Final report for GNE16-132
Project Information
Halyomorpha halys (Stål) is a serious invasive pest of agriculture in the Northeast. Halyomorpha halys feeding damage is economically injurious in a multitude of crops in this region including tree fruit, vegetable crops, and row crops. Many growers in the Mid-Atlantic have abandoned long-standing IPM programs in response to H. halys pest pressure in favor of weekly spray programs. Sustainable management options are being explored to combat this trend including the potential impact of natural enemies. Current research indicates that native predators and parasitoids are ineffective at providing natural control of this pest in the egg stage however, little is known about the predator complex affecting the nymphal stage. The goal of this study was to identify predation rates on H. halys nymphs alone and in the presence of alternate prey, soybean aphid (Aphis glycines, Matsumura). Prey treatments included H. halys alone, H. halys with A. glycines and Aphis glycines alone. These prey were exposed to potential predation from either Hippodamia convergens (Guerin-Meneville) or Podisus maculiventris (Say) nymphs on immature soybean (Glycine max L.) plants for three weeks. Plant growth and insect abundance were monitored twice per week throughout the three week experiment and after, total dry plant biomass was measured. Prey treatments significantly affected plant vertical growth, lateral bud development and final dry mass but in most cases, predator treatments did not significantly reduce the negative effects of herbivory. Plant health metrics did not differ between the A. glycines alone and A. glycines + H. halys prey treatments. Unexpectedly, H. halys survivorship was significantly higher on plants with A. glycines than on those without aphids. Possible causes of this effect are discussed. Due to the strong treatment effect of A. glycines feeding on developing soybeans, the ability of natural enemies to control H. halys in the presence of alternate prey was not demonstrated with the selected combinations of predator and alternate prey species.
Introduction:
Halyomorpha halys (Stål) (Hemiptera: Pentatomidae) is an economically significant invasive agricultural pest in the mid-Atlantic region. Native to East Asia, H. halys was first confirmed in the US in 2001 (Hoebeke and Carter, 2003). Since its initial detection in Allentown, PA, H. halys has spread to over 40 states and has been detected in Canada, and Europe (Fogain and Graff, 2011; Harris, 2010; Vétek et al., 2014; Wyniger et al., 2014). Halyomorpha halys is highly mobile and capable of feeding on over 170 species of plants, leading to its classification as a high risk pest by the Animal and Plant Health Inspection Service, Plant Protection and Quarantine program (Holtz and Kamminga, 2010; “StopBMSB.org,” 2017). Economic damage from BMSB was first recorded in 2006 and subsequent economic losses have increased drastically (Leskey et al., 2012b; Nielsen and Hamilton, 2009b). During the 2010 growing season, H. halys caused significant economic injury to peach, Asian pear, cherry, tomato, corn, and soybeans (Holtz and Kamminga, 2010; Leskey, 2010) and an estimated $37 million in damage to Mid-Atlantic apple production (Leskey et al., 2012a). In New Jersey, Maryland, and West Virginia, some stone fruit growers have reported up to 90% yield loss from BMSB damage (Leskey et al., 2012b). In response to high levels of H. halys damage, insecticide applications in some Mid-Atlantic tree fruit orchards have increased by ~4 fold (Leskey et al., 2012b). Such an approach is disruptive to the agroecosystem and resurgences of secondary pests are occurring in multiple crops due to H. halys management programs (Rice et al., 2014).
According to the Enemy Release Hypothesis, H. halys may succeed in invaded ranges due to a lack of closely evolved natural enemies (Keane and Crawley, 2002). However, as a polyphagous pest species, H. halys provides the opportunity to study the impact of naïve natural enemies across multiple agricultural systems. The effect of predators and parasitoids on H. halys eggs in US agricultural systems was initially evaluated in a multi-state study using sentinel egg masses and laboratory studies. Ogburn et al. (2016) found low utilization of sentinel H. halys eggs by Hymenopteran parasitoids but predators had significantly higher impact. In New Jersey, predators were responsible for 4-9% of egg mortality compared to < 1% mortality from parasitoids (Ogburn et al., 2016). In New Jersey, more sentinel egg masses were preyed upon in soybean than any other crop tested. Based on the results of laboratory no-choice predation bioassays, generalist predators in the families Acrididae, Carabidae, Forficulidae, Gryllidae, Pentatomidae, Salticidae, and Tettigoniidae consume H. halys eggs (Abram et al., 2014; Morrison et al., 2016). Generalist predators in the families Nabidae, Reduviidae, and Pentatomidae caused significant reduction in survivorship of H. halys 1st and 2nd instar nymphs in laboratory predation assays (Pote and Nielsen, in press). Understanding the effect of predators on H. halys is expected to decrease the intensity of insecticide-based management programs and allow for the implementation of conservation biological control as a preventative management tool for this invasive pest.
As a polyphagous and highly mobile pest, field crops like soybean, represent an abundant food resource for H. halys prior to overwintering (Venugopal et al., 2015). In the US, H. halys was first described in soybean in 2006 and has subsequently become the dominant species of Pentatomidae found in soybean across several Mid-Atlantic states (Nielsen et al., 2011; Nielsen and Hamilton, 2009a; Owens et al., 2013). Stink bug feeding damage on soybean is caused by the insertion of the piercing sucking mouthparts into pod or seed tissues and by the release of digestive enzymes which destroys cells and dissolves proteins (McPherson and McPherson, 2000). Owens et al. (2013) found that intense H. halys feeding damage can destroy developing pods, decrease final seed weight, and can delay soybean maturation, which is consistent with feeding by endemic stink bugs (McPherson and McPherson 2000). Halyomorpha halys feeding can lead to a “stay green” effect wherein soybean plant senescence is delayed in patches which have experienced high feeding damage by H. halys, particularly along the edge (Leskey et al., 2012a; Venugopal et al., 2014). Inconsistent maturation can lead to delayed harvest times and lost yield because immature soybean plants can damage mechanical harvesting equipment (Venugopal et al., 2014), but little is known about the effect of predation and parasitism on H. halys populations and subsequent plant damage in this cropping system.
Generalist natural enemies are abundant in soybean (Costamagna and Landis, 2006; Rutledge et al., 2004). Multiple studies have shown how these organisms, specifically at the landscape scale, can provide natural enemy services (Gardiner et al., 2009; Landis et al., 2000; Rutledge et al., 2004; Tonhasca, 1993). Aphis glycines (L.) (Hemiptera: Aphididae), an invasive aphid pest of soybeans, is widely consumed by generalist predators in soybean systems (Rutledge et al., 2004). These predators include aphidophagous Coccinellids like Hippodamia convergens (Guérin-Méneville) (Coleoptera: Coccinellidae) which have substantially increased in abundance since the invasion of A. glycines and are now considered effective natural enemies of this pest (Ragsdale et al., 2011). Generalist natural enemies have been shown to decrease aphid populations by 95% in some cases and can cause a detectable trophic cascade, doubling plant biomass and yield compared to those grown in predator exclusion cages (Costamagna et al., 2008, 2007; Fox et al., 2004; Ragsdale et al., 2011; Rutledge et al., 2004).
