Final report for GNC21-331
Project Information
Groundwater irrigation has been regarded as critical to the sustenance of agricultural production in the U.S. Midwest. Due to continuous extraction, many agricultural regions with heavy reliance on groundwater irrigation have witnessed substantial drawdown or depletion of groundwater reservoirs. To develop effective coping strategies, it is critical to have a thorough understanding of the groundwater system and its interaction with groundwater users and future challenges such as climate variability and change. Thus, this project aims to develop a socio-hydrological modeling framework for examining the sustainability of aquifers in irrigation agricultural landscapes and evaluating potential solutions to improve resilience in a changing climate.
This project will develop a transferrable modeling framework that can: (1) characterize both hydrologic dynamics and producers’ irrigation behaviors, (2) integrate hydrologic and social-behavioral models to represent the integrated system dynamics, and (3) evaluate groundwater sustainability under future climate scenarios. The modeling framework will couple (a) an agent-based model (ABM) for simulating irrigation behaviors in response to physical constraints and hydrological models for characterizing surface processes and groundwater dynamics. Such a framework can be used to examine the interactions between human and water systems with incomplete or ambiguous information on irrigation decision making. The ABM allows the representation of complex decision-making processes by defining collective actions using predefined behavioral rules. Using a Central Platte River basin as the testbed, the Cooperative Hydrology Study (COHYST) groundwater model and a Soil & Water Assessment Tool (SWAT) model developed by the applicant will be utilized to simulate groundwater storage dynamics and to model groundwater recharge rate at different spatial and temporal scales. The ABM shall then be coupled with the hydrologic models to represent the integrated system dynamics. The project is expected to produce outcomes that will be critical to sustainable agriculture in the North Central Region. The expected outcomes encompass tools and information that will help increase awareness, change attitudes, and inform decisions and policies in groundwater management and sustainability. It will improve the understanding of the effects of potential agricultural and water management options on groundwater storage, and allow a better design of intervention strategies to mitigate foreseeable climate impacts on groundwater depletion.
The project outcomes include (1) the critical information that links producers’ irrigation behaviors to the prospect of groundwater sustainability, and (2) a new decision-making tool that allows natural resources agencies to evaluate the impact of future climate and the effectiveness of groundwater management options. First, we expect the producers to increase their awareness of risks to groundwater sustainability imposed by unrestrictive pumping activities and future climate change. Using a range of project dissemination avenues (e.g., local board meetings, flyers, farming group emails, and social media), we expect to change the perception and attitudes of producers towards groundwater resources: from an unlimited reservoir to a limited and vulnerable resource that requires proactive protection. Second, we expect the state and regional natural resources agencies to use the modeling framework as a tool to inform water management options and intervention strategies to mitigate groundwater depletion. Our project findings are expected to facilitate more effective science-based policies to maximize the crop production benefits while allow sustainable water use over short and long terms. This will result in shared groundwater sustainability goals of different conservation programs and policies being put in place for the North Central region.
Research
In my research, I use a socio-hydrological framework to examine groundwater and how people interact with it and make decisions related to it. In theory, socio-hydrological modeling frameworks should assess how humans and groundwater co-evolve, interact, and respond under different circumstances and challenges. In practice, however, most modeling frameworks fail to adequately represent the intertwined groundwater and human systems, especially human behavior due to the use of over-simplified models.
To address this gap, my research combines both natural science and social science methods. I do this by integrating hydrologic models with an agent-based model. As shown in the figure below, it features a social system model (Agent-based Model) for characterizing behaviors of groundwater irrigation and coupled watershed-aquifer models (Soil and Water Assessment Tool (SWAT) and MODFLOW) for modeling surface water and groundwater interactions. The construction of the ABM shall be informed by interviews and surveys to adequately capture and represent human decision-making.
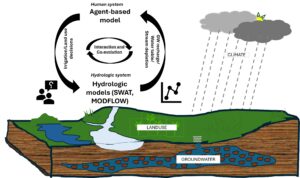
The Soil and Water Assessment Tool (SWAT)
This framework accounts for the feedback and dynamics within and between the human and the water systems. It also makes it possible to make projections and reconstructions to test different ‘what if’ scenarios. One section of this framework was utilized to study the impacts of cover crops and climate change on water dynamics in our study area. The objective of the study was to improve the understanding of the long-term impacts of cover crops in combination with climate change on agricultural water management in an agricultural system following a hydrological modeling framework. To achieve this objective, the Soil and Water Assessment Tool (SWAT) modeling and water footprint analyses were conducted.
The SWAT model was developed for the Central Platte River Basin for the purposes of simulating and evaluating the hydrological system in terms of both water quantity and quality. The Soil and Water Assessment Tool (SWAT) model (Version 2012) was used to cover the 27-year period from 1989 to 2015. Key inputs to the SWAT model included weather (i.e., precipitation, solar radiation, temperature, relative humidity, and wind speed) data, topography, soils data, and land cover (Table 1), as well as typical land management practices in the region. All the inputs were downloaded from either state, national or academic agencies. The coordinate system for all the input data was set to North American Datum 1983 (NAD83) Universal Transverse Mercator Zone 14 N.
Table 1: Data and sources
Data Type |
Resolution |
Source |
Variable |
Climate |
Daily |
HPRCC AWDN PRISM https://gdo-dcp.ucllnl.org/downscaled_cmip_projections |
Precipitation(mm/d), maximum and minimum temperature (0C), solar radiation (MJ/m2), relative humidity (fractional), and wind speed (m/s) Future climate projections (Precipitation(mm/d), maximum and minimum temperature (0C)) |
Topography |
30 m |
USDA-NRCS National Elevation Data 30 meter |
30-meter Digital Elevation Model (DEM) |
Land use |
30 m |
Cropland Data Layer & UNL CALMIT data (1982) |
Crop types |
Soil |
10 m |
USDA/NRCS Geospatial Data Gateway |
SWAT US SSURGO soils Database; gSSURGO spatial and tabular data
|
Streamflow |
Daily |
Gages from U.S Geological Survey, NeDNR, and the COHYST project |
Flow (m3/s) |
The SWAT model requires a wide range of inputs including spatial data and weather data. Table 1 shows the provided data and respective sources for the case study. It includes climate, agriculture management, as well as remotely sensed spatial (e.g. DEM, soil and land use) information.
