Final report for GNE17-155
Project Information
- The purpose of this project is to investigate root-microbe interactions to aid development of sustainable management strategies of soil-borne diseases such as apple replant disease (ARD). One of the greatest challenges facing apple production in the Northeast and worldwide is the development of sustainable and economically viable ARD management strategies. Sustainable and environmentally friendly strategies are needed to encourage the growth of healthy and efficient roots. These strategies should enhance root interactions with beneficial fungi and inhibit infection of fungal pathogens. Improved management, however, can only be accomplished through greater understanding of root-microbe interactions at the individual root level.
- This project aimed to enhance root production and interactions of beneficial mycorrhizal fungi with individual roots at an apple replant site by applying localized nutrient-N sources and enhancing nutrient foraging of both roots and mycorrhizal fungi. This approach was unique as it used root boxes to observe newly planted trees.
- Trees in the grass row grew more roots than trees in the tree row. Trees in the grass row also produced more slow-growing roots than fast growing roots. Nutrient addition did not effect root growth, and roots may have been more reliant on their own roots to enhance nutrient uptake over symbioses with mycorrhizal fungi.
Objective 1) Determine the effect of nitrogen (N) source and location at an apple replant site on root proliferation in young 'Golden Delicious' apple trees on M.26 rootstock. Expected outcome: Trees with localized addition of the readily available N source, urea, will have more root proliferation than trees with the slow-release organic N addition and the control (water) treatment. Trees grown in the grass row middle where perennial grasses were previously grown will have more root proliferation than trees grown in the herbicide strip where mature apple trees were previously grown.
Objective 2) Determine the effect of nitrogen (N) source on growth rate, duration of growth and colonization of individual apple roots by beneficial fungi and pathogenic fungi implicated in apple replant disease (ARD). Expected outcome: Compared to the organic N and control treatments, trees with localized urea addition will have more fast-growing roots that grow for a longer duration. Colonization by both arbuscular mycorrhizal fungi (AMF) and non-mycorrhizal fungi (NMF) will be moderate. Trees with the slow-release organic N addition will have fewer fast-growing roots with greater AMF colonization and minimal NMF infection. Trees with the control treatment will have more slow-growing roots and higher NMF infection.
Objective 3) Determine the effects of location at an apple replant site on growth rate, duration of growth and colonization by AMF and NMF of individual roots. Expected outcome: Trees planted in the herbicide strip where soil-microbial communities developed in association with apple trees will have more slow-growing roots with higher NMF infection and more putative soil pathogens. Trees grown in the grass row middle where apple trees had little influence on development of soil-microbial communities will have more fast-growing roots, higher AMF colonization and fewer putative pathogens.
Objective 4) Determine the effect of root growth rate on root interactions with AMF and NMF. Expected outcome: Fast-growing roots will be rapidly colonized by AMF compared to slow-growing roots which will be uncolonized or colonized by NMF. The presence of AMF in roots will minimize the likelihood of NMF infection in young absorptive roots. These trends are expected across location and nutrient treatment.
Objective 5) Prepare results for academic and extension audiences at academic meetings and multi-state extension events such as Penn State field days and the Mid-Atlantic Fruit and Vegetable Convention. Results will also be disseminated through written extension materials supported by Penn State as described in the outreach plan.
Problem: The purpose of this project is to investigate root-microbe interactions to aid development of sustainable management strategies of soil-borne diseases such as apple replant disease (ARD). One of the greatest challenges facing apple production in the Northeast and worldwide is the development of sustainable and economically viable ARD management strategies. Due to the lack of suitable orchard sites, existing sites are continuously planted with apple. This leads to a buildup of soil-borne fungal pathogens such as Pythium, Fusarium, Cylindrocarpon and Rhizoctonia. Pathogenic fungi target the absorptive roots needed for water and nutrient uptake leading to root death, stunted tree growth and tree mortality.
Historically, ARD management strategies have targeted soil-borne pathogens by sterilizing soil with chemical fumigants or biofumigants. In the Northeast, growers commonly manage ARD through crop rotation or biofumigation. However, these management strategies are associated with high economic costs. For example, growers lose income when their land is taken out of fruit production for pre-plant treatments. Growers also make a substantial monetary investment to plant new trees, particularly in high-density apple orchards (1,000 trees per acre). In addition, crop rotations do not always manage ARD effectively. Therefore, if pre-plant strategies are ineffective, establishment and development of young trees may be delayed or trees may die. This results in further loss of income and additional establishment costs. Moreover, while biofumigation effectively suppresses pathogen populations, it also suppresses beneficial microbes.
