Final report for GNE16-119
Project Information
Vegetative groundcover (also referred to as cover crop) may be planted in perennial fruit and nut systems for a variety of ecological or production goals, including growth reduction in humid climate vineyards. While growth reductions are presumed to be greater in young vines due to less developed root systems, studies have not examined if growth and mechanisms of competition depend on rootstock vigor. Over the 2017 season in a vineyard in Pennsylvania, we examined above- and belowground responses of vines on medium- or low-vigor rootstocks to groundcover (Festuca rubra) competition; assessed by shifts in aboveground biomass, root length, root morphological traits, as well as depletion of water and nutrients in vines and soil. In the presence of groundcover, vines on a medium-vigor rootstock had 40% lower vegetative growth and a 20% reduction in cluster weight. In contrast, vines on a low-vigor rootstock only had 21% lower vegetative growth but maintained similar cluster weights. The growing season was very wet and groundcover competition for nutrients, primarily nitrogen, was the apparent main cause of growth reduction as indicated by reductions in shoot nutrient content and concentrations and an overall decrease in soil nitrate content. Independent of rootstock, grapevine root systems coped with groundcover competition by redistributing roots from a shallow, highly competitive zone to a mid-depth less-competitive, but lower nutrient zone. There were also root morphological shifts; vines exposed to groundcover competition had a higher specific root length and decreased average root diameter. While the low-vigor rootstock had a larger absorptive root diameter, more shallow rooting, and differed in patterns of soil moisture availability in comparison to the medium-vigor rootstock, there was no clear link of resource uptake and growth differences between rootstocks. This study suggests that in a wet year, low-vigor rootstocks may be more tolerant of competition than medium-vigor rootstocks, but both may cope with groundcover competition by similar root responses.
In this study we examined the response of vigorous, genetically identical grapevines to a grass groundcover when vines were grafted onto low- or medium-vigor rootstocks. We hypothesized that in the first year of full grass groundcover, young vines grafted to a medium-vigor rootstock would be more tolerant of groundcover competition, leading to lesser reductions in nutrient and water uptake and aboveground growth as compared to those grafted to a low-vigor rootstock. We also hypothesized that aboveground observations would be supported by mechanisms associated with a plastic response: increased SRL, decreased root diameter, increased arbuscular mycorrhizal colonization and re-distribution of roots deeper, leading to a greater reliance on deep nutrients and soil water. The relatively more plastic response of the medium-vigor rootstock than the low-vigor rootstock to groundcover would explain differences in growth reductions between rootstocks.
Vegetative groundcover (also referred to as a cover crop, sensu ref) in perennial fruit and nut systems is often chosen for a variety of benefits including erosion mitigation, enhanced biodiversity, weed management and growth reduction (Guerra and Steenwerth 2012; Pardini et al. 2002; Vukicevich et al. 2016). Depending on the desired effect, groundcover may consist of various perennial or annual herbaceous plants. Historically, groundcover has been planted in an interrow of approximately 1-2 meters; however, the crop-row area (typically 1-meter wide) has been maintained with herbicide or tillage due to concerns of yield penalties attributable to belowground competition for water and/or nutrients (Guerra and Steenwerth 2012; Merwin et al. 1994a). In regions with ample precipitation and fertile soils, interest grows in extending groundcover directly beneath mature grapevines to control excessive vegetative growth with minimal yield penalty (Hickey et al. 2016; Tesic et al. 2007). This balances vegetative and reproductive growth, an important strategy to improve wine quality and lower disease pressure (Marais et al. 1992; Valdes-Gomez, 2011).
Grapevine aboveground response to full groundcover is highly context dependent, ranging from 0% (Jordan et al. 2016) to approximately 59% vegetative and 49% reproductive growth reductions (Karl et al. 2016). The variability in growth reduction is often attributed to soil properties, seasonal resource availability, groundcover type, and vine age (Hatch et al. 2011; Hickey et al. 2016; Jordan et al. 2016; Karl et al. 2016) and has necessitated belowground investigations in order to define mechanisms of competition (Centinari et al. 2016; Klodd et al. 2016). Plants that successfully maintain growth in competitive soil environments tend to have high root density, high root surface area, and plasticity of root growth (Casper and Jackson 1997). Plastic root systems may respond to heterogeneous supplies of resources by increasing root length to mass ratio (specific root length (SRL), cm g-1), decreasing diameter, increasing association with beneficial microbial symbionts (i.e., mycorrhizal fungal colonization) and increasing density and length of roots in resource-rich patches (Hodge 2004).
In mature grapevines, roots can cope with groundcover competition by avoiding shallow soil regions of high groundcover root length density and migrating into deeper soils not heavily colonized by groundcover roots, so that complementary use of water and nutrients is possible (Celette et al. 2005; Centinari et al. 2016; Klodd et al. 2016). This avoidance appears to be further complemented by decreased root lifespan (Centinari et al. 2016), but not necessarily morphological shifts in specific root length, diameter, mycorrhizal colonization, or root branching (Klodd et al. 2016). It is not clear whether the root responses to groundcover observed in mature grapevines are applicable to young grapevines when groundcover is first established.
