Final report for GNC22-359
Project Information
Cranberry false blossom disease (CFBD) devastated the U.S. commercial cranberry industry in the 1920’s. The causal agent, cranberry false blossom phytoplasma, is vectored by the blunt-nosed leafhopper (BNLH; Limotettix vaccinii). Through combined efforts of cultivar breeding and chemical controls, CFBD and its vector were seemingly eliminated in commercial cranberry production nearly 100 years ago. In recent years however, localized occurrences of CFBD have been noted, as well as outbreaks of the BNLH vector in North America. The objective of this study is to survey the distribution and diversity of CFBD and leafhoppers in Wisconsin cranberry marshes. Field surveying for CFBD was done by visually identifying uprights displaying characteristic symptoms, including witches’ broom and floral abnormalities, which were collected from farms throughout central Wisconsin in 2022 and 2023. Uprights were dissected by plant organ type for DNA extraction, qPCR analysis, and confirmation sequencing. In symptomatic uprights, all plant organs, from roots to flowers and terminal buds, yielded positive results for the CFBD phytoplasma presence, suggesting a systemic infection. To measure the occurrence of BNLH, weekly collections were performed via sweep net over 20m transects along the bed edges and center lines from May through August, 2021-2023. The BNLH comprised 80% of the adult leafhopper population throughout the entire sampling period, with a nymphal stage lasting until late June and an adult stage ending in August. Additionally, a controlled cage feeding assay was performed to determine the degree of feeding injury done to cranberry uprights by BNLH, both the nymph and adult life stage, feeding at different densities for two weeks. We found that the amount of feeding injury was dependent on the density of BNLH at 0, 1, and 2 weeks post feeding for nymphs, but only at 0 weeks for adults. The findings of this study contribute to the understanding of this re-emerging pathogen and its vector, informing the industry of mitigation strategies for phytoplasma transmission by highlighting key phenological patterns of the vector species. Knowing that infection is systemic also suggests that removal of infected uprights requires total excision of root tissues, a rigorous and costly practice if infection is high, and favors control of the insect vector.
The expected learning outcomes for this project are: 1) researchers and cranberry growers will learn the extent of systemic infection of the CFBD phytoplasma throughout cranberry organ tissues; 2) researchers and cranberry growers will learn the seasonal phenology of the BNLH vector; 3) researchers and cranberry growers will learn when feeding is occurring on cranberries by BNLH; 4) researchers and cranberry growers will learn the difference in feeding injury based on BNLH abundance. The expected action outcomes of this project can be split into the short and long term, with short term outcomes being: 1) cranberry growers will learn how to identify the symptoms of CFBD, as well as its insect vector; 2) cranberry growers will learn the level of threat of BNLH feeding injury on yield. Long term outcomes are: 1) growers will know when CFBD is coming out of dormancy and adjust cultural controls for CFBD infection removal; 2) cranberry growers will refine chemical sprays to target BNLH populations at key life stage, based on the BNLH phenology, for optimal CFBD transmission prevention and preventing feeding injury from reaching damaging levels.
Research
Objective 1: Determine degree of infection of CFBD phytoplasma in cranberry
To assess cranberry vines (cv. ‘Ben Lear’) for phytoplasma presence, vines were collected from a single 1 ha bed in Cranmoor, Wisconsin. Whole vines (above- and below-ground organs) were collected by hand on a monthly basis from May to August in 2022-2023, and weekly during bloom (mid-June to mid-July) when symptoms of the disease become most apparent due to distinct floral abnormalities (Figure 1) (Dobroscky 1929). Post harvest collections were done in September, October, and November. Each collection event involved walking the bed in its entirety with five false blossom-symptomatic uprights and five asymptomatic uprights being harvested. When symptoms were identified, the symptomatic upright and an asymptomatic upright were collected in pairs within one meter of each other, and extracted from the soil by hand with roots intact. During the 2023 field season additional sets of five symptomatic uprights were taken on the same schedule from a cluster of symptomatic uprights that exhibited a pattern of growth that suggests infected uprights may have been planted, compared to a pattern of infection typically associated with insect vector transmission. Additionally, another set of five symptomatic uprights were routinely taken from an adjacent bed in which symptomatic uprights were found. Uprights were stored overnight in a 4°C refrigerator, photographed with a digital camera (Nikon, Tokyo, Japan) using a Canon EFM 28mm f/3.5 Macro IS STM lens (Tokyo, Japan) and annotated for symptom progression throughout the growing season.