Sustainable and economically viable management strategies are needed for both conventional and organic apple production to manage ARD. However, there is little understanding of the interactions between apple roots of young trees, beneficial fungi such as arbuscular mycorrhizal fungi (AMF) and pathogenic fungi implicated in ARD. Growers need sustainable and effective management strategies to grow healthy roots and encourage root interactions with AMF. If beneficial microbes can be enhanced, perhaps by the use of organic fertilizers, roots may be less susceptible to pathogen infection at planting and through the life of the orchard.
Justification and Rationale: Traditionally, ARD research focused on identifying associated pathogens, breeding tolerant rootstocks and identifying effective soil fumigation treatments. These research efforts were not designed to determine why certain physiological and environmental factors in a field setting are associated with ARD development. This knowledge gap has led to an increased dependence on fumigation for ARD management and economic loss where pre-plant treatments are ineffective. Sustainable and environmentally friendly strategies are needed to encourage the growth of healthy and efficient roots. These strategies should enhance root interactions with beneficial fungi and inhibit infection of fungal pathogens. Improved management, however, can only be accomplished through greater understanding of root-microbe interactions at the individual root level. For example, in a study of absorptive roots in apple, growth rates of individual young roots influenced the type of fungi colonizing those roots (12). Fast-growing roots were colonized by AMF as soon as three days after emergence. In contrast, slow-growing roots remained uncolonized or were colonized by non-mycorrhizal fungi (NMF) after nine days. In addition, once a root was colonized by AMF or NMF, it was heavily colonized by that fungus alone. This is notable because it suggests that the presence of AMF may inhibit infection of NMF in young, active, apple roots. Only roots older than 25 days were colonized by both AMF and NMF (12).
In an established apple orchard, I investigated the effects of fruiting (fruit vs. no fruit) and localized-nutrient addition of various nitrogen (N) sources (mineral N, organic N, and water) on root growth and colonization by AMF. Results from this study indicated that for fruiting trees, roots growing in mineral N had the highest root length colonized by AMF. In contrast to mineral N addition, roots growing in organic N had the lowest AMF colonization (P=0.05) in fruiting trees. However, results indicated that more AM hyphae grew in soil surrounding roots in the organic N treatment when trees had fruit (P=0.05). This suggests that roots in organic N may use extramatrical hyphae of AMF to help take up nutrients.
The purpose of this project is to investigate the effect of nitrogen source and location at an apple replant field site on root proliferation, and at the individual root level, growth rate, duration of growth and colonization by AMF and replant pathogens. For this study, I will sample young roots (< 21 days old) because they actively respond to environmental factors such as N addition. In fruit crops, roots older than 21 days generally exhibit low activity for nutrient uptake (3, 13 ). I will also use a novel approach of sampling individual roots to investigate possible consequences of N source on roots of apple trees planted at an apple replant site. I will compare roots of two populations, fast- vserus slow-growing roots, to characterize root interactions with AMF and fungal pathogens implicated in ARD.
Research
Field site and experimental design. The field site was located at the Russell E. Larson Agricultural Research Center in Rock Springs, Pennsylvania, USA (40.8°N and 77.9°W, elev. 350 m). This site was chosen because apple trees had previously been planted on this site since 1999. On April 3, 2017, 20 root boxes (root observation windows) were installed at the replant site. Ten root boxes were installed in the grass row middle which was strip killed in the fall of 2016 (Fig. 1a). Ten root boxes were also installed in the herbicide strip (tree row). Root boxes were constructed as described by Comas & Eissenstat (2000). Root box dimensions are 0.7 x 0.7 x 0.3 m. After root box installation, holes were dug to plant trees using a backhoe and auger attachment. Differences in soil structure and characteristics between the grass row and tree row were observed at planting (Fig. 1b). Soil was collected to assess soil health, and samples were sent to the Cornell Soil Health Laboratory (Cornell University, Ithaca, NU). The experiment used ‘Golden Delicious’ apple trees on M.26 rootstock (Adams County Nursery Inc.; Aspers, PA). On April 27, 2017, 80 apple trees were planted at 0.45 x 6.1 m spacing. Rows were oriented in a north-south direction, trees were free standing. After planting, the central leader of each tree was pruned before leaf out. During bloom, flowers on all trees were removed on May 22, 2017. Soil at the study site was in the Hagerstown series (mesic Typic Hapludalf) with a pH of 7.1 and an organic matter content of 1.7% in the top 0.25 m of the soil profile. Trees were not fertilized at planting.