Besides age, the ability for a grapevine to cope with belowground competition may be dependent on rootstock. An additional practice to control vigor in perennial fruit crops is the grafting scions on to devigorating rootstocks, where rootstocks termed “low” or “medium” vigor refer to observations of scion vegetative growth without groundcover. To our knowledge, only one study has compared scion response to groundcover competition when grafted on rootstocks varying vigor. While this investigation concluded that both practices may favorably reduce vegetative growth, rootstock-dependent decreases were not explored in the first year of groundcover establishment (Hatch et al. 2011) nor as vines acclimated to competition over seven years (Hickey et al. 2016; Klodd et al. 2016)
Due to a lack of replicated studies under field conditions, very little is known on how grapevine rootstocks vary in root growth rate, distribution, and morphological traits (Smart et al. 2006). While plants of a higher growth rate are often presumed to exhibit greater morphological plasticity, studies are generally between broad functional classifications (not within genera) and evidence is inconclusive (Hodge 2004). Grapevine rootstocks that promote higher scion mass may have higher root density (Swanepoel and Southey 1989), nitrogen uptake capacity (Nikolaou et al. 2000, Hatch et al. 2011), hydraulic conductivity (Alsina et al. 2011), and plastic root growth to limited water availability (Bauerle et al. 2008).
Cooperators
Research
A note regarding changes from the proposed study:
Late summer and early fall 2016, much of the project time was spent on troubleshooting a rain-out shelter design to impose a drought. We ran into several unanticipated obstacles on how to account for factors such as tractor movement and belowground water flow. Although we were able to find a construction solution, the costs were far beyond the scope of this grant. As a result, in order to examine the influence of water limitations, we decided to rely on variations in irrigation if an extended dry period occurred during the summer of 2017 (it did not). This also meant needing to move the project from the commercial vineyard of our farmer-cooperator, which did not have irrigation installed, to a vineyard located at the Penn State Agricultural Experiment Station (Rock Springs, PA). This shift allowed for an additional benefit of being able to study two rootstocks and install 1-m deep root boxes (as opposed to 30 cm deep) in the spring of 2017.
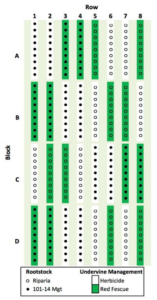
Study site
This study took place between May 2017 and March 2018 in a Noiret (interspecific Vitis vinifera hybrid) vineyard at The Pennsylvania State University Russell E. Larson Agricultural Research Center in Pennsylvania Furnace, PA (40°71’N; 77°97’W). Noiret is reported to have high vegetative growth and rootstock grafts are recommended for long-term productivity (Reisch et al. 2006; Vanden Heuvel et al. 2013). Eight rows of 36 vines each were planted in 2015 with 2.4 m x 2.7 m intra- and inter-row spacing. Rows were oriented north-to-south parallel to a 3-8% slope. The soil type was a Murrill Channery silt-loam with an estimated rooting depth of 217 cm (NRCS Soil Survey 2018). Vines were maintained in accordance with standard management practices for hybrid grapevines in the eastern USA (Wolf 2008). At the time of vineyard establishment, hard fescue (Festuca brevipila) was planted as inter-row grass groundcover and an 85 cm bare soil strip was maintained under all vines using repeated glyphosate herbicide applications until September 2016. During the study, no irrigation was needed and vines were standardized to approximately 32 shoots per vine and 1 to 2 clusters per shoot.
The experimental design for this study was a split-plot rootstock vigor (2 levels) and groundcover (2 levels) factorial with four replications for a total of 16 experimental units. Each experimental unit consisted of two randomly assigned 9-vine subplot row-sections, where the first and last vine in each subplot was excluded from data collection (14 data vines per experimental unit). As additional buffer, the vineyard was bordered by three-vine sections at the beginning and end of each row and by one row east and one west of the experimental plot. As a main-plot factor, half of the Noiret vines (n = 144) were grafted on the low-vigor rootstock Riparia (Vitis riparia), while the other half (n = 144) on the medium-vigor rootstock 101-14 Mgt (Vitis riparia x Vitis rupestris).
The randomized sub-plot treatment of grass groundcover was established in September 2016. The 85-cm under-vine area was either continued as bare soil (=no-groundcover treatment) or tilled and hand-seeded with a creeping red fescue (Festuca rubra L. ssp. rubra) grass groundcover at a rate of 280 kg/ha (=groundcover treatment). Creeping red fescue is a cool season grass often planted for erosion control, turf, or orchard groundcover (USDA, 2006). It has a relatively shallow rooting depth (approximately 40 cm) and height as compared to other cool season grasses (30-40 cm; Brown et al. 2010). The grass groundcover was not fertilized or mowed during this study. The bare soil plots were maintained with herbicide applications on June 11 (carfentrazone-ethyl, oryzalin, norflurazon, and methylated seed oil mixture), July 17 (glufosinate), and November 14 (rimsulfuron and glufosinate mixture). Throughout the study rainfall, solar radiation, and air temperature were recorded hourly with a weather station located at the vineyard site (NEWA, 2018).
Grapevine aboveground growth and nutrient status
Grapevines were harvested on October 5, 2017 and yield was recorded as the total fresh weight (kg) per experimental unit. Total number of clusters was counted and cluster weights were calculated (g/cluster). Sixteen to twenty clusters per subreplicate were collected, frozen, and berry weight (g/berry) was determined from 400 randomly selected berries per experimental unit. Pruning weight (kg) was measured on dormant vines on March 30, 2018 as the mass of one-year-old shoots (canes) produced during the 2017 growing season. The yield and pruning weight measures were averaged to a vine-basis based on the actual number of vines in each experimental unit (kg/vine).