Phytoplasma DNA from V. macrocarpon was extracted from individual plant organs (root, stem, old leaf, new growth leaf, pedicel, flower/fruit) utilizing an adaptation of cetyltrimethylammonium bromide (CTAB) methods used in both Mujadid (2008) and Clements et al (2021). Plant tissues were excised from the plant utilizing a sterile scalpel, flash frozen in liquid nitrogen, and lysed with eight lysing matrix z beads (MP Biomedicals, Irvine, CA, USA) utilizing a PowerLyzer 24 Homogenizer (Qiagen, Hilden, Germany) for three, 45 sec cycles at 3200 rpm, with 10 sec breaks between cycles. Lysed samples were eluted with 500 μL of CTAB solution and re-homogenized following the same program cycle. Samples were then incubated in a 55°C digital heat block (Biodot, Irvine, CA, USA) for one hour, with the addition of 1.5 μL of RNAse A (QIAGEN, Hilden, Germany) halfway through incubation. A 500 μL volume of chloroform was added to samples, which were then mixed via an inversion table (Labnet International, Woodbridge, NJ, USA) for 10 min. In 2022, chloroform:isoamyl alcohol (24:1). Sample tubes were centrifuged (Eppendorf, Hamburg, Germany) at 16 rcf for 10 min before 350 μL of the supernatant was transferred to a fresh microcentrifuge tube for precipitation with ice-cold 28 μL of ammonium acetate and 204 μL of isopropanol, followed by overnight storage at 4°C. Samples were centrifuged at 16 rcf for 15 mins at 4°C for pelletization of DNA before liquid as decanted. Two ethanol washes of the DNA, with 75% and then 95% ethanol, with 10 min centrifugation at 16 rcf for each wash. Then, DNA samples were left to air dry overnight, rehydrated with 50 μL of double distilled water, and re-suspended overnight before storage at -20°C.
All plant DNA extractions from 2022 and 2023 underwent quantitative PCR (qPCR) testing. Primer and probe ratios were used as per manufacturer’s instructions of igScript Probe-Based qPCR 2X Master Mix (Intact Genomics Inc., St. Louis, MO, USA). Primers meant to amplify phytoplasmas but not errant bacteria were selected, following the methods of Christensen et al. (2004) with forward (5’CGTACGCAAGTATGAAACTTAAAGGA3’), reverse (5’TCTTCGAATTAAACAACATGATCCA3’) and probe (5’TGACGGGACTCCGCACAAGCG3’) labeled with a FAM (6-carboxyfluorescein) fluorophore. The qPCR began with an initial 3 min at 95℃, followed by 40 cycles of 95℃, denaturation for 5 sec and 60℃ annealing and elongation for 30 sec, before ending with a stasis of 4℃, and was performed in a CFX96 Real-Time System PCR Detection System (Bio-Rad Laboratories, Hercules, CA, USA). To validate positive qPCR outputs, a subsample of positive returns underwent PCR using the P1A/16S-SR primer pair (5’AACGCTGGCGGCGCGCCTAATAC3’/ 5’GGTCTGTCAAAACTGAAGATG3’) using a T100 Thermal Cycler (Bio-Rad Laboratories, Hercules, CA, USA) (Lee et al. 2004). PCR products were electrophoresed through a 1.5% agarose gel and visualized with a GelDoc Go Imaging System (BIORAD, Oakland, CA, USA) before being sent to Functional Biosciences, Madison, WI, USA for cleanup and sequencing using BigDye v3.1 (Thermo Fisher Scientific, Waltham, MA, USA) on ABI 3730xl instruments (Applied Biosystems, Waltham, MA, USA). Sequence data underwent the same quality checking, trimming and querying as previously described.