Endemic predators are less successful at controlling stink bug pests in soybean (Yeargan, 1979). One study found that predation accounted for <20% of the egg mortality of Euschistus servus (Say) (Hemiptera: Pentatomidae) (Stam et al., 1987). Podisus maculiventris (Say) (Hemiptera: Pentatomidae), a predator of H. halys eggs and nymphs (Morrison et al. 2016, Pote and Nielsen, unpublished), is relatively common in soybeans (O’Neil, 1988) although the impact of P. maculiventris and other native predators on H. halys populations and resultant crop damage in soy has not been assessed. For many native stink bugs in soybean, parasitoids are the primary agent of top-down suppression, such as Telenomus podisi (Ashmead) (Hymenoptera: Platygastridae), a parasitoid used as an augmentative biological control agent for control of Euschistus servus (Say) (Moraes et al., 2005, 2009). In soybeans, H. halys sentinel egg masses are infrequently parasitized successfully, but experience relatively high predation, indicating that the latter may be a more important source of H. halys suppression (Ogburn et al., 2016). Given the shared native range of H. halys and A. glycines (Blackman and Eastop, 2014; Xu et al., 2013), their interactions within soybean ecosystems could have important implications for the ecology and management of these pests.
Aphis glycines, like H. halys, is an invasive pest of soybean native to eastern Asia (Blackman and Eastop, 2014). A. glycines was first recorded in the US in 2000 (Alleman et al., 2002), but by the end of that year A. glycines had been detected in 10 states in the Upper Midwest (Venette and Ragsdale, 2004). Although A. glycines is believed to have existed within the US prior to 2000, within three years of its first official detection, it had spread to 30 US states and three Canadian provinces (Venette and Ragsdale, 2004). A. glycines populations cycle between Rhamnus spp., their preferred overwintering and early spring host, and soybean, their summer or secondary host, through the production of winged morphs in the spring and fall (Ragsdale et al., 2004). Winged morphs may also be produced during the summer months in response to high population densities. Winged morphs allow A. glycines to invade soybean fields in geographic regions distant from their overwintering range, threatening soybean production across central North America (Ragsdale et al., 2004). Although they share a common host plant and an evolutionarily ancestral range, potential natural enemy- or host plant-mediated interactions between H. halys and A. glycines have not been well studied.
Our research has shown that multiple generalist predator species will attack H. halys nymphs in laboratory microcosm experiments (Pote and Nielsen, in press). However, this research has not been replicated in more natural settings or in the presence of alternate prey items. Many of the predators known to affect H. halys in laboratory settings are relatively abundant in soybean agroecosystems (Pote, unpublished, O’Neil, 1988) but it is unclear if impact of predation by these predators is sufficient to protect plant health and development. Given the shared ecological traits of H. halys and A. glycines, the trophic interactions between these pests and potential predators should be more clearly understood. Thus, the objectives of this research were to 1) quantify realized predation rates on H. halys in greenhouse soybean mesocosms, 2) quantify realized predation rates on H. halys in the presence of alternate prey (A. glycines), and 3) determine the effect of predator and prey presence on the growth of soybean plants within greenhouse mesocosms.
(StopBMSB.org) Northeastern IPM Center. 2017. StopBMSB.org. (http://www.stopbmsb.org/where-is-bmsb/state-by-state/).
Abram, P. K., T. D. Gariepy, G. Boivin, and J. Brodeur. 2014. An invasive stink bug as an evolutionary trap for an indigenous egg parasitoid. Biol. Invasions. 16: 1387–1395.
Alleman, R., C. Grau, and D. Hogg. 2002. Soybean aphid host range and virus transmission efficiency, pp. 1–3. In Proc. Wisconsin Fertil. Agline Pest Manag. Conf.
Blackman, R., and V. Eastop. 2014. Aphids on the world’s plants. John Wiley & Sons Ltd.
Costamagna, A. C., D. A. Landis, and M. J. Brewer. 2008. The role of natural enemy guilds in Aphis glycines suppression. Biol. Control. 45: 368–379.
Costamagna, A. C., D. A. Landis, and C. D. Difonzo. 2007. Suppression of soybean aphid by generalist predators results in a trophic cascade in soybeans. Ecol. Appl. 17: 441–451.
Costamagna, A., and D. Landis. 2006. Predators exert top-down control of soybean aphid across a gradient of agricultural management systems. Ecol. Appl. 16: 1619–1628.
Fogain, R., and S. Graff. 2011. First records of the invasive pest, Halyomorpha halys (Hemiptera: Pentatomidae), in Ontario and Quebec. J. Entomol. Soc. Ontario. 142: 45–48.
Fox, T. B., D. A. Landis, F. F. Cardoso, and C. D. Difonzo. 2004. Predators suppress Aphis glycines Matsumura population growth in soybean. Environ. Entomol. 33: 608–618.
Gardiner, M. M., D. A. Landis, C. Gratton, C. D. DiFonzo, M. O’Neal, J. M. Chacon, M. T. Wayo, N. P. Schmidt, E. E. Mueller, and G. E. Heimpel. 2009. Landscape diversity enhances biological control of an introduced crop pest in the north-central USA. Ecol. Appl. 19: 143–154.
Harris, A. C. 2010. Halyomorpha halys (Hemiptera: Pentatomidae) and Protaetia brevitarsis (Coleoptera: Scarabaeidae: Cetoniinae) intercepted in Dunedin . Weta. 44: 42–44.
Hoebeke, E. R., and M. E. Carter. 2003. Halyomorpha halys Stål (Heteroptera: Pentatomidae): a polyphagous plant pest from Asia newly detected in North America. Proc. Entomol. Soc. Washingt. 105: 225–237.
Holtz, T., and K. Kamminga. 2010. Qualitative analysis of the pest risk potential of the brown marmorated stink bug (BMSB), Halyomorpha halys (Stål), in the United States. USDA APHIS-PPQ.
Keane, R. M., and M. J. Crawley. 2002. Exotic plant invasions and the enemy release hypothesis. Trends Ecol. Evol. 17: 164–170.
Landis, D. A., S. D. Wratten, and G. M. Gurr. 2000. Habitat management to conserve natural enemies of arthropod pests in agriculture. Annu. Rev. Entomol. 45: 175–201.
Leskey, T. C. 2010. 2010 Tree Fruit Damage Survey.
Leskey, T. C., G. C. Hamilton, A. L. Nielsen, D. F. Polk, C. Rodriguez-Saona, J. C. Bergh, D. A. Herbert, T. P. Kuhar, D. Pfeiffer, G. P. Dively, C. R. R. Hooks, M. J. Raupp, P. M. Shrewsbury, G. Krawczyk, P. W. Shearer, J. Whalen, C. Koplinka-Loehr, E. Myers, D. Inkley, K. a. Hoelmer, D.-H. Lee, and S. E. Wright. 2012. Pest status of the brown marmorated stink bug, Halyomorpha halys in the USA. Outlooks Pest Manag. 23: 218–226.
Leskey, T. C., B. D. Short, B. R. Butler, and S. E. Wright. 2012. Impact of the invasive brown marmorated stink bug, Halyomorpha halys (Stål), in mid-atlantic tree fruit orchards in the United States: case studies of commercial management. Psyche (Stuttg). 2012: 1–14.
McPherson, J., and R. McPherson. 2000. Stink bugs of economic importance in America north of Mexico. CRC Press, Boca Raton.
Moraes, M. C. B., R. A. Laumann, M. Pareja, F. T. P. S. Sereno, M. F. F. Michereff, M. A. Birkett, J. A. Pickett, and M. Borges. 2009. Attraction of the stink bug egg parasitoid Telenomus podisi to defence signals from soybean activated by treatment with cis -jasmone. Entomol. Exp. Appl. 131: 178–188.
Moraes, M., R. Laumann, E. R. Sujii, C. Pires, and M. Borges. 2005. Induced volatiles in soybean and pigeon pea plants artificially infested with the neotropical brown stink bug, Euschistus heros, and their effect on the egg parasitoid, Telenomus podisi. Entomol. Exp. Appl. 115: 227–237.