In this study, the management practices selected to represent the agricultural conditions were fertilizer application, irrigation, planting operation, harvest and kill operations as well as the incorporation of rye after cash crop (corn and soybean) harvest. Planting and harvesting dates were obtained from literature and communication with local experts. Irrigation and fertilizer application were set up using the automatic option. Therefore, irrigation events were triggered by water stress threshold (soil moisture deficit) and fertilizer was applied based on nutrients stress factor. Auto fertilizer and auto irrigation options of the SWAT model were used based on the assumption that farmers were using good management practices.
Table 2: SWAT model cover crop scenarios
SWAT model scenario |
Year |
Operation |
Crop |
Date |
|
Month |
Day |
||||
Baseline (NWCC) |
1 |
Plant begin |
Corn |
May |
15 |
|
1 |
Harvest and kill |
Corn |
October |
10 |
|
1 |
Plant begin |
Soybean |
May |
15 |
|
|
Harvest and kill |
Soybean |
October |
15 |
Winter cover crop (WCC) |
1 |
Plant begin |
Corn |
May |
15 |
|
1 |
Harvest and kill |
Corn |
October |
10 |
|
1 |
Plant begin |
Cereal rye |
October |
15 |
|
1 |
Harvest and kill |
Cereal rye |
May |
10 |
|
1 |
Plant begin |
Soybean |
May |
15 |
|
1 |
Harvest and kill |
Soybean |
October |
15 |
|
1 |
Plant begin |
Cereal rye |
October |
15 |
|
1 |
Harvest and kill |
Cereal rye |
May |
10 |
This study assessed 38 General Circulation Models (GCMs) from the Fifth Coupled Model Intercomparison Project (CMIP5; Taylor et al. 2012). This represented the set of GCMs that were available, contained daily data from 1950 to 2100, and included historical and future outputs for both the moderate and high representative concentration pathways (RCP4.5 and RCP8.5, respectively; Moss et al. 2010; Knutti 2014). GCM projections of temperature change were calculated as absolute differences (future - current) while projections of future precipitation are calculated as relative changes of the current period (future/current * 100%). The annual precipitation and temperature changes were calculated for the period between 2030−2050, referenced to 1950−1980.
Table 3: Selected representative GCMs
Classification |
Climate Model |
RCP |
Precipitation |
Temperature |
Hot & Wet |
canesm2.1. |
4.5 |
38% |
2.2 |
8.5 |
41% |
1.3 |
||
Cool & Dry |
inmcm4.1. |
4.5 |
30% |
1.9 |
8.5 |
14% |
2.1 |
||
Hot & Dry |
ipsl-cm5a-mr.1. |
4.5 |
28% |
2.3 |
8.5 |
8% |
3 |
||
Central Tendency |
mpi-esm-mr.1. |
4.5 |
34% |
2 |
8.5 |
32% |
2.5 |
||
Cool & Wet |
mri-cgcm3.1. |
4.5 |
30% |
0.4 |
8.5 |
33% |
0.7 |
The SWAT modeling framework was utilized in two studies to help understand how water resources respond to different changes in the natural system. To represent the future climate, downscaled data from five Global Climate Models (GCMs) were utilized in this study. Broadly, we examined the following:
1. Sustainable cropping practices and their impacts on the water balance
The purpose of this study was to use a hydrological modeling framework (Figure 2) to evaluate the long-term impacts of cover crops on water availability under different climate scenarios.
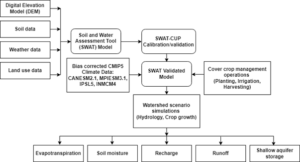
We assessed the impact of introduced winter cover crops on the water flows and fluxes in the watershed under study. We hypothesized that the introduction of an over the winter cover crop with spring termination should influence these variables than the scenario without a cover crop. To address this objective, the SWAT model was set up to assess the effects of cover crops and climate variability on the water flows and fluxes in a regional agricultural watershed by assessing how different water balance components were impacted (Figure 3)
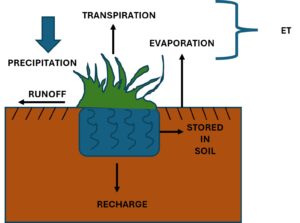
2. Agricultural water management (AWM) and how it affects the resilience of watersheds
Given the knowledge gaps in cover crops and Agricultural Water Management (AWM), it is vital to thoroughly assess the long-term impacts of cover crop installations on water cycles associated with AWM. This study aimed primarily to investigate the impacts of installing cover crops for agricultural systems and their impacts on the resilience of such a system. We examined one of the most utilized cover crop species, Cereal rye (Secale cereale), introduced during the winter period following the harvest of the main crops. This study used hydrological model simulations and water footprint accounting framework (Figure 4) to investigate the effects of using cover crops as an AWM strategy and rebound effects on water resources sustainability. A combination of the comprehensive simulation using the SWAT model and water footprint sustainability indices was employed in an agricultural watershed (Middle Platte watershed) to perform the evaluations.