Objective 2: Assess the seasonal phenology of L. vaccinii (BNLH)
To assess the seasonal phenology of L. vaccinii, we sampled leafhoppers at four to six commercial cranberry farms in 2021-2023 in Central Wisconsin, between the regions of Nekoosa, WI and Black River Falls, WI. Field sites were selected based on previous season reports of L. vaccinii presence from grower operators, industry integrated pest management scouts, and observations at the start of each growing season. Sampling was done weekly at each site and if a site elected to apply a chemical insecticide listed for control of leafhoppers, collections continued for two weeks to confirm the applications efficacy and then ceased. We sampled for leafhoppers at six total farms in 2021, from May 17 until August 16. Sampling began at three sites, all of which chose to spray the week of May 24, 2021. New sites hosting L. vaccinii were added for sampling, one site each for the weeks of June 14, June 21, and July 5, 2021. We sampled for leafhoppers at five total farms in 2022, from May 16 until August 29. Sampling began at three sites, two of which chose to spray the week of May 30 and June 6, 2022. New sites hosting L. vaccinii were added for sampling, both the week of June 13, 2022. We sampled for leafhoppers at four total farms in 2023, from May 15 until August 21. Sampling began at two sites, with the other two being added May 29, 2023. One site did perform chemical sprays on three of the beds we were sampling from on June 12, 2023. The number of L. vaccinii was sampled twice in each bed, using a sweep net along a 20 sweep transect line covering approximately 20m. Fixed sprinkler heads were used as benchmark locations to start sweep sets and starting sprinkler head locations were determined each week via a random number generator. Insects collected were transferred to individually marked paper bags, transported in a chilled cooler to the laboratory, and stored in a -20°C freezer. Specimens were observed in the laboratory under a dissecting microscope for separation by morphotype and life stage (immatures vs. adults) and counted.
Objective 3: Evaluation of feeding injury by L. vaccinii (BNLH) at different densities and life stages
To assess the impact of L. vaccinii injury of cranberry foliage, two experiments were conducted in enclosures harboring different densities of L. vaccinii, at two life stages: nymph and adult. The bioassays, based on McMahan and Guedot, 2018, used a hydroponic system and insect enclosures consisting of individual 221.8 mL, semi-translucent, plastic vials (Squeezetops Pharmacy Vials, United States Plastic Corp., Lima, OH, USA) with removed bases. Vial caps were hollowed and capped with a mesh screen (GreenTek, Janesville, WI, USA), wedged into cutout recesses in a sheet of polystyrene foam. Cranberry uprights were obtained from a farm that received no chemical applications in the previous 14 days, no previous insecticide treatments targeting L. vaccinii, and no confirmed reports of L. vaccinii in the previous growing season. Healthy cranberry uprights collected from the field were stored at 4°C overnight, washed, and immersed in water for 24-hours, to mimic a common submergence practice in the cranberry industry for insect control to ensure insect-free clean uprights (van Zoeren et al. 2017). Uprights were then visually inspected for injury and only asymptomatic uprights were selected and grouped based on similar levels of foliage density across all treatments. The roots of the uprights were passed through a hole cut into a layer of polystyrene into a tray housing water which was aerated with a Tetra Whisper 10-30 air pump for longevity (Tetra, Spectrum Brands LLC, Blacksburg, VA). The hole was plugged with cotton balls to prevent insect escape. Clusters of 2-4 uprights possessing new and old foliage were placed in enclosures. In each enclosure either 0, 5, 10, or 20 L. vaccinii individuals were introduced, with 10 replicates for each treatment and placed in a greenhouse maintained at 21℃ during the day and 17℃ at night, with a 16:8 (L:D) photoperiod with the light period from 5AM to 9PM. Prior to introducing the L. vaccinii to the uprights, a one-week observation period was conducted to ensure survival of the uprights and total number of leaves and uprights per enclosure was noted. Throughout the study, plant trays were filled weekly with water to ensure root immersion.
All L. vaccinii were collected from a commercial cranberry farm that had not sprayed any insecticide at least two weeks prior, on June 17, 2022 for nymphs and on August 1, 2021 for adults and transported in paper bags in a chilled cooler. Upon return to the laboratory, individuals were briefly anesthetized via CO2 gas and individually transferred into enclosures. Leafhoppers were left in the enclosures for two weeks after which they were removed and counted. During the two weeks of insect exposure, observations were performed every 2-3 days to record insect mortality. An individual was considered dead if it was found at the bottom of the enclosure and non-responsive for more than 20 sec. During the two weeks following insect removal, uprights were removed from feeding enclosures and maintained in aerated plant trays and observed for the development of feeding injury, characterized by foliar deformation and/or localized necrosis (Image 2).