Morrison, W. R., C. R. Mathews, and T. C. Leskey. 2016. Frequency, efficiency, and physical characteristics of predation by generalist predators of brown marmorated stink bug (Hemiptera: Pentatomidae) eggs. Biol. Control. 97: 120–130.
Nielsen, A. L., and G. Hamilton. 2009a. Life history of the invasive species Halyomorpha halys (Hemiptera: Pentatomidae) in Northeastern United States. Ann. Entomol. Soc. Am. 108: 608–616.
Nielsen, A. L., and G. C. Hamilton. 2009b. Seasonal occurrence and impact of Halyomorpha halys (Hemiptera: Pentatomidae) in tree fruit. J. Econ. Entomol. 102: 1133–1140.
Nielsen, A. L., G. C. Hamilton, and P. W. Shearer. 2011. Seasonal phenology and monitoring of the non-native Halyomorpha halys (Hemiptera: Pentatomidae) in soybean. Environ. Entomol. 40: 231–238.
O’Neil, R. J. 1988. Predation by Podisus maculiventris (Say) on Mexican bean beetle, Epilachna varivestis Mulsant, in Indiana soybeans. Can. Entomol. 120: 161–166.
Ogburn, E. C., R. Bessin, C. Dieckhoff, R. Dobson, M. Grieshop, K. A. Hoelmer, C. Mathews, J. Moore, A. L. Nielsen, K. Poley, J. M. Pote, M. Rogers, C. Welty, and J. F. Walgenbach. 2016. Natural enemy impact on eggs of the invasive brown marmorated stink bug, Halyomorpha halys (Stål) (Hemiptera: Pentatomidae), in organic agroecosystems: A regional assessment. Biol. Control. 101: 39–51.
Owens, D. R., D. A. Herbert, G. P. Dively, D. D. Reisig, and T. P. Kuhar. 2013. Does feeding by Halyomorpha halys (Hemiptera: Pentatomidae) reduce soybean seed quality and yield? J. Econ. Entomol. 106: 1317–1323.
Pote, J. M., and A. L. Nielsen. 2017. Life stage specific predation of Halyomorpha halys (Stål) by generalist predators. Biol. Control. 114: 1–7.
Ragsdale, D., D. Landis, and J. Brodeur. 2011. Ecology and management of the soybean aphid in North America. Annu. Rev. Entomol. 56: 375–399.
Rice, K. B., C. J. Bergh, E. J. Bergmann, D. J. Biddinger, C. Dieckhoff, G. Dively, H. Fraser, T. Gariepy, G. Hamilton, T. Haye, A. Herbert, K. Hoelmer, C. R. Hooks, A. Jones, G. Krawczyk, T. Kuhar, H. Martinson, W. Mitchell, A. L. Nielsen, D. G. Pfeiffer, M. J. Raupp, C. Rodriguez-Saona, P. Shearer, P. Shrewsbury, P. D. Venugopal, J. Whalen, N. G. Wiman, T. C. Leskey, and J. F. Tooker. 2014. Biology, ecology, and management of brown marmorated stink bug (Hemiptera: Pentatomidae). J. Integr. Pest Manag. 5: 1–13.
Rutledge, C. E., R. J. O’Neil, T. B. Fox, and D. A. Landis. 2004. Soybean aphid predators and their use in integrated pest management. Ann. Entomol. Soc. Am. 97: 240–248.
Stam, P. A., L. D. Newsom, and E. N. Lambremont. 1987. Predation and food as factors affecting survival of Nezara viridula (L.) (Hemiptera: Pentatomidae) in a soybean ecosystem. Environ. Entomol. 16: 1211–1216.
Tonhasca, A. 1993. Effects of agroecosystem diversification on natural enemies of soybean herbivores. Entomol. Exp. Appl. 69: 83–90.
Venette, R. C., and D. W. Ragsdale. 2004. Assessing the invasion by soybean aphid (Homoptera: Aphididae): where will it end? Ann. Entomol. Soc. Am. 97: 219–226.
Venugopal, P. D., P. L. Coffey, G. P. Dively, and W. O. Lamp. 2014. Adjacent habitat influence on stink bug (Hemiptera: Pentatomidae) densities and the associated damage at field corn and soybean edges. PLoS One. 9: 1–10.
Venugopal, P. D., G. P. Dively, and W. O. Lamp. 2015. Spatiotemporal dynamics of the invasive Halyomorpha halys (Hemiptera: Pentatomidae) in and between adjacent corn and soybean fields. J. Econ. Entomol. 108: 2231–41.
Vétek, G., V. Papp, A. Haltrich, and D. Rédei. 2014. First record of the brown marmorated stink bug, Halyomorpha halys (Hemiptera: Heteroptera: Pentatomidae), in Hungary, with description of the genitalia of both sexes. Zootaxa. 3780: 2010–2012.
Wyniger, D., N. History, and M. Basel. 2014. First records of an invasive bug in Europe : Halyomorpha halys Stal (Heteroptera : Pentatomidae). Bull. la Soc. Entomol. Suisse. 81: 1–8.
Yeargan, K. V. 1979. Parasitism and predation of stink bug eggs in soybean and alfalfa fields. Environ. Entomol. 8: 715–719.
1. Quantify the rate of predation experienced by BMSB nymphs on soybean plants in greenhouse mesocosms
2. Quantify rate of predation of BMSB in the presence of alternate prey
3. Determine the effect of predator presence on soybean yield
Cooperators
Research
General. This research was conducted from November 2016 to May 2017 in greenhouses at the Rutgers Agriculture Research and Extension Center (RAREC) in Bridgeton, NJ. Mesh cages (Bugdorm-2120, MegaView Science, Taiwan) served as mesocosms in this experiment, each containing six soybean plants. This experiment was conducted as a 3 x 3 randomized complete block bioassay (three predator treatments, three herbivore treatments). Treatments were randomly assigned within spatial blocks, with three fully replicated spatial blocks per experiment (temporal blocks), over three experiments for a total of nine replicates per treatment. Experiments started on 5 December, 2016, 7 February, 2017, and 11 April, 2017 and each lasted 21 days. Herbivore treatments included the following: 1) eight 2nd instar H. halys nymphs, 2) eight 2nd instar H. halys nymphs + 75 A. glycines or 3) no herbivores (negative control). These treatments were selected to simulate herbivore abundance at high density, such as that found in untreated organic soybean. The predator treatments consisted of 1) two 3rd-4th instar P. maculiventris 2) two adult Hippodamia convergens (Guerin-Meneville) (Coleoptera: Coccinellidae) or 3) no predator control. During the third temporal block, an additional aphid only treatment was added but, due to space constraints, this treatment was only studied in the absence of predators.
Plants. Soybeans (brand: 34A7, variety: 76347; Blue River Hybrids, Ames, IA, USA) were grown from seeds in 50 well trays in the greenhouses at RAREC until the V2 stage of soybean development (Pedersen and Lauer, 2004). Soybean seedlings were transplanted, three plants per pot, into larger 500 mL pots. Plants were fertilized with Miracle-Gro All Purpose Plant Food (NPK: 24-8-16; Miracle-Gro, Marysville, OH, USA) according to manufacturer specifications immediately after being transplanted and again 7 days thereafter. Pots were randomly assigned to cages, two per cage, and treatments were randomly assigned to cages within each block. During experiments, fertilizer treatment was suspended and only soybeans were grown in the room of the greenhouse where experiments were conducted. Plants were lightly watered every 1-2 days. After watering, the contents of each cage were lightly misted via hose-end sprayer through mesh side panels to provide additional moisture for the insects within.