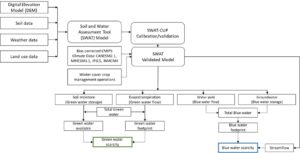
The SWAT model outputs were utilized to assess the impacts of cover crops and climate change on water dynamics. The figures below illustrate the components used in our analysis as well as the procedure:
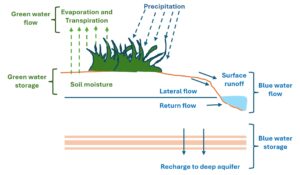
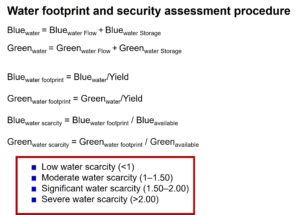
Groundwater Model - Cooperative Hydrology Study (COHYST)
This study developed Python-based FloPy scripts to interact with the COHYST MODFLOW groundwater model. This involved several steps to ensure compatibility and accuracy when handling input and output files. The COHYST model, critical for groundwater management in Nebraska, uses the USGS MODFLOW software, which relies on formatted input and output files structured using Fortran-type format descriptors.
To access and utilize the model effectively, FloPy, an open-source Python library for MODFLOW, was employed. The COHYST MODFLOW model input files were loaded into FloPy using functions such as flopy.modflow.Modflow() to read and interpret the model's configuration. This step required careful handling of directory structures and ensuring all required model files (e.g., NAM, DIS, BAS, and NWT files) were accessible.
MODFLOW's reliance on Fortran-type format descriptors for interpreting input/output files necessitated resetting the format in certain cases. Using FloPy's ArrayOutput and related tools, modifications were made to ensure proper alignment of data read/write operations, adhering to the structured formatting standards described in MODFLOW documentation. This step addressed common challenges, such as incorrect alignment of numerical data or truncation issues during file reading or writing.
To ensure the COHYST model was accurately replicated and operational within the FloPy framework, debugging was conducted using FloPy's plotting and visualization tools. Once the COHYST model was successfully integrated, FloPy was used for sensitivity analyses and scenario testing. Test simulations with varying input parameters (e.g., recharge rates, pumping rates) were run, leveraging the COHYST model's calibrated structure.
This approach provided a flexible and efficient means of interacting with the COHYST MODFLOW model while maintaining the integrity of its original Fortran-based data structures. The use of FloPy facilitated visualization and analysis of results, enhancing the model's applicability for groundwater resource management in the region.
Social System Model - Agent-based Modeling in Mesa
The development of the social system model involved a number of steps. To develop the Agent-Based Model (ABM) that represents local irrigation decision-making, we designed and conducted interviews and mail surveys following a Theory of Planned Behavior (TPB) framework. TPB, developed by Ajzen (1991) poses that the intention to perform is dependent upon three constructs: attitude, subjective norms, and perceived behavioral control. The theory’s concise and efficient structure makes it ideal for analyzing decision-making behaviors from information collected from the study area through interactions with stakeholders via interviews and surveys.
A sample (200) of the research subjects was approached for interviews during the development of the survey instrument. Based on their insights, the survey was developed, revised and updated in preparation for the first round of mailing. We used our data and relevant literature (e.g., irrigation pumping records, published survey results) to refine behavioral rules of irrigators and the causal relations that lead to irrigation decisions, which was ultimately used to inform the design of ABM.
Data collection techniques and study population
Our CPNRD study has been approved by the Southern Illinois University - Carbondale Human Subjects Committee (HSC). We used an exploratory, sequential, mixed-methods approach for optimum analysis as well as robust understanding of issues. Therefore, while quantitative data collection and analysis was prioritized, qualitative methods were also used to shape the questions and statements in the final instruments (mail survey and interview script). This approach followed the Ajzen, (2006) recommendations to collect qualitative data about underlying beliefs before developing the final survey. This aids in expanding the understanding of what considerations involving irrigation decision-making are of importance to the target audience. This information was then used to develop the quantitative instrument, a mail survey that was pre-tested. In the subsequent stage of data collection, the survey was designed such that it would help expand the understanding of intentions of conserving groundwater by sampling a large number of people in a statistically representative sample.
Data was collected in several phases; the first qualitative phase of the study aimed to help us garner beliefs regarding groundwater use and conservation among a small sample of farmers in the study area. 200 farmers were randomly selected and invited to participate in interviews/discussions. An interview guide with standardized open-ended questions was utilized to prompt behavioral, normative, and control beliefs related to irrigation decision-making. During this stage, the survey was also pre-tested to determine whether additional variables could be identified that were absent in the initial survey instrument. The outcome of these interviews was used to inform the development of a survey instrument for use in the second phase of the study. The survey instrument designed in accordance with the TPB was used to quantitatively evaluate factors that influence farmers’ intentions to engage in water saving behavior in a larger sample of farmers. The survey also contained demographic questions to provide context for the result analysis.
The second phase included a mail survey of farmers in CPNRD basin and follow-up interviews with willing participants. This research was designed and implemented as a cross-sectional survey to collect data from a sample of the population in the study area. We surveyed farmers during the spring-summer of 2023 in selected counties within the Central Nebraska region (McPherson, Lincoln, Logan, Custer, Dawson, Gopher, Frontier, Phelps, Kearney, Adams, Hamilton, Buffalo, Nance, Platte, Butler, Polk, Hall, Howard, and Merrick). A sample of 1,000 addresses was purchased from a reputable company that specializes in farmers and ranchers’ databases in the US. Of this population, a simple random sample of 800 individuals were selected and addresses were confirmed. To administer the mailed survey, a modified Dillman et al. (2014) tailored design method was used where a pre-notice flyer was shared via social media and contacts in the study area in mid-April 2023. This was followed by a survey packet at the end of April 2023, followed by a reminder postcard in mid-May 2023, with replacement survey packets whenever necessary. In order to encourage participation and improve response rate, this study utilized gift card incentives for participants.
Theoretical framework
The survey design was informed by the Theory of Planned Behavior (TPB) developed in 1991 by Ajzen as a successor to the Theory of Reasoned Action (Fishbein and Ajzen, 1975). TPB has been widely applied to investigate complex relationships between intentions and behaviors/actions. According to TPB, the immediate precursor of action is the intention to act, and the strength of the intention determines the likeliness to perform a behavior, or the willingness to try and exert effort towards the task at hand (Ajzen, 1991; Suh and Hsieh, 2016).