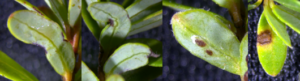
All statistical analyses were performed utilizing Python 3.10 (Python Software Foundation, Wilmington, DE), utilizing the following packages: pandas (ver. 2.2.2, Feb. 2020, pandas Development Team, doi: 10.5281/zenodo.3509134; McKinney et al. 2010), numpy (ver 1.25.1, NumPy team 2024; Harris et al. 2020), matplotlib (ver 3.9.0; Hunter 2007), math and statistics (Python Software Foundation, Wilmington, DE; Van Rossum 2020). An one-way ANOVA was performed to test for differences in the average number of nymph (2022 and 2023) and adult (2021-2023) L. vaccinii present at the center and edge of cranberry beds each year. Statistics were not run on nymphs from the 2021 growing season due to missing data on account of grower chemical applications. These weeks were chosen as representative data with respect to the lifecycle of L. vaccinii, and to limit autocorrelation within the data. An ANOVA was used to measure the effect of leafhopper density on the percent of leaves injured for each feeding period and each life stage. Replicates that exhibited cranberry upright mortality were excluded from statistical analyses. A Bonferroni correction was used to calculate a new critical value for each set of tests for a Tukey HSD Pairwise comparison.
References:
Dobroseky, I. D. Cranberry False-Blossom Disease Spread by a Leafhopper.Science 70, 635-635 (1929). DOI:10.1126/science.70.1826.635.a
Clements, J., Bradford, B., Garcia, M., Piper, S., Huang, W., Zwolinska, A., Lamour, K., Hogenhout, S., Groves, R. ““Candidatus Phytoplasma Asteris” Subgroups Display Distinct Disease Progression Dynamics during the Carrot Growing Season.” PloS One, vol. 16, no. 2, 4 Feb. 2021, pp. e0239956–e0239956, https://doi.org/10.1371/journal.pone.0239956.
Christensen, Nynne Meyn, et al. “Distribution of phytoplasmas in infected plants as revealed by real-time PCR and bioimaging.” Molecular Plant-Microbe Interactions®, vol. 17, no. 11, Nov. 2004, pp. 1175–1184, https://doi.org/10.1094/mpmi.2004.17.11.1175.
Harris, C. R., Millman, K. J., van der Walt, S. J., Gommers, R., Virtanen, P., Cournapeau, D., Oliphant, T. E. (2020). Array programming with NumPy. Nature, 585, 357–362. https://doi.org/10.1038/s41586-020-2649-2
Hunter, J. D. (2007). Matplotlib: A 2D graphics environment. Computing in Science & Engineering, 9(3), 90–95.
Lee, I.-M., Polashock, J., Bottner-Parker, K.D., Bagadia, P.G., Rodriguez-Saona, C., Zhao, Y., Davis, R.E. “New Subgroup 16SrIII-Y Phytoplasmas Associated with False-Blossom Diseased Cranberry (Vaccinium Macrocarpon) Plants and with Known and Potential Insect Vectors in New Jersey.” European Journal of Plant Pathology, vol. 139, no. 2, 30 Mar. 2014, pp. 399–406, https://doi.org/10.1007/s10658-014-0396-7.
McKinney, W., & others. (2010). Data structures for statistical computing in python. In Proceedings of the 9th Python in Science Conference (Vol. 445, pp. 51–56).
Mujadid A. CTAB DNA Extraction Protocol This protocol was used in the Kalisz Lab for the Outcrossing Rates/Microsat project from 2006 on. Adapted for the Kalisz lab from Soltis lab protocol. Academia.edu. 2008. https://www.academia.edu/4761019/CTAB_DNA_Extraction_Protocol_This_protocol_was_used_in_the_Kalisz_Lab_for_the_Outcrossing_Rates_Microsat_project_from_2006_on_Adapted_for_the_Kalisz_lab_from_Soltis_lab_protocol
Van Rossum, G. (2020). The Python Library Reference, release 3.8.2. Python Software Foundation.
van Zoeren, J., Guedot, C., Steffan, S.A. “Conserving Carnivorous Arthropods: An Example from Early-Season Cranberry (Ericaceae) Flooding.” The Canadian Entomologist, vol. 150, no. 2, 18 Jan. 2018, pp. 265–273, https://doi.org/10.4039/tce.2017.66.
Objective 1: Determine degree of infection of CFBD phytoplasma in cranberry
A total of 913 plants were collected and processed in the laboratory for phytoplasma DNA presence (Table 1). Symptomatic organ samples tested positive for phytoplasma in 83.8% of extractions across both years, while asymptomatic organ samples tested positive in 19.5% of extractions.