Insects. Aphids were kept in colony in the greenhouse. The colonized individuals were sustained on trays of young soybean seedlings. As plant health within the colony declined and more winged morphs developed, stems of plants with aphids were cut at soil-level and transferred to new trays of seedlings (Kaser, J. personal comm.). Predators used in this study were acquired from commercial biological control suppliers (H. convergens: Arbico Organics, Oro Valley, AZ, USA; P. maculiventris: Natural Insect Control, Stevensville, ON, Canada). Predators were kept in colony for at least one generation prior to use in experiments to ensure consistent nutritional status. Podisus maculiventris was reared on Galleria mellonella (L.) (O: Lepidoptera, F: Noctuidae) and 10% honey-water solution while H. convergens was sustained on A. glycines from colony. Halyomorpha halys were acquired from a colony kept at RAREC founded originally with individuals from the New Jersey Department of Agriculture’s Philip J. Alampi Beneficial Insects Laboratory.
Sampling Procedure. During each temporal block, metrics of plant growth were assessed prior to prey introduction and twice per week after that. These metrics included a count of the number of trifoliate vertical nodes (omitting the cotyledon and auxiliary bud), and a count of the number of lateral trifoliate buds (those not arising from vertical growth) (Pedersen and Lauer, 2004). Buds at the vertical growing point were considered a single node unless the leaflets of the older node had begun to unfold and were no longer touching the leading bud. Lateral buds were only counted when the leaflets of the developing bud had unfolded enough that they no longer touched one another.
Experiments. Once all transplanted soybean plants had reached at least the V3 stage, an initial sample of plant growth metrics was assessed (mean vertical nodes during initial samples: 3.4 ± 0.16). Next, A. glycines were collected from the colony by removing whole soybean leaves, and aphid abundance was counted under magnification with a compound dissecting scope (Stemi 2000, Zeiss, Oberkochen, Germany). Aphids were gently removed from leaves with a soft tipped paint brush until the total abundance was 75 aphids distributed between two leaves. Preliminary experiments indicated inoculating one plant in each pot with aphids led to a more even distribution of aphid density. Total aphid load (75) was therefore distributed between two leaves so plants in each pot could be inoculated simultaneously. Due to high transfer mortality, A. glycines were given three days to colonize the soybean plants prior to the introduction of other organisms.
Three days after the introduction of aphids, plant growth metrics were assessed again. Eight 2nd instar H. halys were then introduced onto the plants with forceps for herbivore treatments 1 and 2. After 30 minutes, predators were introduced into the appropriate cages. During the course of 21 d experiments, predator and prey abundance was visually monitored and plant health metrics were assessed twice per week. Abundances of H. halys and the predators were counted directly, but aphid abundance was estimated by counting the approximate number of 25-aphid groups per cage. Dead H. halys and predators were removed from the cages at this time. Those in predator treatments may have been killed by predation, and those in no predator control treatments served as checks of environmental mortality. To ensure constant predation pressure, predator abundance was kept constant by replacing dead predators found during the biweekly assessments. Podisus maculiventris nymphs that had molted to adulthood were replaced with appropriate instar nymphs from the colony.
Vertical and lateral buds were often heavily damaged by herbivory. Vertical plant nodes were counted even if both buds at a node had withered or broken off entirely, but side buds were not counted if they appeared dead. Lateral buds were considered dead if their leaves were no longer green and were dry and brittle to the touch. After three weeks, a final assessment of insect abundance and plant growth was conducted. Live predators were not reused between experiments. Any plants that died during the course of the 21 d experiments were cut at the base of the stem, removed from cages and discarded.
Plant Biomass. After final assessments were completed, full plant dry biomass (g) was measured. Soil was removed from roots and plants were transferred to paper bags and stored at -20º C. After at least 24 h in the freezer, plants were desiccated in a 55º C drying oven for 24 h (Jensen and Newsom, 1972) and weighed to the nearest 0.01g (OHAUS Scout Pro, OHAUS Corporation, Parsippany, NJ).
Statistical Analysis. All statistical analysis was conducted in R Studio v3.2.2 “fire safety” (R Development Core Team, 2011). Final plant health metrics and prey abundance counts were checked for assumptions of normality and analyzed for differences between treatments. Due to the possibility of plant death, plant growth metrics were calculated and analyzed per plant. In addition to the number of vertical nodes per plant (NPP), the change in vertical nodes per plant (ΔNPP) was calculated and analyzed for differences between treatments (final NPP – initial NPP). Plants did not have lateral buds at the onset of the experiments, so these data were analyzed only as lateral buds per plant (LBPP). Vertical growth metrics (NPP and ΔNPP) and plant biomass data conformed to assumptions of normality and were analyzed as a 3x3 two-way ANOVA (model: variable = predator × prey treatment + spatial block + temporal block + ε). Prey treatments which did not include stink bugs were omitted from the analysis of stink bug abundance, as was the case for aphids.
Data from the aphid only-no predator treatment, which was only included in the final temporal block, were analyzed separately. To do this, we excluded treatments with predators (since the aphid alone treatment was not tested in the presence of predators) and then analyzed plant metrics from the final temporal block for differences between the four prey treatments (H. halys alone, A glycines alone, H. halys + A. glycines, and no prey control). Analyses were conducted as above but without model terms for predator treatment, which were not included in this analysis (model: variable = prey treatment + spatial block + ε). We then compared aphid abundance alone and in the presence of H. halys. Data that did not meet assumptions of normality were analyzed using generalized linear models with Poisson error distribution and log link function. Where appropriate, analysis of deviance tests were used to determine the statistical significance of model terms, and Tukey’s HSD was used to separate significantly different means (P-values < 0.05 were considered significant).
Results
Plant Growth Characteristics. Development of vertical nodes was significantly impacted by the presence of herbivorous insects. Nodes per plant (NPP) was significantly affected by prey treatments (df = 2, 68, F = 54.17, P < 0.0001) but not predator treatments (df= 2, 68, F = 0.796, P = 0.315) (Fig. 1a). Plants in the no prey-no predator treatment had the most vertical nodes (NPP = 7.7 ± 0.17), while those in both prey-no predator treatment ended with the fewest (5.2 ± 0.11) (Fig. 1a). Plants in the no prey-control cages had the highest NPP (7.6 ± 0.2), while those exposed to both H. halys + A. glycines had the lowest NPP (5.6 ± 0.1) (Fig. 1a). The NPP of plants in the H. halys alone treatment was 6.8 ± 0.1. All pair-wise comparisons between prey treatments were statistically significant. The interaction between predator and prey treatments did not significantly affect NPP (df = 4, 68, F = 2.0, P = 0.10). We detected marginally significant evidence for an interaction between predator and prey treatment effects on NPP (df = 2, 68, F = 2.393, P = 0.053). Values of NPP were significantly different between temporal blocks (df = 2, 68, F = 26.50, P < 0.0001).