This study incorporated moral norms to an extended TPB. A moral norm is something that mediates a person's attitude before it affects his intention. This approach was taken in line with Kaiser and Scheuthle (2003) findings where a moral norm was found to be a supplementary predictor of a person's intention to act in a conservational manner (after attitudes, subjective norms, and perceived behavioral control). Additionally, risk perception was also included since both environmental policy and the perceived acceptability of environmental policy are to a large extent based on the perceptions and judgments of risk of various groups of both experts and laypersons (Douglas and Wildavsky, 1982; Roe, 1996).
Survey questions/questionnaire design
To develop the survey, we created questions related to general farm information, basic farmer characteristics, irrigation and conservation practice, moral norms and risk perception. Farmers were asked about irrigation methods, timing, amounts and water sources, decisions on irrigation as well as their opinions on issues pertaining to groundwater availability and use and perceptions on changing climate. In addition, factors that could impact groundwater conservation (e.g., groundwater availability, behavioral norms, social norms, perceived behavioral control, risk perception, moral norms, etc.) were listed in Likert type survey questions with option to choose a level of agreement ranging between strongly agree and strongly disagree or extremely likely to extremely unlikely. Besides these questions, information on factors influencing decision-making was sought to assess the motivation and intention of farmers towards groundwater conservation. Questions including aforementioned factors and additional questions were mainly developed based on reviewed literature, discussions with agriculture extension staff and farmers. Overall, the survey contained 37 questions across 12 pages (Survey questionnaire available in supplementary information).
Limitations
The following limitations were identified for this study:
- Despite numerous efforts to encourage participation in the study, the survey garnered a low response rate. We tried to remedy this by having an additional round of surveys shared during a harvest day celebration in Fall 2024
- To obtain producers’ mailing addresses, we procured a list from a marketing database service. Therefore, the study is limited by the use of a convenience sample.
- The study relied on self-reported data, which may be influenced by individual response bias, participant understanding or interpretation of questions, and introspective ability (Hoskin, 2012).
Data Exploration with PCA (Principal Component Analysis) and Cluster Analysis (CA)
PCA is a dimensionality reduction technique that simplifies large datasets by identifying the most important variables (principal components). It's widely used for visualizing and understanding complex data. High-dimensional data, like survey data, can be challenging to interpret. PCA reduces this complexity, making it easier to spot patterns, clusters, or outliers.
In this project, PCA was utilized for preprocessing the data because it provides a clear visual representation of sample variability, helping identify batch effects or experimental differences. We paired PCA with the k-means clustering technique for deeper insights into our data's structure. We utilized Python's scikit-learn to make PCA implementation simple.
Factors that were constructed based on PCA results were rotated using the varimax method for easier interpretation. Only the factors exceeding an eigenvalue of one were retained for cluster analysis. Cluster analysis helped us to group farmers based on our data according to their (dis)similarity in terms of their attributes represented by selected variables. Cases within a certain cluster should be similar to each other, and cases belonging to different clusters should be dissimilar. The K-means clustering method was utilized in this study. The result was four clusters.
In total, 54 variables were included in the PCA, of which ten principal components with eigenvalues >1 were identified for further analysis. Then, 10 components explained >70 % of the total variability in the dataset were selected. Each component was evaluated to help develop descriptive labels that characterize them according to the variables with which they were most strongly associated.
The Soil and Water Assessment Tool (SWAT)
1. Sustainable cropping practices and their impacts on the water balance
Conservation practices have shown many soil and water conservation benefits, such as improving soil water retention and reducing runoff and erosion. Despite a plethora of studies on the conservation benefits of cover crops, it is still unclear whether a regional installation of cover crops is a viable option to counteract the negative impacts of climate change on agricultural water availability. Modeling the effects of the adoption of cover crops on water quantity provides opportunities for assessing the long-term impacts of this conservation practice on water resources. In this study, the use of an over the winter cover crop in Central Nebraska was evaluated using a Soil and Water Assessment Tool (SWAT) model modified using cereal rye cover crop and future climate data. The projected data from the Coupled Model Intercomparison Project Phase 5 (CMIP 5) of 5 General Circulation Models (GCMs) were used. Climate data for a 20-year period of middle (2030-2050) of the 21st century under two Representative Concentration Pathway (RCP) emission scenarios (RCP 4.5 and RCP 8.5) was utilized. Our goal was to assess the long-term effects of cover crops on soil-water dynamics and evapotranspiration in a semiarid agricultural region in Central Nebraska during the period from late fall to early spring. The study found that evapotranspiration (ET) and recharge increased by 2% and 26 % respectively. Furthermore, the study found that rye cover crops decreased annual surface runoff, soil moisture and shallow aquifer storage by 3 %, 3 %, and 6 % respectively. Under the climate change scenarios, results show increases in ET ranging from 1 % to 2 %. This increase in ET was accompanied by increases in surface runoff in all scenarios except 2 (Hot & Dry RCP 8.5 and Cool & Dry RCP 4.5). Groundwater recharge also increased by between 28% - 39% with accompanying reductions in soil moisture and shallow aquifer storage (1 % - 8 % and 7 % - 20 % respectively) across different climate scenarios. This research offers insights into the effects of cover crops on water balance and fluxes related to the impacts of climate change.