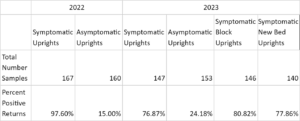
In 2022, DNA extractions from symptomatic plants indicated that over 90% of samples in each organ type category tested positive for the presence of cranberry false blossom phytoplasma (Table 2). Phytoplasma DNA in asymptomatic tissue extractions ranged from 6.06% detection in ‘old leaves’ to 50% amplification in ‘stem’ tissue and 62.86% amplification in ‘roots’.
Phytoplasma DNA recovered from symptomatic plants in 2023 saw a wider range of detection frequency, with less than 45.45% and 63.65% amplification in ‘root’ and ‘stem’ tissues, respectively, and greater than 90% amplification in ‘new leaf, ‘pedicle’, and ‘floral’ tissues (Table 2). Asymptomatic plants yielded 8.33% and 10.71% phytoplasma DNA amplification in respective ‘old leaf’ and ‘new leaf’ tissues, and 30-37% amplification in all other organ types. All seven positive controls in 2023 saw amplification, while all seven negative controls did not. Phytoplasma DNA was consistently recovered from plant tissues throughout the entirety of the 2022 and 2023 collections, represented by data for old leaf tissue and new leaf tissue in 2023 (Table 3). Symptomatic samples ranged from 66.67% (old leaves in June 2023) to 100% in number months for both organ types. Asymptomatic plant samples were fewer in number and did not amplify in most extractions, but did range up to 33.33% in new leaf samples in November 2023.
Despite amplification in qPCR, multiple samples failed to amplify in the nested PCR protocol. Numerous samples showed streaking in gel electrophoresis along the entire length of well columns. All samples that showed strong amplification bands, as well as a number of streaking bands, were submitted for Sanger sequencing, but only a small subset came back with high quality forward and reverse sequences. In successful sequencing for all 19 samples, (both the 1800bp P1/P7 PCR and 1500bp P1A/16S-SR PCR) nucleotide local alignment showed very strong match identity (>98%) in samples with registered cranberry false blossom sequences. All amplicons from PCR with P1A/16S-SR primer pair saw top matches with registered cranberry false blossom phytoplasma references, while P1/P7 amplicons saw higher identity matches with other phytoplasmas over cranberry false blossom phytoplasma. Amplicons from the 80bp UPPFw/UPPRv qPCR had strong identity match (82-88%) with registered cranberry false blossom phytoplasma references.
This study provides insight into the field detection of cranberry false blossom disease using a sensitive real time PCR protocol for expedient and sensitive testing of plant material. The findings of the spatial and temporal distribution of the CFBD phytoplasma within V. macrocarpon indicates that infection is detectable in all plant organs, during each month of the growing season.
Our results showed that the CFBD phytoplasma causes a systemic infection in all organs of V. macrocarpon, which is consistent with other phytoplasma infections; such as in lime (Ghosh et al. 1999), grape (Laimer and Bertaccini 2019), and citrus (Trivedi et al. 2010). Phytoplasma infections exhibit varying levels of plant host colonization, and therefore detection, in different plant organs (Christensen et al. 2004). While we were able to successfully extract CFBD phytoplasma DNA from each of our plant organs (stem, roots, old and new growth leaves, flowers, and fruit), extraction efficiency was lower in woody stem (63.63%) and root (45.45%) tissues. Reliable extraction of phytoplasma DNA from these tissues has been found to be lower due to lower overall abundance (Harrison et al. 2013) and frequently requires modified extraction methodology and/or harsher chemical reagents, such as phenol:chloroform:isoamyl alcohol (Green and Thompson 1999). Additionally, phytoplasmas are known to have extensive incubation periods within both plant and insect hosts (Hogenhout 2008). The latency and incubation periods of CFBD phytoplasma is poorly understood, with estimates ranging from 1 month to 1 year before symptoms appear following infection (Polashock et al. 2017). Severity of symptom expression has also been found to correlate with the extent of the infection (Christensen et al. 2004). Even more, the uneven and slow colonization of vines by the CFBD phytoplasma may account for the inconsistent detection of the phytoplasma in asymptomatic vines across organ types. These data showing a systemic infection suggest that the current control mechanisms of pruning or mowing infected vines (University of Maine Extension), are ineffective cultural control practices due to the extent of infection within vines requiring full excision. Additionally they present an improved, precise method for other researchers and diagnostic laboratories with increased sensitivity of detection and the potential for temporal-based inquiry.