Change in Nodes per Plant. The growth of new vertical nodes (described by ΔNPP) was significantly affected by prey treatments (df= 2, 68, F =64.96, P < 0.0001) (Fig. 1b). Plants in the no prey control cages had higher ΔNPP (4.2 ± 0.2) than those exposed to H. halys alone (3.5 ± 0.2) and those exposed to both H. halys and A. glycines (2.2 ± 0.2) (Fig. 1b). All pair-wise comparisons of ΔNPP between prey treatments were statistically significant. Differences in ΔNPP between predator treatments were not statistically significant (df= 2, 68, F = 2.17, P = 0.118), however, there was some evidence for an interaction between predator and prey treatments (df = 4, 68, F = 2.393, P = 0.0531). Values of ΔNPP varied significantly between temporal blocks (df = 2, 68, F = 127.1, P < 0.0001) and spatial blocks (df = 2, 68, F = 4.70, P = 0.0318).
Lateral Buds per Plant. Development of lateral buds (LBPP) was also significantly affected by prey treatments (df = 2, 76, F = 28.39, P < 0.0001) (Fig. 1c). Plants exposed to H. halys and A. glycines together produced significantly fewer SBPP (1.4 ± 0.2) than those exposed to H. halys alone (3.0 ± 0.5) or those in the no prey control (3.7 ± 0.5). Differences in LBPP between the H. halys alone treatment and the no prey control were not statistically significant. Predator treatments did not have a significant effect on LBPP (df = 2, 78, F =1.17 P = 0.31) (Fig. 1c). Interactions between predator and prey treatments showed a marginally significant effect on LBPP (df = 4, 68, F = 2.08, P = 0.079). Similarly, LBPP was affected by spatial blocking but this was only marginally significant (df = 2, 72, F = 3.57, P = 0.058).
Plant Biomass. Average plant biomass was significantly affected by prey treatment (df = 2, 68, F = 24.51, P < 0.0001) and predator treatment (df = 2, 68, F = 5.94, P = 0.004) (Fig. 1d). Mass of plants exposed to H. halys alone (1.906g ± 0.140g) was significantly lower than that in no prey control cages (2.276g ± 0.169g) (Fig. 1d). However, the mass of plants exposed to H. halys + A. glycines (1.424g ± 0.132g) was significantly lower than each of these. Averaged across all prey treatment, plants grown without predators had the lowest average mass (1.628g ± 0.164g); significantly lower than that of H. convergens (2.013g ± 0.162g) or P. maculiventris (1.967g ± 0.152g) treated plants. Predator-prey treatment interaction did not significantly affect plant mass (df = 4, 68, F = 1.62, P = 0.177), nor did spatial blocking (df = 2, 68, F = 1.62, P = 0.151). However, differences in plant mass between temporal blocks were statistically significant (df = 2, 68, F = 139.55, P < 0.0001).
Figure 1. The Effect of Predator and Prey Treatments on Plant Growth Characteristics.
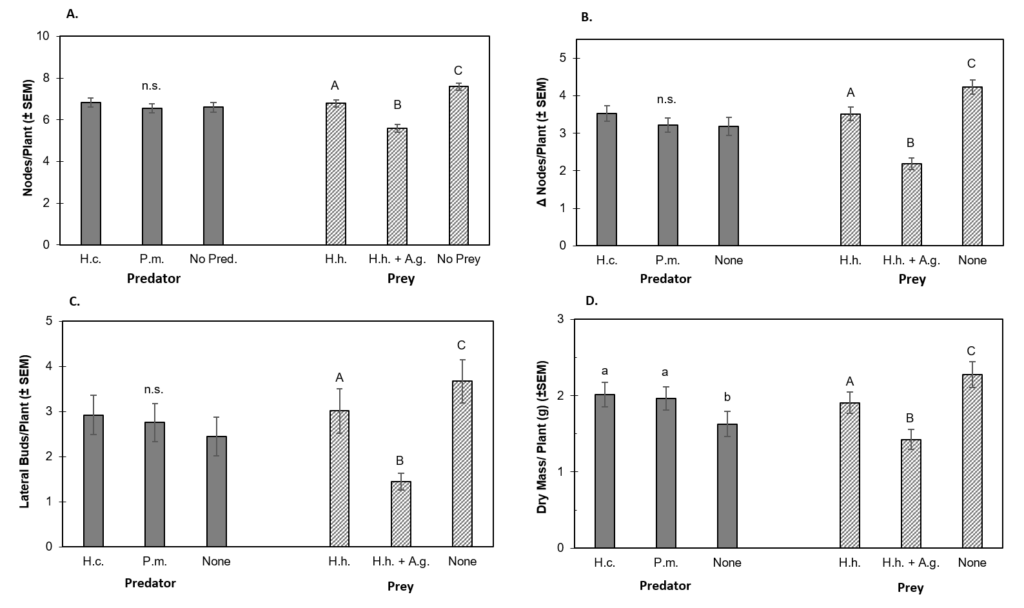
Prey Abundance. The abundance of H. halys was significantly higher in the presence of A. glycines (df = 1, 50, F = 33.01, P < 0.0001). On average, 3.0 ± 0.4 H. halys nymphs survived the 21 d experiments in the presence of aphids, while only 0.5 ± 0.1 survived in treatments without A. glycines. More H. halys nymphs survived in the no predator control (2.3 ± 0.5) than those caged with P. maculiventris (1.0 ± 0.3) or H. convergens (1.7 ± 0.4), although the latter contrast was not statistically significant (Fig. 2). Podisus maculiventris was observed feeding on H. halys during the course of the experiments, but H. convergens was not. Effects of predator-prey treatment interaction (df = 2, 44, F = 0.388, P = 0.678) and spatial block (df = 1, 46, F = 0.973, P = 0.378) were not statistically significant.
Figure 2. The Effect of Predator and Prey Treatments on Final Mean (±SEM) H. halys Abundance.
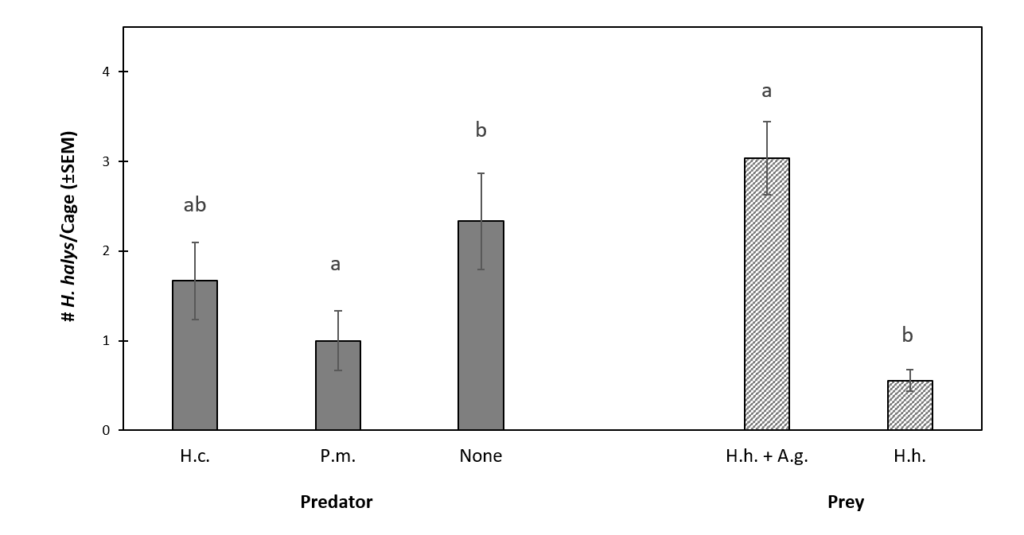
Final aphid abundance was significantly affected by predator treatments (df = 2, 27, F = 1242.7, P < 0.0001) (Fig. 3). Significantly higher A. glycines abundance was observed in the no predator control (386.1 ± 65.8) than either predator treatment. Aphid abundance in cages with H. convergens (63.9 ± 36.1) was significantly lower than those with P. maculiventris (313.9 ± 46.8). Both predator species were observed feeding on A. glycines during the experiments. Unlike H. halys presence, which declined throughout experiments, A. glycines continued reproduction and final populations were often considerably higher than initial.