Nutrient status of the grapevines was assessed by late season leaf petiole analysis (Wolf 2008). On August 20, 2017 all petioles were collected from 8 shoots per experimental unit and dried at 70 ᵒC for 48 hours. Nutrient content in vegetative and reproductive tissues produced during the growing season was assessed shortly before harvest. On October 1, 8 shoots per experimental unit were collected and divided into leaf blade, stem, and cluster components, dried at 70ᵒC for 5 days for vegetative tissues and 8 days for clusters, and weighed. All plant tissues were ground and submitted to Pennsylvania State University Agricultural Analytical Services Laboratory for nutrient analysis. Phosphorus (P) and potassium (K) were analyzed by acid digestion (Huang et al. 1985) and total nitrogen (N) by combustion (Horneck and Miller 1998). Nutrient concentrations of leaves, shoots, and clusters at harvest was scaled to whole-plant nutrient content based on mass per shoot of tissue samples and average number of shoots per vine within each experimental unit.
Root distribution and morphological traits
Four 80 to 100-cm-deep soil cores were collected in each experimental unit on either August 30 or September 1, after seasonal peak of grapevine root growth has typically occurred and root standing crop may be greatest (Radville et al. 2016). Cores were 5.2 cm in diameter and each core was separated into five depth increments: 0-10, 10-20, 20-40, 40-60, 60-100 cm. Each depth increment was vertically divided in two halves and each half was randomly assigned to soil nutrient analyses or root length analyses. Samples were transported on ice to The Pennsylvania State University. Those assigned to root length analysis were stored at 4 ᵒC for no more than 75 days and wet sieved through a 2 mm sieve to separate grapevine roots from groundcover roots. Grapevine roots were easily differentiated from groundcover roots based on darker coloration, relatively large diameter, and acute branching angles (Klodd et al. 2015).
Grapevine roots were classified as either absorptive or transportive based on root order, where the most distal root segments are considered first order (Pregitzer et al. 2002; McCormack et al. 2015). First and second order roots were considered primarily absorptive while third order and greater were considered primarily transportive (Valenzuela-Estrada et al. 2008, Klodd et al. 2016). All grapevine roots within each classification were laid on transparent plastic sheets and scanned. Scanned root images were analyzed for root length and diameter in WinRhizo image analysis software (Regent Instruments, Inc., Canada). All grapevine and groundcover roots were dried at 60 ᵒC for 48 hours and weighed. For grapevine absorptive roots within each depth, root length was divided by soil volume of each sample to calculate root length density (RLD; cm cm-3), by total absorptive root length to calculate proportion of root length (%), or mass of each sample to calculate SRL (cm g-1). Groundcover root length was estimated from root mass based on the average SRL of Festuca rubra across heterogeneous soil conditions as reported by Mommer et al 2011 (424 m g-1), which was consistent with other reported values (e.g., Boot and Mensink 1990).
Arbuscular mycorrhizal fungal colonization
Arbuscular mycorrhizal fungal colonization (% of root length) was determined from 12 roots per experimental unit within three depth increments only (0-10, 20-40, 60-100 cm). Roots were rehydrated in 70% ethanol, boiled in 10% potassium hydroxide, cleared in 3% alkaline hydrogen peroxide, and re-acidified in 5% hydrogen chloride. They were then stained for 30 minutes in trypan blue dye and stored in 1:1:1 glycerol:lactic acid:deionized water destaining solution (Klodd et al. 20165). Percent arbuscular mycorrhizal colonization was determined on a root-by-root basis using a standard line-intercept method with a light microscope. At each intercept any fungal identifying structures (arbuscules, hyphal coils, spores, vesicles) were noted in order to classify hyphae as mycorrhizal or non-mycorrhizal (Resendes et al. 2008).
Soil nutrient availability
Within eight hours of collection, the soil samples assigned to nutrient analysis were dry sieved through a 2-mm sieve to separate roots and organic matter from bulk soil. Bulk soil was then dried at 60 ᵒC for 48 hours and submitted to The Pennsylvania State University Agricultural Analytical Services Laboratory for nutrient analyses. Phosphorus (P) and potassium (K) were analyzed by Mehlich 3 ICP (Wolf and Beegle 2011) and nitrate (NO3) (Griffin et al. 2011) and ammonium (NH4) (Mulvaney 1996) by specific ion electrode.
Soil moisture
In June 2017, four soil moisture probe access tubes were installed in each experimental unit to a depth of 100 cm. Soil water content (percent volume) was measured by a frequency domain refractometer probe (PR2, Delta-T Devices; Cambridge, UK) weekly from July 17 until October 10 (14 measurement dates total) in each access tube simultaneously at depths of 10, 20, 40, 60, and 100 cm.
Relative Water Uptake
Between August 3 and 10 2017, twenty flexible vinyl tubes with an 0.8 cm inner diameter were installed to a depth of 40 cm at one randomly selected vine in each subreplicate (32 vines total). Tubes were installed centered on every other centimeter to create a 40 cm transect located 50 cm from the vine base. Before dawn on September 8, 5 mL of water enriched with 70% of the deuterium isotope (2H) was applied to each tube (100 mL per vine). Transpired water was collected daily, by sealing two shoots per vine in plastic bags for approximately 2-5 hours, until 10-15 mL of water was collected in the bags. Transpired water samples were collected for one week, from one day prior to deuterium application (September 7) until six days after (September 14). Samples were submitted to the Cornell Stable Isotopes Laboratory for percent deuterium enrichment. The difference between the isotope enrichment values of the baseline (September 7) and days post application (September 8-14) was calculated; all days of collection without rainfall (September 11-14) were summed to represent the magnitude of isotope uptake, this was used as a proxy for relative water uptake from 40 cm.
