated with other Extension professionals to host a virtual session on wetlands for the Forces of Nature Spark Club, which engaged 24 4-H members from across Indiana (January 17, 2024) [other educational activities]
- Hosted two sessions for 21 4-H members that introduced different types of water pollution and demonstrated the impact of wetland restoration on water quality in urban and agricultural watersheds with the Enviroscape watershed models at the January session of the Forces of Nature Spark Club (January 20, 2024) [other educational activities]
- Team representative presented preliminary findings from the project at the W3 Seminar at the University of Florida in Gainesville, FL as a presentation - Wetland restoration strategies in agricultural landscapes for multi-ecosystem functions (2024) [presentation]
This project has also been featured in two press releases. The Department of Forestry and Natural Resources at Purdue University released a press release that discussed this work and associated research under a USDA National Institute of Food and Agriculture (NIFA) grant: Hosen to Study Wetland Usage for Nutrition Retention, Greenhouse Gas Minimization. Agriculture News from the College of Agriculture at Purdue University released an article on recipients at Purdue of NC-SARE funding, which included my project: SARE grants to support Purdue's sustainable agriculture programs.
Project Outcomes
We anticipate that this project will yield recommendations for planting vegetation types in wetlands within agricultural watersheds that balance water quality enhancement with greenhouse gas emissions.
During this project, I increased my knowledge of how wetlands can be engineered to limit the water quality and climate impacts of intensive agriculture. This project also provided opportunities for me to strengthen my project management and mentorship skills. Through extensive K-12 outreach efforts during this project, I also gained an awareness of the critical need to recruit future environmental professionals to address emerging challenges in sustainable agriculture.