Figure 3. The Effect of Predator and Prey Treatments on Final Mean (± SEM) A. glycines Abundance.
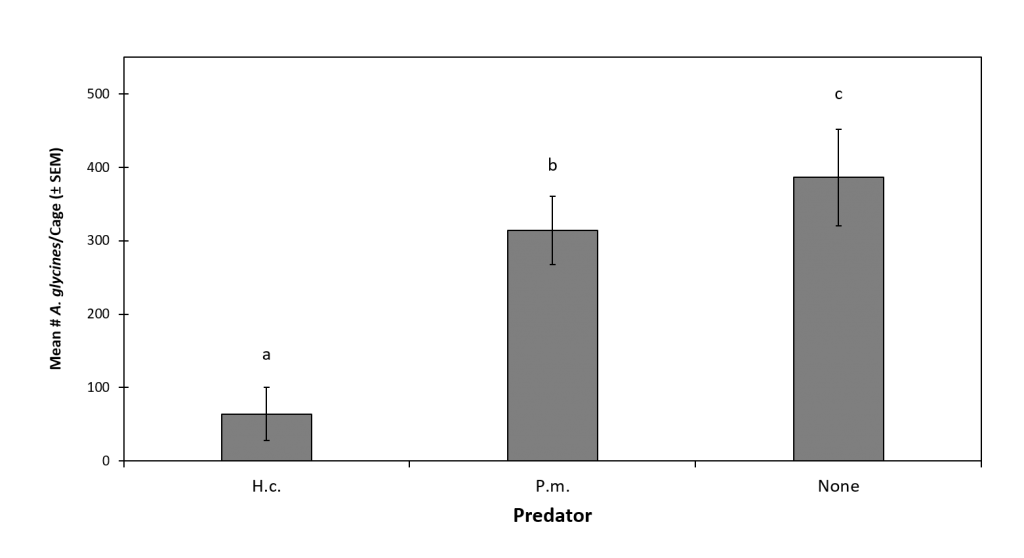
Plant Health Impacts of A. glycines. The effect of A. glycines without additional prey or predator was only evaluated during the final temporal block. When data from this block were separately analyzed, prey treatments significantly affected NPP (df = 3, 19, F = 32.89, P < 0.0001), ΔNPP (df = 3, 19, F = 31.7, P < 0.0001), SBPP (df = 3, 23, F = 2.83, P = 0.037) and plant mass (df = 3, 19, F = 446.5, P < 0.0001). Plants grown in the no prey control treatment had significantly higher NPP (8.8 ± 0.1) than those with H. halys alone (7.4 ± 0.2), or A. glycines alone (6.6 ± 0.2) (Fig. 4). The lowest NPP was observed on plants grown with both prey species together (5.3 ± 0.4); significantly lower than all other prey treatments. The NPP of plants grown with H. halys alone or A. glycines alone were not significantly different. Similarly, plants grown without prey had significantly higher ΔNPP (5.8 ± 0.1) than those with H. halys alone (4.6 ± 0.2), A. glycines alone (3.9 ± 0.2) (Fig. 4). Plants exposed to both prey species had significantly lower ΔNPP (2.3 ± 0.4) than all other prey treatments. The ΔNPP of plants grown with H. halys alone or A. glycines alone were not significantly different. There were no significant differences in LBPP between prey treatments during pair-wise comparison (Fig. 4). Plants in the no prey control had significantly higher mass (2.852g ± 0.169 g) than those exposed to H. halys alone (2.487 g ± 0.140 g) (Fig. 4). Plant mass did not vary significantly between the A. glycines alone treatment (1.108 g ± 0.117 g) and the H. halys + A. glycines treatment (0.988 g ± 0.132 g), but each of these was significantly lower than that of the no prey control and the H. halys alone treatment (Fig. 4).
Figure 4. The Effect of Exposure to A. glycines on Metrics of Soybean Health.
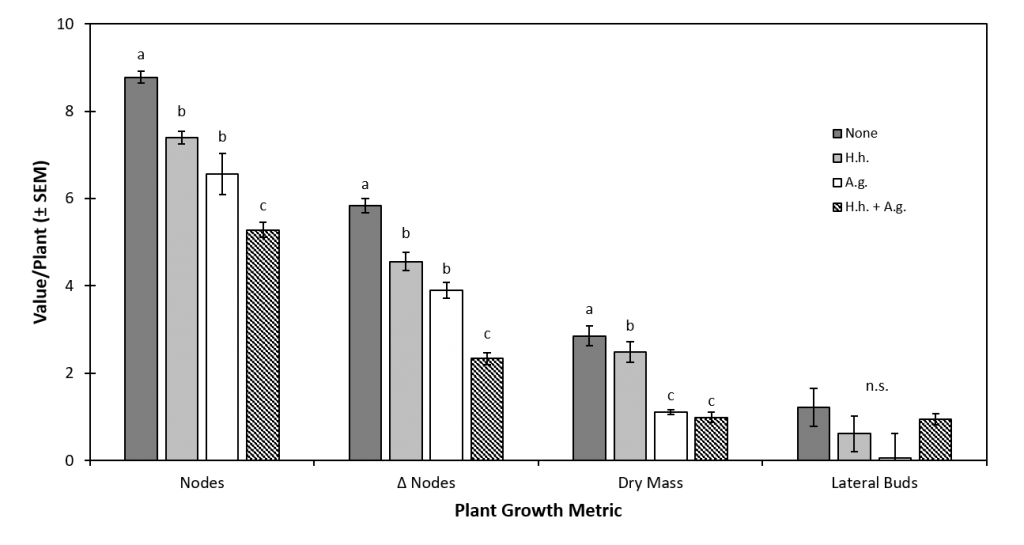
Discussion
To determine the realized predation of H. halys nymphs on soybean, we performed greenhouse mesocosm experiments using A. glycines as an alternate prey. The findings of this study indicate that although predators were able to significantly decrease prey populations, plant growth and development were still strongly impacted by prey treatments. Predator treatments significantly affected average plant mass, but not metrics of vertical or lateral growth. Similarly, the presence of H. halys significantly affected NPP, ΔNPP, and plant biomass, but our results have demonstrated that aphid presence was a more significant factor in determining final plant health. Among the treatments that included only H. halys as the prey item, none of the plant growth metrics evaluated indicated a top-down effect by the predator. Although plant growth metrics in treatments with H. halys alone were significantly lower than those in the no prey control, these metrics were consistently and significantly lower in treatments that included A. glycines.
The cause of low H. halys survivorship in no predator control treatments is currently unknown but may be due to the effect of the experimental environment. Averaged across all prey treatments, H. halys final survivorship in no predator control treatments was relatively low given that Glycine max is a known H. halys host plant (Owens et al., 2013; Venugopal et al., 2015, 2014). However, other stink bug species are known to develop poorly on pre-pod set soybeans, possibly due to the lack of nitrogen rich reproductive structures (Stam et al., 1987) and H. halys does not colonize soybean until the R3-R4 stage (Nielsen et al. 2011) so poor the survivorship observed could be due to a nutritional mismatch. Halyomorpha halys survivorship also differed between temporal blocks, which may indicate significant differences in greenhouse conditions over time. Temperature data collected within the greenhouse showed that average temperature increased slightly between each successive block, but during the final replicate temperatures exceed 33º C on three non-consecutive days. At this temperature H. halys generational mortality is 95% (Nielsen et al., 2008). The final abundance of H. halys in this final temporal block were nearly half that observed in the first temporal block.