Table 1: Relative changes in average annual water balance components after the installation of
winter cover crop
Scenario |
RCP |
ET |
Soil Moisture |
Runoff |
Shallow Aquifer Storage |
Recharge |
Baseline |
NA |
2% |
-3% |
-3% |
-6% |
26% |
Hot & Wet |
4.5 |
2% |
-1% |
6% |
-20% |
38% |
8.5 |
2% |
1% |
3% |
-20% |
39% |
|
Cool & Wet |
4.5 |
2% |
-3% |
2% |
-18% |
31% |
8.5 |
2% |
-2% |
3% |
-20% |
32% |
|
Hot & Dry |
4.5 |
2% |
-3% |
3% |
-12% |
37% |
8.5 |
1% |
-8% |
-2% |
-7% |
28% |
|
Cool & Dry |
4.5 |
1% |
-5% |
-1% |
-11% |
30% |
8.5 |
1% |
-5% |
2% |
-8% |
30% |
|
Central Tendency |
4.5 |
2% |
-3% |
1% |
-13% |
30% |
8.5 |
2% |
-2% |
4% |
-14% |
35% |
The results concurred with studies that reported increases in ET with the installation of winter cover crops in the fields which would otherwise be bare (Qi et al., 2011; Nielsen et al., 2015; Basche et al., 2016b; Meyer et al., 2019). This is expected because cover crops enhanced transpiration through increased leaf cover areas (Nielsen et al., 2015b; Qi et al., 2011). Our results also indicate fluctuations between increase and decrease in runoff while groundwater recharge significantly increased under the cover crop scenarios. This outcome adds to previous studies, which found that cover crops impacts on water infiltration lead to alterations in runoff (Dabney 1998; Unger and Vigil 1998; Owuor et al., 2016; Singh et al., 2018). These studies suggest that, beyond affecting evapotranspiration (ET), cover crops improve infiltration, which can subsequently decrease runoff and soil erosion. However, in our study, cover crops led to increased runoff in some instances which could be attributed to soil saturation due to higher groundwater recharge (Stanton et al., 2011). While the percentage increase in runoff was not substantial, it came alongside an increase in recharge, which is beneficial for groundwater sustainability.
2. Agricultural water management (AWM) and how it affects the resilience of watersheds
Results show that winter cover crops significantly affected water dynamics and watershed resilience. The average annual resources of the watershed for the study period (1994 to 2014) were 911.5 mm with an annual average green water resource of 753.1 mm (474.2 mm flow and 278.9 mm storage). The blue water resource constituted 158.3 mm (104.5 mm flow and 53.8 mm storage). This indicates that the primary water resources utilized in the watershed are green water resources which were almost 5 times the amount of blue water resources.
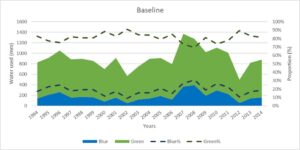
Green water was about five times blue water in the baseline. Following the incorporation of the winter cover crop, the average annual resources of the watershed for the study period (1994 to 2014) reduced to 901.5 mm with an annual average green water resource of 721.3 mm (486.5 mm flow and 234.8 mm storage). The blue water resource reduced to 180.2 mm (125.2 mm flow and 55.0 mm storage). Thus, in the study area the green water resources remained primary but reduced to about 4 times the amount of blue water resources. As seen in figure 2, blue water consumption was lower than that of green water, but the proportion decreased under the baseline WCC scenario.
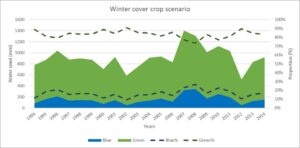
The inter-annual variability of blue and green water under the climate scenarios is shown in Table 2. Following the installation of cereal rye over the winter, the total green water resources consumed slightly decreased under all the climate change scenarios. The reduction of green water consumed is likely due to lower soil moisture (green water storage) which is taken up by the cover crop. The changes in green water in turn impact subsurface flows (lateral and groundwater flow) impacting the water yield and hence some reductions in blue water for all scenarios. The green, blue, and total water footprints showed decreases in all the climate change scenarios.
Table 2: The relative changes in the amount of blue and green water consumed and footprints after the winter cover crop installation (under the climate scenarios)
Scenario |
RCP |
WB |
WG |
BWF |
GWF |
WFTOTAL |
Hot & Wet |
4.5 |
-17% |
-4% |
-54% |
-52% |
-52% |
8.5 |
-17% |
-3% |
-45% |
-39% |
-40% |
|
Cool & Wet |
4.5 |
-19% |
-2% |
-48% |
-41% |
-41% |
8.5 |
-20% |
-2% |
-43% |
-31% |
-32% |
|
Hot & Dry |
4.5 |
-19% |
-10% |
-54% |
-51% |
-51% |
8.5 |
-20% |
-13% |
-43% |
-40% |
-40% |
|
Cool & Dry |
4.5 |
-24% |
-9% |
-50% |
-42% |
-42% |
8.5 |
-22% |
-11% |
-48% |
-42% |
-42% |
|
Central tendency |
4.5 |
-22% |
-7% |
-47% |
-40% |
-41% |
8.5 |
-19% |
-8% |
-47% |
-46% |
-46% |
Overall, the installation of the winter cover crop during the baseline period resulted in slight changes in the blue and green water availability (increases of approximately 4 % and 5 % respectively). The spatial variability of green and blue water scarcity was mapped out to determine the ecological hot spots (scarcity > 2). Green water scarcity is represented in Figure 3. High values of water scarcity are observed in the upstream sub-basins to the west and along the river in the mid and downstream sub-basins. Under the winter cover crop (WCC) scenario, the pattern of severe water scarcity persists as seen in the no winter cover crop (NWCC) scenario, with additional sub-basins also exhibiting high scarcity values.
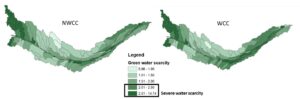
The spatial variability of blue water scarcity is represented in Figure 4. High values of scarcity occur to the west in the upstream sub-basins and two sub-basins close to the middle of the watershed. Under the WCC scenario, we still see severe water scarcity in the same pattern as NWCC with one downstream sub-basin showing slightly higher scarcity values
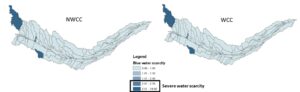
The results indicate that while cover crops primarily rely on green water, their use can lead to reduced groundwater recharge, thus impacting blue water availability. Our findings align with and expand upon previous research whereby changes in green water impact blue water and vice versa (Postel et al., 1996; Rockström et al., 1999; Hoekstra et al., 2011; Schyns et al., 2015) This reduction in blue water flow, particularly in downstream sub-basins, suggests that cover crops might compromise groundwater sustainability if not managed properly. However, by improving soil moisture retention and reducing runoff, cover crops can enhance the resilience of watersheds to drought conditions.