Grapevine Water Status and Gas Exchange
Predawn stem water potential (September 10) and midday stem water potential (September 11) were measured on four mid-shoot leaves per experimental unit (16 per treatment) with a pressure chamber (Plant Water Status Console 3000, Soil Moisture Equipment Corp., Santa Barbara, CA, USA) to characterize the grapevine water status. Concurrently, on September 10, leaf gas exchange (photosynthesis, transpiration, stomatal conductance) was measured on two mid-shoot leaves per subreplicate (16 per treatment) with a CIRAS-3 portable system (PP Systems, Amesbury, MA). Measurements were taken from 11 am to 2:30 pm. Leaf gas exchange and predawn leaf water potential were repeated as described on September 28 and 29 respectively.
Data analysis
Statistical analyses were done with linear mixed models with the PROC MIXED procedure in SAS software package (SAS Institute Inc., Cary, NC). Interactions between groundcover (gc), rootstock, depth, and date were tested as fixed effects. The four experimental blocks and the interaction of rootstock and block were treated as random effects. Due to the frequent loss of statistical power in split plot designs (Bradley and Russell 1998) and the high variability of root traits (Maeght et al. 2013), both significant interactions (P < 0.05) and marginally significant interactions (P < 0.10) between fixed effects were considered. Interactions were examined with the “by” statement for depth and date and the “lsmeans slice” option for gc and rootstock.
Relative changes (%) between no groundcover (noGC) and groundcover (GC) treatments were determined for each rootstock for measures of yield, cluster weight, pruning weight, and total vegetative nutrient content as well as within depth increments for root length, root diameter, SRL, soil nitrate, and soil moisture. Relative change (RC) was calculated from the difference between no groundcover (noGC) and groundcover (GC) treatments normalized to the no groundcover value.
Growing conditions
The 2017 growing season started with budburst on April 26 and ended with harvest on October 2. There was a total of 536 mm of rainfall, which was comparable to the previous 10- year average (505 mm; NOAA 2018). However, the majority of rainfall occurred between the months of May and August (486 mm) with precipitation on 54% of days; this was considerably wetter than the previous ten year May-August average (418 mm; NOAA 2018). September was much drier than the previous 10-year average (87 mm; NOAA 2018) with only 34 mm of rainfall (Figure 1).
Aboveground growth
Both groundcover and rootstock affected aboveground growth; however, influences differed between reproductive and vegetative measures. Crop yield was not influenced by groundcover or rootstock (Pgc = 0.285, Prootstock = 0.452, Pgc*rootstock = 0.126), but cluster weights were influenced by both (Pgc*rootstock = 0.004; Figure 2). Without groundcover, cluster weights were smaller for vines grafted on the low-vigor rootstock than the medium-vigor rootstock (98 g and 112 g, respectively). With groundcover, cluster weights were higher for vines grafted on the low-vigor rootstock than medium vigor rootstock (90g and 106 g, respectively). While groundcover presence (compared to no groundcover) reduced cluster weights for the medium-vigor rootstock, weights were similar regardless of groundcover presence for the low-vigor rootstock.
Vegetative growth was decreased overall by the presence of groundcover (Pgc = 0.003), but magnitude of decrease tended to be rootstock dependent (Pgc*rootstock = 0.083; Figure 2). For the vines with no groundcover, those grafted on the medium-vigor rootstock had larger pruning weight (1.20 kg/vine) than those grafted on the low-vigor rootstock (0.99 Kg/vine). However, pruning weight was similar for both rootstocks with groundcover presence (0.78-0.79 kg/vine).
Grapevine nutrient status
Vines with groundcover generally had decreased nutrient concentrations in all the vegetative tissues analyzed (Pgc<0.05; Appendix A). The decrease was consistent across tissues for N, while it was tissue-dependent for P and K. When scaled to content of all one-year-old shoots, shoots of vines growing with groundcover had on average 13% less N, 22% less P, and 23% less K content than vines growing without groundcover (Figure 3). While vegetative tissues (shoots and leaves) had lower nutrient content, cluster N, P and K content was not influenced by the presence of groundcover (Appendix A). On the whole, rootstock vigor did not affect nutrient concentration nor content for all tissues analyzed.
Root length and distribution
The grass groundcover differed markedly from grapevines in both amount and distribution of root length. The groundcover had more than an order of magnitude greater root length density (RLD) than the grapevine between 0-10 cm. Groundcover roots had a very shallow root distribution with 80% of roots located between 0-10 cm (Figure 4). Groundcover RLD strongly decreased with depth down to 40 cm and less than 0.1% of total groundcover root length was between 40 and 100 cm (Figure 4; Appendix B). In comparison to the groundcover root distribution, grapevine roots without groundcover were present up to 100 cm and had a more even distribution across depth (Figure 4).