Objective 2: Assess the seasonal phenology of L. vaccinii (BNLH)
Earliest incidence of leafhoppers in sweeps occurred in the third week of May each year and saw a sharp increase in populations for two to three weeks following egg hatch (Figure 3). Due to the difficulty of identification at the nymphal lifestage, collected nymph leafhoppers cannot be assumed to all be L. vaccinii. Despite this, it is assumed that the majority of these individuals are L. vaccinii based on the sheer numbers collected and the percentage of leafhopper species diversity that is attributed to the species. Nymph populations persisted through the second week of July in 2021 and 2023, and through the third week of July in 2022. First occurrence of adult L. vaccinii was in the third week of June in 2021, and the fourth week of June in 2022 and 2023. Adult populations steadily grew for a number of weeks (two weeks in 2021, four weeks in 2022, and three weeks in 2023) before declining. The last occurrence of L. vaccinii in sweep sets happened in the second, fourth and first week of August in 2021, 2022, and 2023 respectively.
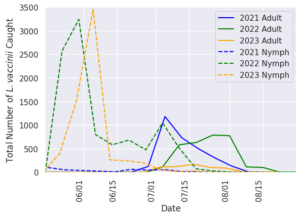
Leafhopper species identification for the nymphal lifestage was not possible due to the necessity of adult form sexual organs for positive identification. Limmotettix vaccinii was the most prevalent leafhopper species throughout the 2021 (80.87% of 3685 leafhoppers), 2022 (93.53% of 3325 leafhoppers), and 2023 (63.4% of 951 leafhoppers) growing seasons (Figure 4). Identification of the five next most prevalent genera throughout the same three years were Macrosteles quandrilineatus (Aster leafhopper; 64.54%, 43.26%, and 63.25%), Empoasca sp. (most likely Potato leafhopper; 17.73%, 35.35%, 5.15%), Athysanus argentarius (Silver leafhopper; 4.11%, 6.98%, 29.06%), Scaphytopius sp. (most likely Sharp-nosed leafhopper; 7.23%, 13.95%, 2.28%), and Gyponana sp. (most likely Red-eyed leafhopper; 6.38%, 0.47%, 0.28%).
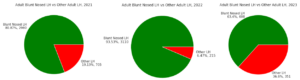
Our results show the seasonal phenology of the L. vaccinii vector in Wisconsin cranberry over three consecutive growing seasons and is similar to the phenology described in New Jersey (Rodriguez-Saona 2022). While we were not yet able to confirm that the nymphs collected were L. vaccinii , we strongly suspect that they were due to the sheer number of nymphs and adult L. vaccinii collected in the same season. Eggs hatch in mid-May, with a nymphal life stage lasting until the last week of June into the first week of July, and then adult populations persist into the middle of August. Climatic trends can almost assuredly be tied to shifts in these events from year to year as insect activity is tied to temperature. It is difficult to speak to the degree of infestation in a single growing season because commercial growers decided to utilize chemical insecticides for L. vaccinii control. These chemical applications can be seen in Figure 3 as drastic drops in the nymph populations, when growers are attempting to control the species before gaining the mobility and reproductivity of adulthood.
The discovery that the majority of leafhopper individuals in cranberry are L. vaccinii emboldens our claim that the majority of leafhopper nymphs in cranberry likely are L. vaccinii. Interestingly, other leafhopper species occurred in fewer numbers while L. vaccinii was present, with numbers rising as L. vaccinii died off. It could be that other leafhopper species hatch and molt later in the season, or the data could suggest that these other species are opportunistic transients that moved into beds as L. vaccinii numbers decline. Despite this, there may be reason to consider these other species as possible vectors of CFBD. Macrosteles quandrilineatus, the aster leafhopper, is a known vector of the aster yellows phytoplasma (Clements et al. 2021), a major disease of carrots. One species of the Gyponana genus, Gyponana hasta, is reported to vector the California aster yellows virus, now known to be a phytoplasma, which is detrimental to celery and sunflower. Record also shows that Scaphytopius acutus, the sharp-nosed leafhopper, has been found to be able to acquire the CFBD phytoplasma from cranberries in the states of Massachusetts and New Jersey (Stevens 1931). While the leafhoppers observed in Wisconsin cranberry could be a different species, it warrants further understanding if there may be more than one species capable of vectoring the CFBD phytoplasma.