The results of this study indicated weak interactions between the selected predator and prey treatments. Weak interactions between predator and prey treatments are likely the result of unpredicted predator preferences. Hippodamia convergens did not significantly affect the survivorship of H. halys 2nd instars in bench-top predation bioassays, but this species is a predator of A. glycines (Rutledge et al., 2004, Pote and Nielsen, in press). Conversely, P. maculiventris is a predator of H. halys nymphs (Pote and Nielsen, in press) but was not expected to consume A. glycines. These predator species were intentionally selected to help clarify how predators respond to H. halys in the presence of a preferred secondary prey species, and how secondary prey species are affected by the presence of predators which prefer H. halys. However, the lack of a strong interactive effect on plant growth metrics may be the result of unexpected A. glycines predation by P. maculiventris. Final aphid abundance was significantly lower in the presence of P. maculiventris compared to no predator controls. The magnitude of this population reduction may have been insufficient to protect soybean plant development as the presence of P. maculiventris did not significantly improve plant growth metrics over no predator controls (with the exception of plant mass). Increased replication may have clarified the effect of interacting predator and prey treatments.
The inclusion of an aphid only-no predator treatment during the final temporal block allowed for the direct comparison of H. halys feeding damage to that of A. glycines. Metrics of vertical and lateral growth were not statistically different between the aphid only and stink bug only treatments, but these treatments did differ significantly in their impact on plant mass. The average mass of plants grown with only H. halys nymphs was more than double that of plants grown with only A. glycines. Furthermore, plant mass was not significantly different between the aphid only prey treatment, and that which included both prey items. These findings further reinforce the conclusion that A. glycines feeding was significantly more damaging to plant health and development than that of H. halys.
The relative impacts of A. glycines and H. halys on soybean seedlings may vary widely from their impacts on mature plants with developing reproductive tissues. The rapid proliferation of A. glycines observed in this study may be diminished on mature plants with greater energy reserves to dedicate to defense, while relatively little stink bug feeding can dramatically affect the quantity and quality of developing soybean seedpods (Jensen and Newsom, 1972; Owens et al., 2013). Plant age may have also affected the survival of H. halys nymphs on soybean plants. Despite the fact that soybean is a known H. halys host plant (Owens et al., 2013; Venugopal et al., 2014), relatively few H. halys nymphs survived the course of the 21 d experiments. However, H. halys only infests soybean fields once bean pods or other reproductive tissues are present on plants (Nielsen et al., 2011; Venugopal et al., 2015, 2014) thus pre-reproductive G. max, like those used in this study, may be a sub-optimal H. halys host plant. Additional experimentation is recommended to clarify the effect of plant age on the predator-prey interactions discussed here.
Unexpectedly, the presence of A. glycines significantly increased the survivorship of H. halys regardless of predator treatment. Fewer nymphs survived in the H. halys alone-no predator treatment combination (0.77 ± 0.5) than those deployed with A. glycines and P. maculiventris (1.77 ± 0.5), a known H. halys predator which was repeatedly observed feeding on H. halys nymphs in this study. The cause of this phenomenon is currently unclear. Aphid feeding is known to alter plant defensive chemistry and photosynthetic physiology (Bell et al., 1995; Macedo et al., 2003), either of which could affect the survivorship of H. halys nymphs. Nutritional analysis of plants from the 2nd and 3rd temporal blocks is currently underway to determine if prey treatments affected the concentrations of nutrients that may influence H. halys survival. Plants exposed to treatments including A. glycines were often coated in honeydew which may have been consumed by H. halys nymphs, extending their lifespans. Interestingly, the increased survivorship experienced by H. halys in the presence of A. glycines was mitigated somewhat by the presence of H. convergens, a predator of A. glycines (Rutledge et al., 2004). The average final abundance of H. halys in both prey-no predator treatments was significantly lower than that of treatments with both prey and H. convergens. Hippodamia convergens may reduce H. halys survivorship by reducing aphid abundance thereby reducing the magnitude of the beneficial presence of A. glycines, or H. convergens may impact stink bug survival through direct predation or sub-lethal effects. Regardless of the cause, facilitative interactions between H. halys and A. glycines could be important for the ecology and management of these pests. As the H. halys invasion continues into the major soybean growing regions of the US, understanding the relationship between H. halys, A. glycines and their shared natural enemies may become a key concern for growers and researchers.
Due to the aforementioned issues with this experiment, it is difficult to interpret the results in a meaningful way. It is clear that A. glycines is capable of causing significantly more damage to developing soybean plants than is H. halys. However, the speed at which A. glycines reproduces makes this comparison questionable: stink bug numbers stayed constant or declined as the experiment did not last long enough to allow for H. halys reproduction, while A. glycines was able to reproduce et infinitum on its preferred host plant. However, this experiment does reinforce our understanding of the ability of generalist predators to consume H. halys in field-like conditions, with Podisus maculiventris feeding on H. halys nymphs occurring in the majority of cages where these species were exposed to one another. Conversely, these results reinforce earlier findings by the authors which show that Coccinellidae, despite constituting a large proportion of predatory invertebrates in soybeans, do not readily accept H. halys as prey in this crop. Unfortunately, the effects of A. glycines presence on soybeans overshadowed treatment effects from predators and H. halys presence, so the rate of attack on H. halys by generalist predators in the presence of alternate prey remains unclear.
Education & Outreach Activities and Participation Summary
Participation Summary:
As stated in other sections of this report, the results of the experiments funded by this grant were not conducive to the creation and dissemination educational materials. Unfortunate and unforeseeable treatment effects have obfuscated the primary goals of this research which would otherwise have been of interest to growers and agricultural researchers. Due to the lack of clear results, educational material originally planned as part of this grant has been cancelled. Additional replication of this work may clarify the role of alternate prey in H. halys population dynamics and help to justify the importance of natural enemies in the management of this important pest. Finally, further research is needed to understand the apparent facilitation between H. halys and A. glycines observed during this experiment. Despite the lack of clear results toward the primary goals, this behavior may not be limited to greenhouse environments and may therefore be of great interest to growers of soybeans affected by A. glycines and/or H. halys. However, because the cause of the apparent facilitation is unknown and has been observed only once, this information alone is of limited applicability and usefulness to growers and researchers.
Project Outcomes
Unfortunately, unforseen interactions have muddled the results of this study limiting their application to growers and to agricultural sustainability in soybeans and other agronomic crops. Although predators are an important source of Aphis glycines mortality in soybeans, the extreme herbivory exerted by this pest overshadowed the experimental effects of the presence of H. halys as well as the presence of predators. Essentially, these findings reinforce the importance of A. glycines as a pest of soybeans; most soybean growers are intimately aware of this fact. Due to the magnitude of A. glycines feeding damage on young soybean plants, its impossible to draw conclusions about the apparent preferences of H. convergens and P. maculiventris for aphids or stinkbug nymphs. It is similarly difficult to understand how these predators might prevent damage to plant growth or yield. Experimental issues leading to these issues will be discussed in the following section.