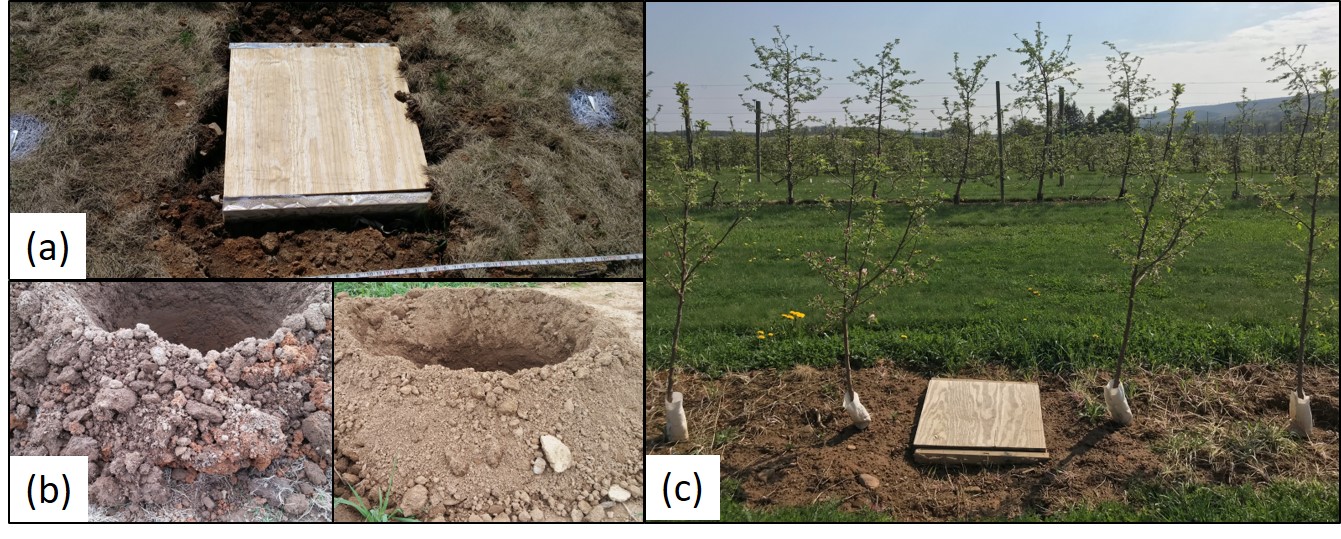
The experimental design consisted of two replant locations (grass row middle and herbicide strip) and three localized nitrogen (N) patches (inorganic N, organic N, and unfertilized) with 10 replications each. Apple trees were divided into 20 experimental units of four trees each. Experimental units included one root box with two trees on both the north and south side of the root box (Fig. 1c). The two outer trees were used as guard trees, and the two middle trees were treatment trees. Treatment trees were planted 30 cm from the root box window. Root boxes had two viewing windows to allow observation of and access to newly produced roots. One window was on the north side and a second window was on the south side of the root box, each facing a different apple tree. Each viewing window was further divided into two smaller windows that were 0.5-m long and 0.3-m deep (4 smaller windows per root box). Smaller windows were made of 3-µm thick sheets of clear acetate film (United State Plastic Corporation; Lima, Ohio) to permit root observation and access for root excision. Soil was collected during root box installment, sieved with a 1-mm screen, and used to fill in gaps between windows and the undisturbed soil. To minimize temperature fluctuations within the root box, 2.5-cm thick removable Styrofoam® was placed against each window when not in use. A removable wooden lid was also placed over each root box after installation to exclude sunlight and rain.