The study also used water scarcity metrics in addition to water footprint (WF) to offer a comprehensive understanding of the resilience of watersheds to AWM driven by human decision-making. Water scarcity assessments are useful in determining the degree to which freshwater use approaches or exceeds limits to freshwater availability in the face of land use changes. This study aimed to add to other studies that have addressed blue water scarcity (Vörösmarty et al., 2000; Vörösmarty et al. 2010; Kummu et al., 2016; Mesfin et al., 2016). In addition, our study also addressed the gap left by most studies where green water scarcity is rarely considered. The results showed the importance of the green water resources in supporting the system, specifically, crop growth within the study area. The study results concur with previous research and demonstrates the importance of green water in similar landscapes underscoring the need for its assessment (Alcamo et al., 2003; Arnell et al., 2004; Rost et al., 2008). Our study showed some spatial variability in the occurrence of green water scarcity. The availability of green water for crops depends on precipitation levels, losses to runoff and evaporation, farming practices, soil types, land topography, and is further influenced by increasing water demands and climate change. The different sub-basins in the study area had such differences and hence the different values of water scarcity. The areas with high values of water scarcity were considered as hotspots. This is because the increasing green water scarcity means that reduced green water flows remain for the agricultural system and for fulfilling additional demands stemming from changes in the system (Postel et al., 1996; Rockström et al., 1999; Hoekstra et al., 2011; Schyns et al., 2015).
Groundwater Model - Cooperative Hydrology Study (COHYST)
The integration of the COHYST MODFLOW model into FloPy proved successful, enabling seamless interaction with the model's complex Fortran-based input and output files. The modified FloPy scripts effectively handled the COHYST model's structured data requirements, ensuring accurate interpretation of input parameters and output results.
Key outcomes included the successful resetting of format descriptors, which resolved initial issues related to data alignment and truncation. By standardizing the input/output handling processes, the FloPy implementation replicated the COHYST model's original behavior, producing consistent outputs such as groundwater heads, flow patterns, and water budgets. Debugging and validation steps confirmed that FloPy-generated results matched the original MODFLOW outputs, demonstrating the accuracy of the approach.
The visualization tools in FloPy provided clear insights into groundwater dynamics, with contour maps and flow vectors illustrating recharge and discharge zones, as well as the effects of pumping wells. Additionally, the automation capabilities of FloPy allowed for efficient scenario testing, enabling the analysis of different hydrological conditions and management strategies. This integration highlights FloPy's utility in enhancing the functionality and usability of large-scale groundwater models like COHYST, making them more accessible for advanced analysis and decision-making.
Social System Model - Agent-based Modeling in Mesa
In total, 54 variables were included in the PCA, of which ten principal components with eigenvalues >1 were identified for further analysis. Then, 10 components explained >70 % of the total variability in the dataset were selected. Each component was evaluated to help develop descriptive labels that characterize them according to the variables with which they were most strongly associated.
PC1 explained approximately 20% of the total variance. PC1 to PC3 together explain around 50% of the variance. PC1 to PC5 explains a little over 60% of the variance. PC1 to PC7 explain close to 70% of the variance. In this case we aimed to retain enough PCs to explain a significant portion of the variance (e.g., 70% to 90%). We wanted to explain at least 70% of the variance, thus we included 10 components.
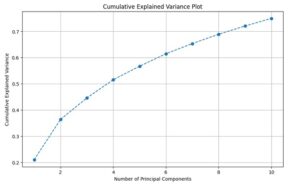

Cluster analysis: identifying and characterizing farmer types
The nine factors retained from PCA were analyzed using K-Means clustering methods, and further reduced to four clusters. The clusters were labeled according to their defining attributes: Risk Averse, Socially Influenced, Conditional, and Traditional. A radar chart representing four distinct farmer groups: Risk Averse, Socially Influenced, Conditional, and Traditional, categorized based on their responses to key psychological and behavioral factors is shown in figure 7. Each radar chart maps variables such as Attitude, Social Norms, Perceived Behavioral Control (PBC), Moral Norms, Perceived Risk, Trust, and Climate Perception.
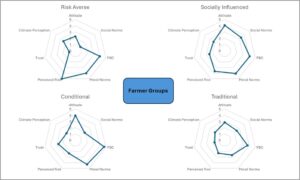
The following sections describe each cluster and discuss the rationale for the labels we assigned them.
Risk Averse: High on PBC (Perceived Behavioral Control) and moderate on other variables. Likely represents Risk-Averse Adopters, who feel confident in their control but are cautious.
Socially Influenced: High on Social Norms and PBC, low on Perceived Risk. This cluster might be best labeled as Socially Influenced Adopters, as they are influenced by social norms and feel confident in their actions.
Conditional: High scores across most variables, especially Attitude, Climate Perception, and Moral Norms. This is characteristic of Innovative Adopters, who are proactive and have a strong positive attitude.
Traditional: Generally lower scores across all variables. This cluster likely represents Traditionalists or Reluctant Adopters, who are less influenced by norms and have lower behavioral control.
The use of Principal Component Analysis (PCA) and cluster analysis to inform the creation of the agent-based model described above resulted in a structured and data-driven categorization of farmers based on shared characteristics and behaviors. PCA was employed to reduce the dimensionality of the dataset, retaining the most significant variables (e.g., age, income, water source, irrigation method) while minimizing redundancy. This allowed for clearer differentiation between farmers based on patterns in their responses. Following PCA, cluster analysis grouped farmers into distinct behavioral archetypes—Innovative, Risk-Averse, Socially Influenced, and Traditional—based on their irrigation decisions, water use practices, and future plans. These clusters encapsulate the diversity in farmer decision-making strategies and attitudes toward resource management.