To compare the grapevine RLD and proportion of root length with and without groundcover, three depth increments were defined based on comparisons of groundcover root length and grapevine root length in the no groundcover treatment (Figure 4; Appendix B). The increments were considered high-competition (groundcover RLD greater than grapevine RLD; 0-20 cm), low-competition (groundcover RLD comparable with grapevine RLD; 21-40 cm), and no-competition (grapevine RLD greater than groundcover RLD 41-100 cm).
Groundcover decreased grapevine root length overall (Pgc= 0.005; Appendix B) and influenced root distribution across depth (Pgc*depth < 0.001; Figure 5). As a proportion of total root length, grapevines with groundcover had a deeper root distribution, with a higher percentage of root length deeper than 21 cm regardless of rootstock. In the high-competition zone, the proportion of root length decreased from about 47% to 23%, for both rootstocks (Pgc =0.046). Whereas the proportion of root length increased considerably in the low-competition zone, from about 21% to about 41%, regardless of rootstock (Pgc =0.046). In the no-competition zone, there was no change in distributions as a proportion of root length (Pgc=0.447).
Groundcover did not have as large of an influence on magnitude of root length as on proportion of root length. In the high-competition zone RLD decreased from approximately 2.7-3.2 cm cm-3 (medium-vigor and low-vigor rootstocks respectively) to 1.0 cm cm-3 for both rootstocks (Pgc=0.017). Within the low-competition zone (21- 40 cm), RLD was not affected by the presence of grass groundcover (Pgc =0.169). In the no-competition zone, there was no change in RLD (Pgc=0.254). Overall, there was a 26% reduction in RLD with groundcover (Pgc=0.005).
Compared with the low-vigor rootstock, the medium-vigor rootstock tended to have more roots distributed deeper (Figure 5). In order to examine differences in proportional root length of deep roots between the two rootstocks, we compared the proportional root length of a somewhat shallow increment (0-40 cm) with that of deeper soil layers (41-100 cm). On average, groundcover had no influence within these depth increments (Pgc*depth = 0.630). However, the medium-vigor rootstock had a larger proportion of deep roots than the low-vigor rootstock (40% and 29% respectively; Prootstock = 0.032), resulting in a more even distribution with depth in the medium-vigor rootstoDepth had a stronger influence on root traits than either groundcover or rootstock (Table 1). As depth increased, mean diameter of the absorptive roots increased and SRL and percent arbuscular mycorrhizal fungal colonization of roots decreased (Pdepth < 0.001 for all; Table 1). Vines with groundcover when compared to vines without groundcover, had smaller root diameter and larger SRL, regardless of depth (Pgc = 0.005 and 0.028 respectively). Arbuscular mycorrhizal fungal colonization was not influenced by groundcover; however, it was high (57-81%) in the 0-10 and 21-40 depth increments and substantially lower in the 61-100 depth increment (18-50%; Prootstock < 0.001). Regardless of the groundcover, the low-vigor rootstock tended to have a larger absorptive (Table 1; Prootstock = 0.097) but a smaller transportive (Prootstock = 0.073; Appendix C) root diameter than the medium-vigor rootstock. While rootstock tended to interact with depth for SRL (Prootstock*depth=0.064) and arbuscular mycorrhizal colonization (Prootstock*depth = 0.094), when examined further, there were no meaningful differences between rootstocks within any particular depth increment.
Soil resource availability
Soil availability for N, P, and K decreased with depth and were more strongly influenced by depth (Pdepth<0.001 for all) than groundcover, and not at all influenced by rootstock (Table 2). There was no groundcover influence on NH4 or K. There tended to be an overall 14% decrease in NO3 when groundcover was present (Pgc=0.073). Groundcover influence on available P was inconsistent across depth (Pgc*depth=0.004). Phosphorus tended to be higher (mean response of 38%) in the 0-10 depth increment when groundcover was present than absent (Pgc=0.069). However, the presence of groundcover had no influence on P availability at deeper depths.
Over the course of the season, soil moisture consistently increased with depth (Pdepth <0.001) and fluctuated with rainfall events (Pdate < 0.001), including during a brief dry-down period in September (Figure 6).
Soil moisture was differentially affected by the combined effects of rootstock, groundcover and depth, independent of date (Pgc*rootstock*depth < 0.001). To examine influences of groundcover and rootstock, comparisons were made at each depth for the whole season average (Figure 7). At 20 and 30 cm, rootstocks generally diverged in soil moisture with and without groundcover (Pgc*rootstock = 0.072 at 20 cm and 0.028 at 30 cm). At 20 cm, soil moisture was higher for the low-vigor rootstock with groundcover than all other treatment combinations. At 30 cm without groundcover, soil moisture was similar in the medium-vigor rootstock plots as compared with the low-vigor rootstock plots. However, when groundcover was present, soil moisture diverged with rootstock: soil moisture was higher in the low-vigor rootstock than medium-vigor rootstock plots. Soil moisture tended to be decreased at 60 cm from 20.9% to 19.2% when groundcover was present, regardless of rootstock (Pgc = 0.066).