Objective 3: Evaluation of feeding injury by L. vaccinii (BNLH) at different densities and life stages
Visible injury manifested in the form of leaf curl and/or localized leaf necrosis (Figure 2). Plant feeding injury was noted in all treatments, even in the control, at the time of the initial observation, when insects were removed from the enclosures. Some uprights began browning as early as this initial observation and this worsened during the duration of the observation period (Table 4). When testing if the number of plant injuries is dependent on leafhopper density in observations without upright mortality, an ANOVA was used and found dependence on nymph density for week 0 (F3,34 = 6.0528; P = 0.002), 1 (F3,34 = 23.880; P < 0.001), and 2 (F3,23 = 7.749; P< 0.001), as well as for adult density for observation 1 (F3,33 = 7.271; P < 0.001).
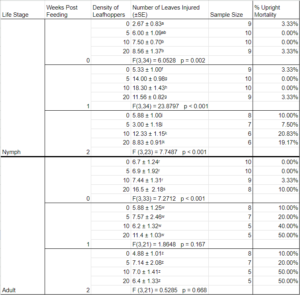
Results of the feeding assay show that the injuries that were categorized as leafhopper feeding injury occurred in the control as well as all three treatments in both the nymphal and adult studies. The injury observed on the control uprights could indicate that the uprights obtained from the field may have been fed upon by leafhoppers prior to the assay. However, the data suggests that the density of insects plays a role in feeding injury manifestation at all time points for nymphal feeding and at the initial observation in adult feeding. The increased amount of upright die-off in the adult assay may be attributed to study design and plants being at a phenological stage requiring higher nutrients than the hydroponic system offered. The presence of injury on leaves throughout all treatments and the control may suggest that BNLH feeding might not be directly responsible for the injuries, but rather exacerbate an existing injury. It is also known that other stressors, both biotic and abiotic, can cause similar types of injury in cranberries. Even in the presence of high densities, feeding injury by L. vaccinii has not been characterized (de Lang 2015, Guedot 2021, Sylvia and Averill 2023) but draining of vines at densities of BNLH of 100 to 200 per 25 sweeps has been reported (Armstrong). While there is no economic threshold for L. vaccinii, it is recommended to apply a broad-spectrum insecticide pre-bloom during the nymphal life-stage (Rodriguez-Saona 2019) primarily to control the spread of CFBD. Despite current management suggestions claiming that adults are responsible for CFBD transmission (Polashock et al. 2017), further consideration for the potential for transmission by nymphs should be considered.
References:
Armstrong, Charles. “Blunt-Nosed Leafhopper.” The University of Maine. https://extension.umaine.edu/cranberries/grower-services/insects/blunt-nosed-leafhopper/
Clements, J., Bradford, B., Garcia, M., Piper, S., Huang, W., Zwolinska, A., Lamour, K., Hogenhout, S., Groves, R. ““Candidatus Phytoplasma Asteris” Subgroups Display Distinct Disease Progression Dynamics during the Carrot Growing Season.” PloS One, vol. 16, no. 2, 4 Feb. 2021, pp. e0239956–e0239956, https://doi.org/10.1371/journal.pone.0239956.
Christensen, Nynne Meyn, et al. “Distribution of phytoplasmas in infected plants as revealed by real-time PCR and bioimaging.” Molecular Plant-Microbe Interactions®, vol. 17, no. 11, Nov. 2004, pp. 1175–1184, https://doi.org/10.1094/mpmi.2004.17.11.1175.
de Lange, E., and Rodriguez-Saona, C. “Blunt-Nosed Leafhopper: A Vector of Cranberry False Blossom Disease.” Rutgers University, Nov. 2015.
Ghosh, D. K., et al. “Association of a Phytoplasma with Witches’ Broom, a New Disease of Acid Lime (Citrus Aurantifolia).” Current Science, vol. 77, no. 1, 1999, pp. 174–77. JSTOR, http://www.jstor.org/stable/24102927. Accessed 20 June 2024.
Green, Margaret J., et al. “Easy and efficient DNA extraction from woody plants for the detection of phytoplasmas by polymerase chain reaction.” Plant Disease, vol. 83, no. 5, May 1999, pp. 482–485, https://doi.org/10.1094/pdis.1999.83.5.482.
Guedot, C. “Watching for Leafhoppers This Spring and Summer.” University of Wisconsin Madison, 27 Apr. 2021.
Harrison, Nigel A., et al. “DNA extraction from arborescent monocots and how to deal with other challenging hosts.” Methods in Molecular Biology, 19 Aug. 2012, pp. 147–158, https://doi.org/10.1007/978-1-62703-089-2_13.