The clustering outcomes informed the behavior rules implemented in the FarmerAgent class. Each cluster type was associated with unique decision-making patterns that influence irrigation decisions. For instance, Innovative adopters tend to make higher irrigation investments, particularly when groundwater or mixed water sources are available, while Risk-Averse farmers adopt more conservative irrigation strategies. Socially Influenced farmers balance their decisions based on social norms and water sources, whereas Traditional farmers adhere to minimal irrigation practices. This data-driven approach enhances the realism of the model by ensuring that the agents' behaviors are grounded in observed patterns, providing insights into how different farmer groups respond to water scarcity and other challenges in agriculture. This enables the simulation to explore resource management scenarios and the potential impacts of targeted interventions.
Socio-hydrologic Modeling
Our project employs a socio-hydrological modeling framework that utilizes a loosely coupled setup to integrate human decision-making processes with hydrological dynamics. This approach allows the agent-based model (ABM) to simulate the behaviors and decisions of stakeholders, i.e., farmers, while the hydrological processes are captured separately through MODFLOW. The two models operate independently but exchange information at predefined intervals, enabling the ABM to update its agents’ decisions based on groundwater conditions and MODFLOW to incorporate water use or recharge rates derived from the ABM. This loosely coupled design maintains flexibility and modularity, facilitating iterative improvements to both the social and hydrological components without requiring full integration at each computational step.
Outputs from the ABM can be utilized to manually modify MODFLOW simulations and vice versa. The two models (ABM and MODFLOW) operate independently, and the data exchange occurs manually at predefined intervals or after completing a set of simulations. The key characteristic of a loosely coupled system is that our models are not directly integrated or automated in real-time; instead, they rely on manual adjustments for information transfer.
The socio-hydrological modeling framework employed in this study utilizes a loosely coupled setup, characterized by independent operation of the ABM and MODFLOW models. Each model is run separately, with simulations completed independently before outputs are shared to inform the other. The interaction between the models occurs through intermediate data exchange, which is handled manually rather than automated. This setup does not feature real-time feedback, as the models do not interact during runtime, distinguishing it from tightly coupled systems where dynamic and automated data exchange occurs at every time step. The loosely coupled approach offers simplicity by avoiding the complexity of full integration and automation while providing flexibility to explore various coupling scenarios or modify the exchanged data without requiring reconfiguration of the framework.
All analysis and modeling codes are freely available on the project website with links to GitHub.
Educational & Outreach Activities
Participation Summary:
In December 2021, I gave a virtual presentation at the American Geophysical Union (AGU) Fall Meeting sharing the preliminary findings from my research. I developed and calibrated a Soil and Water Assessment Tool (SWAT) hydrological model to help improve the understanding of the long-term impacts of cover crops in combination with climate change on the agricultural water management in agricultural systems. A combination of cover crop practices and climate change scenarios was examined using a SWAT-based water footprint assessment. I mainly shared the preliminary results that showed the impact of installing cover crops on soil-water dynamics and evapotranspiration in a semiarid agricultural region in Central Nebraska.
In April 2022, I was invited to the Southern Illinois University Sustainability Celebration to speak about my research. I was able to talk to students and faculty about the importance of groundwater, the challenges facing groundwater sustainability, and the importance of tackling these challenges now. I also talked about the methods that I am using for my research, the expected results, and their importance in addressing the challenges facing groundwater.
In October 2022, I presented additional results of my study on the impact of installing cover crops on soil-water dynamics and evapotranspiration at the Association of American Geographers (AAG) West Lakes Division Annual Meeting. The presentation garnered a lot of interest due to the reported impacts of the cover crop not only on soil water dynamics and evapotranspiration but also their impacts on climate change impact mitigation.
I shared my research at the 2024 North Central Agriculture and Climate Conference in Peoria, Illinois. The event was organized by the North Central Climate Collaborative (NC3), the USDA Midwest Climate Hub, Extension Foundation, and partners. At this event I shared my research with extension and agricultural professionals from across the region.
I shared my research at the 2024 American Water Resources Association, the Universities Council on Water Resources, and the National Institutes for Water Resources (AWRA, UCOWR, NIWR) Joint Water Resources Conference in St Louis, Missouri
Currently, we have finalized the Groundwater Sustainability (linked here) website which will serve as an educational and outreach platform designed to promote awareness and understanding of groundwater resources and their sustainable management. The site features accessible resources, including case studies, research findings, and practical tools, to support community engagement and informed decision-making. Outreach efforts highlighted include documents and videos (continually added), published journal articles addressing critical groundwater issues (as they come out), and announcements of upcoming presentations at academic and professional conferences or updates. The website also fosters collaboration by sharing ongoing projects and inviting stakeholders to participate in discussions about innovative solutions for groundwater sustainability challenges. This resource underscores a commitment to bridging science, policy, and community needs in addressing groundwater resilience and climate change adaptation.
To support education and outreach efforts in the field of groundwater modeling, a publicly accessible GitHub repository has been created to share Python codes and workflows for Agent-Based Models (ABM) with MODFLOW simulations. The repository provides a centralized platform for researchers, educators, and students to access freely available resources, including tutorials, example models, and scripts for simulating the interaction of farmer decision-making (ABM) with groundwater flow dynamics (MODFLOW). The repository is designed to promote open collaboration and learning, offering step-by-step documentation, visualization tools, and practical examples to bridge the gap between human decision-making processes and groundwater sustainability modeling.
This initiative complements ongoing outreach efforts, such as conference presentations, journal articles, and educational workshops, by ensuring that these resources remain available to a broader audience. The repository also serves as a foundation for future enhancements, inviting contributions and feedback from the community to advance the use of ABM and MODFLOW in addressing real-world groundwater management challenges. Regular updates will keep users informed of new additions, fostering a growing community of practitioners and learners in this interdisciplinary field.