Relative change in aboveground growth, root distribution and traits, and resource use with groundcover
When presented as a relative change, it is easier to compare the influence of groundcover on reproductive and vegetative growth, root distribution and morphological traits, and resource use (Figure 8). When groundcover was present, there was a clear pattern of decreased aboveground growth, total nutrient content in vegetative tissues, root diameter and soil nitrate and an increase in SRL. However, influences on root length and soil moisture were depth dependent. Yield and pruning weight decreased by 20% and 40%, respectively, for the medium-vigor rootstock, while only pruning weight was affected (19%) for the low-vigor rootstock. In terms of absolute root length, there was a 56% decrease in the high-competition zone and an increase of 111% in the low-competition zone, regardless of rootstock. Overall, vines growing with groundcover had 6% smaller root diameter and 27% greater SRL, and change was not depth or rootstock-dependent. While vegetative nutrient content and total soil nitrate content were both decreased by groundcover (13-23% and 14%, respectively), soil moisture patterns were inconsistently affected across depth and were rootstock dependent. Relative changes were only different between rootstocks for aboveground growth and soil moisture.
Discussion
Even in a normal-to-wet year, we found that groundcover competition substantially decreased vegetative growth in young vines and vegetative growth reductions were greater for the medium-vigor rootstock (40%) than for the low-vigor rootstock (19%). The greater reductions in growth with groundcover presense for vines on the medium-vigor rootstock, when compared to the low-vigor rootstock, essentially mitigated any rootstock influence on vegetative growth. Reproductive growth was less affected by groundcover. Cluster weights were decreased for vines on the medium-vigor rootstock (20%) but vines on low-vigor rootstock maintained cluster weight regardless of groundcover presence. This amounted to a reversal in rootstock response: when compared to the low-vigor rootstock, the medium-vigor rootstock without groundcover had higher cluster weights, but with groundcover had lower cluster weights. This change in cluster weight was not due to a change in berry weight (Appendix D), suggesting a decreased number of berries per cluster (i.e. fruitset) for vines on medium-vigor rootstock with groundcover. Contrary to our hypothesis, young vines grafted on the low-vigor rootstock were more tolerant of competition than those grafted on the medium-vigor rootstock.
The reductions in growth with groundcover were primarily explained by nutrient competition. The vines with groundcover had decreased tissue nutrient concentration and content, in particular in vegetative tissues. While potassium and phosphorus reductions were substantial, nitrogen had the most consistent reductions across grapevine tissues and the soil column. Furthermore, in comparison to target values for late-season nutrient concentrations of petioles, potassium and phosphorus were not deficient regardless of treatment, but nitrogen was at a deficient level (<0.8%) for grapevines without groundcover (0.7%) and even moreso when groundcover was present (0.6%; Wolf 2008). Nitrogen has been shown to be more limiting on grapevine vegetative growth than phosphorus or potassium, while either nitrogen or phosphorus limitations can reduce cluster weights (Schreiner et al. 2013, Skinner and Matthews 1989). We acknowledge that we only examined tissue nutrients of one-year old shoots and did not compare tissue nutrients of perennial organs (trunk or roots); the nutrient concentration or content of these storage organs may be different amongst treatments.
We found belowground evidence of competition that supports mechanisms of nutrient reductions in aboveground tissues. While lower nutrient content in aboveground tissues may be in part due to direct competition for soil nutrients from groundcover roots, there were likely indirect decreases in nutrient uptake due to the overall differences in root growth pattern. Grapevines growing with groundcover had a higher proportion of root length in deeper soil of lower nutrient concentration. Our expectation of deeper nutrient uptake by grapevines with groundcover is supported by higher absorptive root length in deeper soil and a decrease in soil nitrate at depth. Furthermore, there was a suggestion of less reliance on shallow soil P. Grapevines exhibited tissue P reductions despite soil P increasing between 0-10 cm, perhaps due to increased P mineralization with grass presence (Armstrong and Heylar, 1992). We did not find evidence of increased nutrient tissue concentration in grapevines on the medium-vigor rootstock as reported in previous studies (Hickey et al. 2016) or deeper uptake as we expected.
In previous studies with young vines, when growth reduction occurred, it was primarily attributed to competition for water or water-limited uptake of soluble nutrients, with direct nutrient competition considered a secondary factor (Hatch et al. 2011, Karl et al. 2016). With a wet year, we were unable to directly relate differences in soil moisture to limitations on water or nutrient uptake. It is possible that the presence of groundcover promoted higher water infiltration (Merwin et al. 1994b); Furthermore, rainfall was high during bloom (mid-June), the critical time period for current season nutrient uptake (Schreiner et al. 2006). Deeper (60 cm) soil moisture was reduced by groundcover for both rootstocks; however, there was no change in root growth between 41-100 cm to suggest opportunistic plant responses to acquire deeper water. Previous studies that directly measured depth of water uptake (i.e. stable isotope methods) have not shown an increase in deep water uptake for grapevines with groundcover (Klodd et al. 2015) or lianas in competitive forest environments (De Deurwaerder 2018).
The only suggestion of different nutrient or water uptake between rootstocks was patterns of shallow soil moisture. Without groundcover, the there was no difference in soil moisture between the two rootstocks. However, the patterns of soil moisture for the rootstocks were differently influenced by groundcover at shallow depths; in comparison to without groundcover, the low-vigor rootstock had higher water availability (20 and 30 cm), but the medium-vigor rootstock had lower availability (30 cm). While data is not presented here, in the driest month (September) the vines in our study did not exhibit water stress (as measured by predawn and stem water potentials; Appendix E) or a difference in magnitude of water uptake at 40 cm (as measured by a deuterium tracer; Appendix F). When compared with all other treatment combinations, vines grafted on low-vigor rootstock without groundcover may have exhibited greater water use efficiency during dry conditions (P=0.107; Appendix G) but not in relatively-normal conditions. Ultimately, these confounding results do not confirm our hypothesis that groundcover presence would increase deep water uptake, nor does it offer a mechanistic explanation of the difference in growth between rootstocks.