In year one of this study, on September 15, 2017, fine roots first appeared against the observation windows. Following root appearance, one of the three N treatments was applied to each window section. For the unfertilized treatment, two viewing window sections were treated per root box. One window section for each side of the root box that faced a tree was treated so that both treatment trees in the experimental unit had an unfertilized control. Twenty ml of water were applied weekly at the soil-acetate film interface. Water was applied across the top of the window at four locations using a pipette. Organic or inorganic N treatments were randomly assigned to the remaining window sections in each root box. For the organic-N treatment, fully expanded green leaves were randomly collected on May 20, 2017 from non-experimental mature ‘Golden Delicious’ apple trees on M.26. Mature trees that were planted in 1997 at an adjacent field site at the Russell E. Larson Agricultural Research Center in Rock Springs, Pennsylvania, USA. Leaves were dried at 70˚C for five days in a drying oven and ground using a ball mill. At the time of application, four 5-mm soil cores were taken to a depth of 10 cm along the soil-acetate film interface of the treatment window. For each treatment window, 5 g of ground leaves were divided and funneled down the holes of the four cores, and soil was back-filled over the ground leaves. A subsample of the leaves was analyzed for total nutrient content using an elemental analyzer (Vario MAX cube, Elementar; Langenselbold, Germany; Horneck & Miller, 1998). Gradual release into soil of about 20% of total N contained in ground leaf material (2.2% N) was predicted from May to October in 2013 (Moore et al., 2006). For the inorganic-N treatment, 20 ml of urea in water at 70 ppm in water were applied weekly in the third window section to simulate the concentration of N released by ground apple leaves. Urea was applied across the top of the window at four locations using a pipette.
Percent soil moisture and soil temperature were measured biweekly at depths of 15 and 30 cm in each root box using a 10-cm long time domain reflectometry waveguide (TDR 100; Campbell Scientific Inc.; Logan, UT) and a HH21 microprocessor-based thermometer (OMEGA Engineering, Inc.; Stamford, CT), respectively. The waveguide and thermometer were inserted horizontally and perpendicular to the treatment windows. Soil solution was collected weekly in the treatment window sections of 10 root boxes using 5-cm-long micro-lysimeters (Rhizon soil moisture samplers, Eijkelkamp Agrisearch Equipment; Giesbeek, The Netherlands; Fig. 2a). This solution was sampled 24 h after urea application and stored in vials at -20˚C until further analysis. Total N content of soil solution samples was analyzed (Brookside Laboratories Inc.; New Bremen, OH).
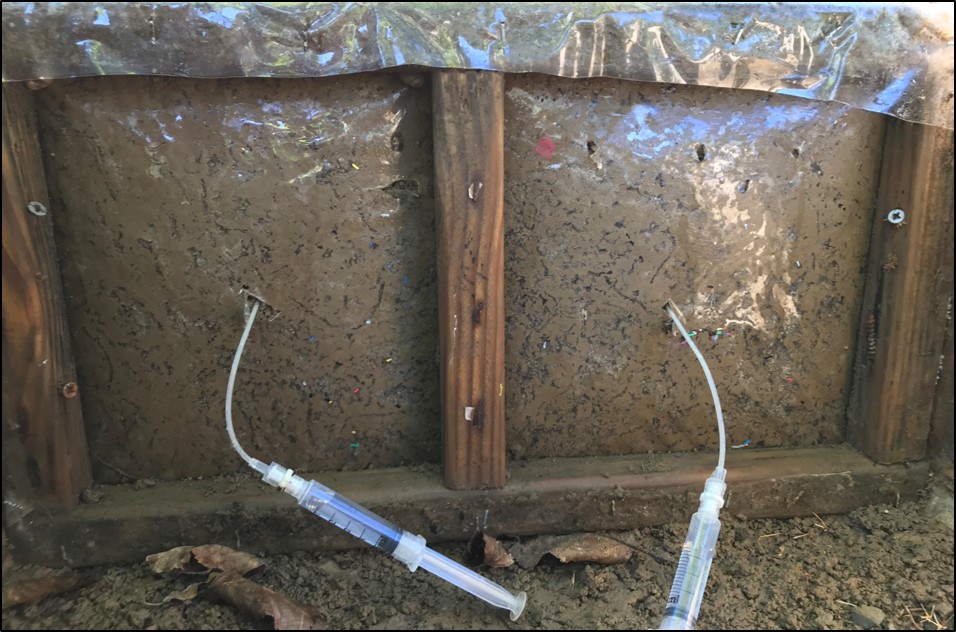
Due to the limited number of roots produced in year one of this study, this experiment was extended and repeated a second year in 2018. In year two of this study, apple trees were in full bloom on May 10, 2018. All blossoms were counted on the middle two experimental trees of each root box, and blossoms were removed from all experimental trees. On May 5, 2018, fully expanded green leaves were collected from mature 'Golden Delicious' apple trees, and leaves were dried and prepared for the organic-N treatment as described above. Fine roots appeared in the root box windows on June 13, 2018 and on June 14, 2018, unfertilized control (water), inorganic-N and organic-N treatments were applied to root box windows as described previously.