Despite these difficulties, growers may benefit from understanding how valuable the background levels of predation exerted by natural enemy communities in soybean truly are. Polyphagous predators, like Coccinellidae, are often under-appreciated as natural enemies due to their generalist nature, but my results show that the ability of generalists to persist on non-target prey allows them to "lie-in-wait" within agro-ecosystems until the arrival of the primarily damaging prey. These results also show how damaging A. glycines feeding can be on young plants, which are likely protected from this pest by natural enemies in on-farm settings. Aphid populations are generally not at damaging levels until the plants are large enough to sustain some feeding damage without adverse affects on plant health or yield. Finally, these results reinforce an important idea within integrated pest management: there is no cure-all tactic for controlling all pests. Although predation is an important driver of A. glycines population dynamics, in this experiment, natural mortality was a more important driver of H. halys population dynamics.
Throughout the course of this study, I learned a great deal about the pest status of H. halys in relation to Aphis glycines in soybean crops and how these pests interact in the presence of predators. Regardless of predator treatments, plant growth-related response variables were primarily driven by the presence of A. glycines. Although Hippodamia convergens adults, one of the included predator treatments, regularly fed on aphid populations during the experiment, the growth rate of aphid populations was generally higher than the rate of aphid removal by lady beetles. By the conclusion of the 21 day experiments, aphids were often absent from cages due to high rates of predation, but this predation apparently occurred too late to protect the developing soybean plants as many showed signs of stunted growth. Podisus maculiventris, a predator of H. halys, did consume its target prey throughout the study, but the magnitude of H. halys herbivory was greatly overshadowed by the herbivory potential of Aphis glycines.
These results have highlighted several important characteristics of H. halys biology, including it's role as an herbivore of reproductive or fruiting structures and not of primarily vegetative plant tissue. Halyomorpha halys survivorship was low, even in treatments which did not include predators, indicating pre-reproductive soybean (like that used in this experiment) is a sub-ideal host for H. halys. We know that H. halys follows the phenology of host plants in the field, preferring to feed on fruiting/reproductive tissues, but I had previously assumed development on preferred hosts was still possible in the absence of these tissues. This is clearly not the case.
Additionally, I learned a great deal about the applicability of biological control of herbivorous pests. Based on the tenants of ecological population dynamics, I assumed predators could play an important role in limiting feeding damage from all herbivores, but the results of this study showed that only certain pests, like aphids, can be adequate controlled by predators under realistic conditions. Although prior research of mine has shown that predators will accept H. halys as prey, this study showed that biological control works best against small, fast reproducing pests with very few defensive adaptations like aphids, and is less effective against larger, defended prey like stinkbugs.
Finally, the most interesting result of this study was the revelation of an interesting yet unexplained interaction between A. glycines and H. halys. As previously mentioned, H. halys survivorship on pre-reproductive soybean was very low. However, this effect was negated by the presence of A. glycines. Fewer nymphs survived in the H. halys alone-no predator treatment combination (0.77 ± 0.5) than those deployed with A. glycines and P. maculiventris (1.77 ± 0.5), a known H. halys predator which was repeatedly observed feeding on H. halys nymphs in this study. Several hypotheses have been suggested to explain this phenomenon: increased humidity from A. glycines honeydew increased H. halys survivorship, A. glycines feeding altered plant chemistry and/or plant defenses to allow successful feeding and development by H. halys, or H. halys may have utilized A. glycines as an alternate food source comprised of higher nitrogen content than the non-reproductive soybean tissue. Although the cause of this interaction is unknown, implications for soybean growing regions of the U.S. are clear. If A. glycines presence facilitates H. halys development, the latter could become a significantly more important pest of soybeans where the ranges of these pests overlap (much of the soybean growing regions of the Upper Midwest).
I have included the research funded by this grant in a chapter of my doctoral dissertation and have subsequently graduated and accepted a position as a post-doctoral researcher at Michigan State University. As a result of experienced gained during my work on this grant, I was uniquely qualified for the position I currently occupy. Not only is H. halys an important pest in the Midwest, where I now work, but my experience soybeans and Aphis glycines during this project helped familiarize me with one of the most important crops in my new home range and gave me invaluable experience working with Aphis glycines in colony (as I had never worked with aphids in colony before). During the course of this research, I worked closely with a post-doctoral researcher in my previous lab (Dr. Joseph Kaser) who studied Aphis glycines and its parasitoids during his doctoral studies. As a result of this collaboration, Dr. Kaser and I have plans to collaborate on projects related to the H. halys invasion of the Midwest and the recent discovery of Trissolcus japonicus, an invasive parasitoid of H. halys.
Although certain findings yielded from this research have objective value to researchers and growers, the primary objective of the experiment was unable to be addressed due to unforseen challenges during the experiment. There are a number of experimental design choices made during the conduct of this experiment which may have negatively effected the quality of the results. I will discuss these here.
Preliminary experiments were conducted to test the ability of H. halys nymphs to survive on greenhouse-grown soybean. The results of these experiments showed a very high survival rate (>85%) in the absence of predators. However, the soybeans used in this test were first grown under natural light outdoors in the fall and were flowering and beginning to develop seed pods. When soybean plants were grown entirely in greenhouse settings during the early winter, they rarely developed reproductive or fruiting structures and those plants that did were very large, completely filling the BugDorm tents used as mesocosms in this experiment. As a result, accurately assessing the populations of insects within these cages was impossible so I decided to use younger, pre-reproductive plants for all replicates in this experiment. Unfortunately, the results of this study revealed that H. halys nymphs survive very poorly on vegetative soybeans. Future experiments of this nature should ideally be conducted on field-grown reproductively mature soybean plants grown in-situ.
Other issues affecting the success of the experiment are related to the difficulty of establishing new colonies of insects. I had planned to conduct this experiment from November through February when conditions in the greenhouses could be assumed to be relatively constant. However, due to issues with establishing colonies of two species of predators, one new species of prey (H. halys was already in colony in our lab) and attempting to time the health of these colonies with the correct developmental stage of soybean plants was extremely difficult. As a result, the time between replicates was necessarily increased to get the timing of colonies and plants synchronized, leading to experiments continuing on into early May, when temperatures in the greenhouses became hot enough to affect the experimental results. In the future, adequate time should be given to order, ship, and receive commercial insects as well as time for the establishment of colonies and potentially time to re-order new commercial propagules if necessary. Similarly, my grant proposal originally called for estimating yield and soybean pod health at the conclusion of this experiment, but given the issues with growing reproductively mature plants in the greenhouse, this was also not possible.
Finally, the choice of predator species and alternate prey items was unfortunately impacted by species that were available commercially or in the field at the time this grant was accepted. Due to the start date occurring in early Fall, the species which were considered ideal candidates for the goals of this grant were unavailable. Namely, the difficulty of starting colonies of damselbugs (F: Nabidae), and native stink bugs detrimentally impacted the nature of this experiment. Damselbugs would have been a preferred predator treatment over H. convergens due to their acceptance of H. halys nymphs in laboratory predation studies, and native stink bugs would have been preferred over Aphis glycines, as native stink bugs have similar anatomy, behaviors and physiology to H. halys. However, these organisms were not available commercially or in sufficient quantities in the wild to start self-propagating colonies in time for the conduct of this experiment. Externalities of this type are unfortunately common in entomological research, so I selected the best candidates given the predators and prey species available. However, the substitution of prey species ended up being a critically unfortunate one as Aphis glycines are voracious herbivores of young soybean plants, while H. halys is less so. In an ideal world, unconstrained by commercial availability and incipient graduation deadlines, this experiment would have been conducted using the ideal predators and prey, first described in the grant proposal. However, I believe the changes to the experimental design were still in-line with the goals of the project and included the best options available given the aforementioned limitations.