The marked increase in grapevine root length in the 20-40 cm soil zone with groundcover suggests that the young vines in the first year of groundcover establishment coped with competition somewhat differently than grapevines after several years of acclimation (Klodd et al. 2016, Celette et al. 2005). For example, mature grapevines grafted on the same medium-vigor rootstock and groundcover species as in this study, had a substantial decrease (63%) in absorptive root length, mainly in the 0-20 cm zone, but no increase in root length in any other soil depth nor changes in other traits (SRL, branching intensity, root diameter) after seven years of acclimation to groundcover (Klodd et al. 2016). In comparison, while the vines in our study substantially decreased root length in the high-competition soil zone (56%), this decrease was compensated for by a large increase (111%) in the low-competition soil zone; this ultimately lead to only a 26% reduction in overall root length. Furthermore, the vines in our study increased SRL and decreased diameter. We found high mycorrhizal fungal colonization in shallow soil zones, which is not uncommon (Schreiner et al. 2005). Additionally, although low P uptake can lead to increased mycorrhizal colonization in roots, our results are similar to other studies which showed no difference in colonization with groundcover (Baumgartner et al. 2010) or between these two rootstocks (Linderman and Davis 2001).
An increase in root length in a low-competition zone has been reported in the first years of groundcover establishment in young avocados (RLD; Atucha et al. 2013) and in mature grapevines (proportion of root production; Centinari et al. 2016). While grapevines acclimated to groundcover might be able to cope with competition with a reduced but still adequate root system overall (Klodd et al. 2016), young vines with a smaller root system may respond to groundcover establishment by selectively increasing standing crop of roots in less competitive zones. In addition to vine age or years of acclimation, there are other plausible explanations for why we saw a substantial increase in root length in the low-competition zone but no change in the no-competition zone. With the ample precipitation during this study, it is possible that adequate deep rooting for water access had already developed in previous years (Maeght et al. 2013), so in order to compensate for shallow root reductions, preferential root growth was only in the middle soil zone and not the deeper zone as well (Craine et al. 2006). We also acknowledge previous suggestions of allelopathic properties of groundcovers contributing to root avoidance (Klodd et al. 2016; Smith et al. 2001).
Our observations of large changes in root distribution and SRL confirm grapevines’ highly plastic responses to groundcover competition. We did not see a difference in root system size, density, distribution, SRL or mycorrhizal fungal colonization that would explain the different aboveground responses to competition observed between rootstocks. Regardless of groundcover presence, the low-vigor rootstock did have a shallower root distribution, which has been associated with highly dwarfing rootstocks in apple (Ma et al. 2013), and a larger absorptive root diameter, as is common in slower growing species in congeneric contrasts (Comas and Eissenstat 2004). While root size and density are considered important in comparing traits imparted to scion by grapevine rootstocks, these root traits may be more influenced by soil properties and management practices (Smart et al. 2006) or even the scion (Tandonnet et al 2010) than rootstock genetics. In these two rootstocks that share similar parentage, traits that take temporal or flux considerations into account such as root production (Ma et al. 2013; Bauerle et al 2008) or rate of nitrogen uptake (Volder et al. 2005) may be more important in explaining the differing aboveground growth response.
In this study, both groundcover and rootstock management practices reduced grapevine vegetative growth. However, groundcover reduced growth regardless of rootstock, while rootstock only reduced growth when groundcover was not present. The low-vigor rootstock in this study has been shown to have slightly lower hydraulic conductance when compared to the medium-vigor rootstock (Carbonneau 1985). It’s possible that in a normal-to-dry season, the differences in conductance would allow for greater magnitude of water and nutrient uptake by the medium-vigor rootstock (Jones 2012) so that even with groundcover, the medium-vigor rootstock could have higher vegetative growth (Hatch 2011). For both rootstocks, above- and belowground response to groundcover may likely change as vines age and acclimate to groundcover competition (Hickey et al. 2016).
In a wet year, grapevines grafted on a low-vigor rootstock were more tolerant to groundcover competition than those grafted on a medium-vigor rootstock. Vines responded to competition, primarily for nutrients, by reducing absorptive root length in a high-competition soil zone, increasing root length in a low-competition zone, increasing SRL and decreasing root diameter. Aboveground vegetative reductions with differing rootstock vigor were not clearly linked to differences in vine water or nutrient status. This study underscores the importance of mechanistic studies of groundcover competition early in vineyard establishment with an emphasis on examining belowground responses of different rootstocks across temporal scales.
Additional Results (Appendicies)
Rootstock selection and groundcover are two important strategies for sustainable, high-quality grape production. As interest in wine grape production expands to regions with often fertile soil and abundant precipitation, such as the eastern USA, there is potential for these practices to reduce undesirable growth at high-resource sites. Without mechanistic understanding of how rootstock and groundcover management practices control vegetative growth of grapevines, researchers and growers are limited in the ability to fine-tune these practices for applications in new contexts. This thesis project focused on using whole-plant mechanistic observations of grapevines of varying vigor and their response to groundcover competition.