Hogenout, S. A., Oshima, K., Ammar, E.-D., Kakizawa, S., Kingdom, H., Namba, S. “Phytoplasmas: Bacteria That Manipulate Plants and Insects.” Molecular Plant Pathology, vol. 9, no. 4, 28 June 2008, pp. 403–423, https://doi.org/10.1111/j.1364-3703.2008.00472.x.
Laimer, Margit, and Assunta Bertaccini. “Phytoplasma elimination from perennial horticultural crops.” Phytoplasmas: Plant Pathogenic Bacteria - II, 2019, pp. 185–206, https://doi.org/10.1007/978-981-13-2832-9_9.
Polashock, James J., et al., editors. Compendium of Blueberry, Cranberry, and Lingonberry Diseases and Pests. 2nd ed., APS Press, The American Phytopathological Society, 2017.
Rodriguez-Saona, C., Holdcraft, R., Kyryczenko-Roth, V. “Blunt-Nosed Leafhopper Control on Cranberries, 2018.” Arthropod Management Tests, vol. 44, no. 1, 1 Jan. 2019, https://doi.org/10.1093/amt/tsz009.
Rodriguez-Saona, C. “Recommendations for Blunt-Nosed Leafhopper Management in Cranberries.” Rutgers University, 28 May 2022.
Stevens, Neil. The Spread of Cranberry False Blossom in the United States. U.S. Department of Acriculture, Jan. 1931.
Sylvia, M., and Averill, A. “BLUNT-NOSED LEAFHOPPER in MA CRANBERRY.” University of Massachusetts Amherst., May 2023.
Trivedi P, Duan Y, Wang N (2010) Huanglongbing, a systemic disease, restructures the bacterial community associated with citrus roots. Applied and Environmental Microbiology 76, 3427–3436.
Educational & Outreach Activities
Participation Summary:
The Wisconsin cranberry industry is one that lends itself to education and outreach. The Wisconsin Cranberry Board has close ties with the University of Wisconsin (UW) Extension faculty and together strive to disseminate information to growers at multiple times throughout the year. There is a Cranberry School education program that is ran yearly in the spring to discuss prior growing season developments, at which our project data were presented. There is also a yearly Summer Field Day mini-clinic at which both Dr. Guedot and Dr. Holland interact with growers in a face-to-face demonstration of the continuing issues in cranberry, to include this research project's objectives.
The results of this research project will be submitted for publication(s) in scholarly journals, as well as exist on UW Extension archives for easy access by stakeholders. It is the intent of our program to create a useful field guide document of in field symptoms that growers and IPM field scouts can consult for accurate identification of CFBD.
Project Outcomes
Reviewing literature archives of the researchers from the 1930's displayed the generational gap in approaches to agricultural issues; where they had aggressive, broad-spectrum chemistries for insect control, modern agriculture employs targeted sprays for minimized ecological fallout. Our goals were truly to characterize the state of CFBD and its vector in Wisconsin, in ensure that management practices that were prescribed would be effective. Means of control of CFBD is quite limited, with no means of curing infected plants. Prior to our research it was assumed effective to either prune or burn away infected plant material, but we found that the below-ground tissues remain infected and grow back as a source of inoculum. For now CFBD remains fairly localized in Wisconsin cranberry, but growers that have the diseased plants need to weigh the costs of crop destruction and re-establishment. For many, the extent of infection would likely need to be severe before they would consider such an approach. In the meantime, our project outcomes provide windows of priority for monitoring fields for both symptoms and the vector.
Being that CFBD is a serious historical problem that predates the current farming generation, the chance to engage in exploratory science was extremely exciting for us. While there is working knowledge coming out of other growing regions, the alarm over the CFBD causal phytoplasma and its vector has been absent from Wisconsin cranberry for almost 100 years. The cranberry community is very generational, so most growers grow up working the marshes and learning the tricks of the trade. This, however, is was not the case with our project and came with a large amount of understandable ignorance, for growers and researchers, in things like identification. Learning how to discuss sustainable agricultural practices with growers that are understandably concerned and may stand to benefit from unsustainable practices was a real challenge. We learned along side the growers and aimed to present them with a dynamic range of information from base symptom identification to complex molecular analyses.
Further steps can be taken to expand upon our project on both the entomological and pathological fronts. Phenological characterization is a stepping stone for creating models of degree days for the insect vector, allowing for more robust modeling of year to year insect management. Further characterization of CFBD phytoplasma colonization within plants via quantification qPCR, as well as measurements of incubation periods before symptom expression occurs would go a long way towards aiding growers with this pathosystem.