Percent soil moisture and soil temperature were measured bi-weekly at depths of 15 and 30 cm in each root box as described previously. Microlysimeters were installed on June 15, and soil solution samples were collected bi-weekly as described above. Samples were analyzed for total N (Brookside Laboratories, Inc., New Bremen, OH).
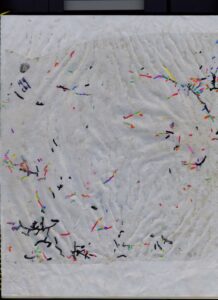
Root observation and harvest. In year one of this study, new root growth was traced every two to three days on the acetate film of the root box windows using a different colored marker (Uchida of America, Corp.; Torrance, California) for each measurement date (Fig 2b). Roots were traced from September 13 to October 24, 2017. New roots were also traced on the day of root collection in addition to 1, 2, 4, and 6 days prior to collection from the windows. On October 25, fine roots were harvested and stored in 1.5-ml Eppendorf tubes at 4˚C until further analysis. The acetate film used for the treatment windows was collected from the root boxes.
In year two, new root growth was traced every two to three days as described previously. Roots were traced over two measurement periods from June 14 to July 19, 2018 and August 10 to September 5, 2018. On July 19 and September 5, 2018, fine roots were harvested and stored in 2 ml Eppendorf tubes at 4˚C until further analysis. The acetate film used for the treatment windows was collected from the root boxes, cleaned, and scanned at 400 dots per inch (DPI) using an Epson Perfection 4490 Photo Scanner (Epson America, Inc.; Long Beach, CA). Root tracings on the scanned images were analyzed for root length, root number, and root age based on tracing color with ImageJ 1.46r (Schneider et al., 2012).
Subsamples of roots from each measurement period in 2017 and 2018 were selected for microscopic observations and quantification of fungal colonization. Roots selected for microscopy were cleared in 10% KOH at 75˚C and stained with 0.05% trypan blue in a lactic acid:glycerol:water (1:1:1) solution for 30 minutes. Roots were destained in a lactic acid:glycerol:water (1:1:1) solution overnight and stored in destain solution until observation (Brundrett et al., 1996). Samples were viewed with a compound light microscope. Individual roots were mounted parallel to the long axis of a microscope slide with grid lines and covered with a 40x22 mm cover slip. At each grid intersection, fungal colonization of arbuscular mycorrhizal (AM) and non-mycorrhizal (NM) fungi was recorded to determine the percentage of root length colonized.
Extramatrical and internal fungal development. Soil samples were collected from behind each treated window section in 20-ml scintillation vials at each root harvest in 2017 and 2018. Soil samples were stored at -80˚C until further analysis. Soil samples were freeze dried at -5˚C for 4 days. Phospholipid and fatty acid (PLFA) extractions were used to estimate extramatrical hyphae biomass which could indicate changes in hyphal foraging of arbuscular mycorrhizal (AM) fungi in response to nutrient additions. Phospholipid lipid extraction and analysis followed the method described by Bossio et al. (1998). Briefly, polar lipids, containing phospholipids, were extracted from the soil using organic solvents. The internal standards for PLFA were the fatty acid methyl esters 21:0 (Avanti Polar Lipids; Alabaster, AL). Lipids were separated into PLFA fractions using silicic acid solid-phase chromatography columns. Lastly PLFA wias converted into fatty acid methyl esters (FAME) through methanolysis. Extracted FAMEs were analyzed using a HP GC-FID (HP6890 series, Agilent Technologies, Inc.; Clara, CA) gas chromatograph. External FAME standards (K101 FAME mix, Grace; Columbia, MD) were used to determine biomass. Biomarkers were identified using the Sherlock System (v. 6.1, MIDI, Inc.; Newark, DE).