While we confirmed our hypothesis that groundcover reduced growth of grapevines, we unexpectedly observed the greatest growth reductions in grapevines on the medium-vigor rootstock. Growth reductions imparted to the low-vigor rootstock in comparison to the medium-vigor rootstock were mitigated when groundcover was present; the low-vigor rootstock was more tolerant to competition. Our study largely supported previous research that vines coped with groundcover competition through a plastic root system response and deeper resource use. The study took place during a normal-to-wet year and grapevines did not exhibit water stress but substantially reduced nutrient concentration and content in vegetative tissues. Tissue nutrient reductions were clearly linked to mechanistic observations belowground. Roots redistributed deeper and augmented root morphological traits (diameter and SRL). Soil nitrate was less available in soil with groundcover compared to without groundcover. While water competition was likely not a limiting factor to growth, the difficulty in quantifying water uptake from any particular depth should be noted. Future studies in climates where dry-down periods are not predictable would benefit from methods that more precisely determine depth of water uptake (i.e. dual isotope pulses; Caldwell and Eissenstat 1987).
The methods in this study did not identify the mechanisms by which the rootstocks imparted different scion growth. While root distribution and morphology have previously been useful in comparing plasticity of growth between functional classifications of plants, temporal traits of roots, such as turnover or water conductance may be more indicative of plasticity within genera. It is possible that gene expression may ultimately be most determinate of differences in scion response between rootstocks. Future studies relating rootstock genetics to scion traits would benefit from incorporating temporal-based root traits and identifying which gene loci confer desirable scion phenotypes (i.e. hormone metabolism, aquaporin expression or rate of stomatal closure).
In addition to furthering mechanistic understanding of grapevine-groundcover competition, this study provided directly applicable information for growers. When vines are young, groundcover may be a more dependable approach to reducing vegetative vigor than rootstock, however vegetative growth reductions may be greater than desirable for long-term vine health. Coupling the low-vigor rootstock in this study (Riparia) with groundcover may offer the unique opportunity to harness the known ecological benefits of groundcover, reduce vegetative growth, and maintain yield. This study also provided evidence that even if a site is not water-limited, groundcover may still compete with young grapevines for nutrients. If a grower does decide to plant groundcover when vines are young, plant nutrient status should be closely monitored and nitrogen and/or phosphorus applications should be considered.
When compared to a study in Virginia with similar manipulated treatments and climate, this work reaffirmed the context-dependence of grapevine response to belowground management practices (Hickey et al. 2016). Growth differences between rootstocks were present regardless of groundcover when vines were young in the Virginia study, but not in the work presented here. Soil properties, seasonal-weather patterns, scion genotype, and canopy management practices all differed between these two studies. While the root response of the young vines in this study was markedly different from mature vines at the Virginia site, we are limited in our ability to directly compare how vines responded between the two sites without evidence of root responses in young vines at the Virginia site. Concurrent, multi-year studies across several sites may address this gap and offer opportunities for modeling growth; however, they are highly intensive and rarely funded. Ultimately, this work emphasizes the importance of mechanistic studies that span multiple management practices and occur early in perennial crop establishment.
Education & Outreach Activities and Participation Summary
Participation Summary:
Fleishman, S, D Eissenstat, M Centinari. Do Cover Crops Stabilize Wine Grape Productivity?. Our Farms Our Future Conference: SARE and ATTRA. 2018 April 3-4; St. Louis, Missouri. Poster Presentation.
Fleishman, S, D Eissenstat, M Centinari. Controls on grapevine vigor: decoupling groundcover competition and rootstock vigor. American Society for Enology and Viticulture - Eastern Section Conference: SARE and ATTRA. 2018 June 12-13; King of Prussia, Pennsylvania. Student Talk.
On Farm Tour, Oct 24: NCCC-212 Small Fruit and Viticulture Research Group and Coordinating Committee (12 Participants)
On Farm Tour, Aug 22: HORT 431 Small Fruit Culture Course Presentation (12 participants)
Project Outcomes
This project is the first in-depth investigation of cover crop competition with grapevines in Pennsylvania. Combined with results from other regional investigations, it provides further evidence that cover crops can provide benefits without necessarily sacrificing yield. Once the 2017 and 2018 year’s data are analyzed and presented in future outreach and extension efforts, this project has the potential to assist in reducing risks of cover crop adoption for viticulturists in the region by presenting growers with specific information for hybrid vines and in a wet year. This will allow growers to make the most informed decisions on incorporating under vine cover crops as a sustainable vineyard practice.
Long-term, this project has provided the foundation for long term study and an in depth investigation. The study will be extended over the next three years with an AFRI Foundational grant ($500,000) to examine the microbiome on grapevine roots and as influenced by cover crops. Concurrently, yield and soil moisture measurements will continue in order to examine treatment influences on growth.
This project required the installation and management of large field equipment. Belowground processes are very difficult to study and grapevines have a deep root distribution, to our knowledge root boxes to this depth have not been installed in a replicated field experiment previously. This helped me understand the logistical difficulty with studying sustainable practices with respect specifically to soil function.
This project was the core of the thesis culminating in my graduation with a MS horticulture degree in August of 2018 from Penn State. My experience with this project encouraged me to commit to a PhD program in Ecology to improve my background in ecology in order to apply it to research in agricultural ecosystems. I will expand upon concepts explored in this grant through a more in-depth investigation of how cover crops shift soil microbial communities (collaboration with Dr. Terrance Bell) and developing a model to help better predict how cover crop selection may impact grapevine growth (collaboration with Dr. Armen Kemanian). This PhD will facilitate my career plans in agroecological research, teaching, and outreach.