Project Outcomes
This project aims to increase awareness of risks to groundwater sustainability imposed by unrestrictive pumping activities and future climate change. Using a range of project dissemination avenues (e.g., presentations, flyers, farming group emails, and social media), we expect to change the perception and attitudes of producers towards groundwater resources: from an unlimited reservoir to a limited and vulnerable resource that requires proactive protection. Second, we expect the state and regional natural resources agencies to use the modeling framework as a tool to inform water management options and intervention strategies to mitigate groundwater depletion.
Specific examples are our two research papers under preparation for publication which examines a common option to support sustainable agriculture. Sustainable cropping practices have emerged as a beneficial alternative to conventional cropping measures with growing popularity among farmers, researchers, and government agencies (Islam et al., 2006). Focus has been placed on those climate-resilience practices that mitigate the risks from excess rainfall and drought events (Stewart and Peterson, 2015). Cover crops have been recommended to help mitigate climate change impacts due to their ability to increase water infiltration while reducing runoff and soil evaporation. (Unger and Vigil, 1998; Qi et al. 2011a; Nielsen et al. 2015; Eshel et al. 2015; Yu et al. 2016). Additionally, cover crops have been found to positively impact physical and chemical soil properties, thus improving soil productivity and soil water storage capacity (Kay, 1998; Basche et al., 2016a). They also have numerous positive environmental impacts such as weed suppression and the sequestration of nutrients (Unger and Vigil, 1998; Bergtold et al., 2012; Kladivko et al., 2014). As a result, cover crops have increasingly been recommended in annual cropping systems (e.g., maize and soybeans) (Basche et al., 2016a; Kaspar and Singer, 2011). However, despite many studies agreeing on the effects of cover crops on the water balance components, there is still a lack of consensus on the magnitude of these effects. In response to this we utilized the SWAT model to address some gaps in knowledge: How cover crops as a sustainable cropping practice impacts water balance and how cover crops as part of agricultural water management impact watersheds' resilience.
SWAT Modeling outcomes
The adoption of cereal rye as a winter cover crop in Central Nebraska demonstrated significant impacts on the water balance, with a 2% increase in evapotranspiration (ET) and a 26% increase in recharge, while surface runoff, soil moisture, and shallow aquifer storage decreased by 3%, 3%, and 6%, respectively. Under climate change scenarios, recharge increased by 28%–39%, and ET rose by 1%–2%, indicating the potential of cover crops to enhance groundwater sustainability. Variability in water balance components across scenarios highlighted increases in recharge and fluctuations in runoff, while improvements in infiltration and reductions in soil erosion and water scarcity patterns underscored the long-term benefits of cover crops for watershed management.
Winter cover crops predominantly relied on green water resources, which were five times greater than blue water under baseline conditions. Their incorporation reduced green water consumption while increasing blue water availability by 4%–5%, contributing to enhanced drought resilience. Spatial analyses identified ecological hotspots of water scarcity, emphasizing the need for targeted management interventions. Additionally, both green and blue water footprints decreased across climate scenarios by 40%–52%, highlighting the critical role of balanced water resource management in supporting agricultural productivity and ecological sustainability.
Additionally, we developed some tools to support sustainability initiatives:
Integration of Groundwater Modeling with MODFLOW
The successful integration of the COHYST MODFLOW model into FloPy enabled efficient scenario testing and enhanced visualization of groundwater dynamics, including recharge, discharge patterns, and water budgets, validating FloPy as an accurate tool for large-scale groundwater modeling. Additionally, FloPy's automated workflows streamlined scenario analysis, improving the accessibility and usability of complex groundwater models for decision-making and resource management.
Agent-Based Modeling of Farmer Decision-Making
Farmers were categorized into four behavioral clusters—Risk Averse, Socially Influenced, Conditional, and Traditional—based on factors like perceived behavioral control, moral norms, and climate perception. These clusters informed the development of decision-making rules in the FarmerAgent class, enabling simulations of diverse responses to water scarcity. Additionally, a loosely coupled socio-hydrological modeling framework integrated agent-based modeling (ABM) with MODFLOW, facilitating iterative analyses of farmer decisions and groundwater dynamics and providing valuable insights into resource management strategies across various scenarios.
Educational and Outreach Initiatives
- Groundwater Sustainability Website:
- A dedicated platform was launched to disseminate research findings, case studies, and practical tools for groundwater management.
- Outreach materials, including videos, journal articles, and announcements, foster community engagement and collaboration.
- Open-Source Resources:
- A GitHub repository provides Python scripts and tutorials for simulating agent-based and MODFLOW interactions, supporting interdisciplinary learning and innovation in groundwater sustainability.
These outcomes highlight the project's contributions to understanding the impacts of sustainable cropping practices, advancing groundwater modeling techniques, and promoting community-centered approaches to water resource management.
During this project, both my advisor and I significantly deepened our knowledge and awareness of the nuanced impacts of sustainable agriculture, particularly through the use of winter cover crops like cereal rye. We developed a stronger appreciation for the complexity of water balance dynamics, gaining insights into how cover crops can enhance groundwater sustainability by increasing recharge and evapotranspiration while simultaneously reducing surface runoff, soil moisture, and shallow aquifer storage. Our understanding of agricultural water management expanded as we observed the critical balance between green and blue water resources, noting how cover crops could improve drought resilience and reduce water footprints across various climate scenarios. The identification of ecological hotspots of water scarcity emphasized the importance of spatially targeted interventions, broadening our perspective on the strategic planning required for sustainable watershed management. These findings have shaped our attitudes towards the role of conservation practices in building resilient agroecosystems and reinforced the importance of integrating scientific research with practical, data-driven solutions to address agricultural and ecological sustainability challenges.