Microbial diversity in soil is generally very high, but the fungi of interest will only include the beneficial AM fungi and the small percentage of soil-borne fungi implicated in apple replant disease which are Phytophthora spp., Pythium spp., Cylindrocarpon spp., and Rhizoctonia spp. To investigate which fungal groups colonized the root, 120 individual roots with known growth rates from 2017 and 2018 root samplings were selected for next-generation DNA sequencing. For illumina sequencing, the primer ITS2, was selected to target AMF fungi. For NM fungi, ITS1 and ITS4 primers were selected for sequencing. DNA sequencing of individual roots was proposed as an approach to improve our understanding of the specificity of root-fungal interactions; however, isolating high-quality fungal DNA from a single fine root was challenging. After consulting with a private company (Bejing Genomics International; Cambridge, MA) to troubleshoot and improve DNA extraction methods, samples were eventually sent directly to BGI for DNA extraction and sequencing. Roots of similar growth rates within each treatment combination were combined to obtain 100 mg fresh weight of root tissue per sample. However, DNA concentrations were only sufficient for sequencing in 25% of the samples. Therefore, 75% of the samples failed library preparation and could not be sequenced. There is additional difficulty sequencing AM fungi because they are not detected by standard PCR primers commonly used for other types of fungi.
This was a setback to the project and has caused further delays; however, after consulting with other Penn State faculty members and the director of the Huck Institute Genomics Core Facility, I am pursuing a new approach to sequencing arbuscular mycorrhizal fungi using PacBio. PacBio has a much higher chance of successfully sequencing DNA for arbuscular mycorrhizal fungi due to the technology used. I am also using a different primer set that has a higher likelihood of amplifying AM fungal DNA regions of the ribosomal small subunit (SSU). Under the direction of Dr. Terry Bell at Penn State University, I have learned the procedure for high-throughput DNA extractions and am currently testing DNA quality to provide sufficient DNA concentrations for sequencing.
Additional considerations: Trees were managed with standard agricultural practices for the eastern United States. Shoot extension and trunk circumference were measured to quantify above-ground tree growth in response to replant site location. Total blossom clusters was also counted for each treatment tree.
Statistical analysis: Total nitrogen content in soil solution, soil temperature, percent soil moisture, root length, and root number data were analyzed using analysis of variance (ANOVA) or analysis of covariance (ANCOVA) to test for statistical differences between replant location and N source using SAS’s Glimmix Procedure (Cary, NC). If interactions were significant, multiple comparisons were conducted using the slicediff option in SAS Glimmix. Interactions between root growth rate, AM fungi and non-mycorrhizal fungi were investigated using mixed models in Proc Mixed. Due to the unpredictability of root production both spatially and temporally, techniques to analyze root and colonization data and account for natural variation are still being developed.
Literature Cited
Bossio DA, Scow KM, Gunapala N, Graham KJ (1998) Determinants of soil microbial communities: Effects of agricultural management, season, and soil type on phospholipid fatty acid profiles. Microb Ecol 36:1-12
Brundrett M, Bougher N, Dell B, Grove T, Malajczuk N (1996) Working with mycorrhizas in forestry and agriculture. Australian Centre for Int Agric Res Monogr Ser Number 32. Pirie Printers, Canberra, Australia
Moore TR, Trofymow JA, Prescott CE, Fyles J, Titus BD, CIDET Working Group (2006) Patterns of carbon, nitrogen and phosphorus dynamics in decomposing foliar litter in Canadian forests. Ecosyst 9:46-62
Schneider CA, Rasband WS, Eliceiri KW (2012) NIH Image to ImageJ: 25 years of image analysis. Nat Methods 9:671-675
The results of the comprehensive assessment of soil health rated the herbicide strip as a medium soil (quality score of 54) with lower biological ratings than the grass row soil which was rated as excellent (quality score of 60). Notably, the biological indicator, active carbon, was identified in the assessment as low (value of 337) in the tree row compared to the grass row (value of 401). This may have be important for tree establishment and long term tree health as active carbon provides energy to support soil microbes.
Nitrogen content in soil solution collected with microlysimeters was higher in the inorganic (urea) and organic (ground apple leaves) than the control (water) regardless of sampling date which confirmed that the nutrient treatments provided a nitrogen (N)-rich patch (P=0.05; data not shown). Nitrogen content of soil solution was not different between inorganic and organic treatments which supported our efforts to provide a similar amount of N in the nutrient-rich patches regardless of N source.
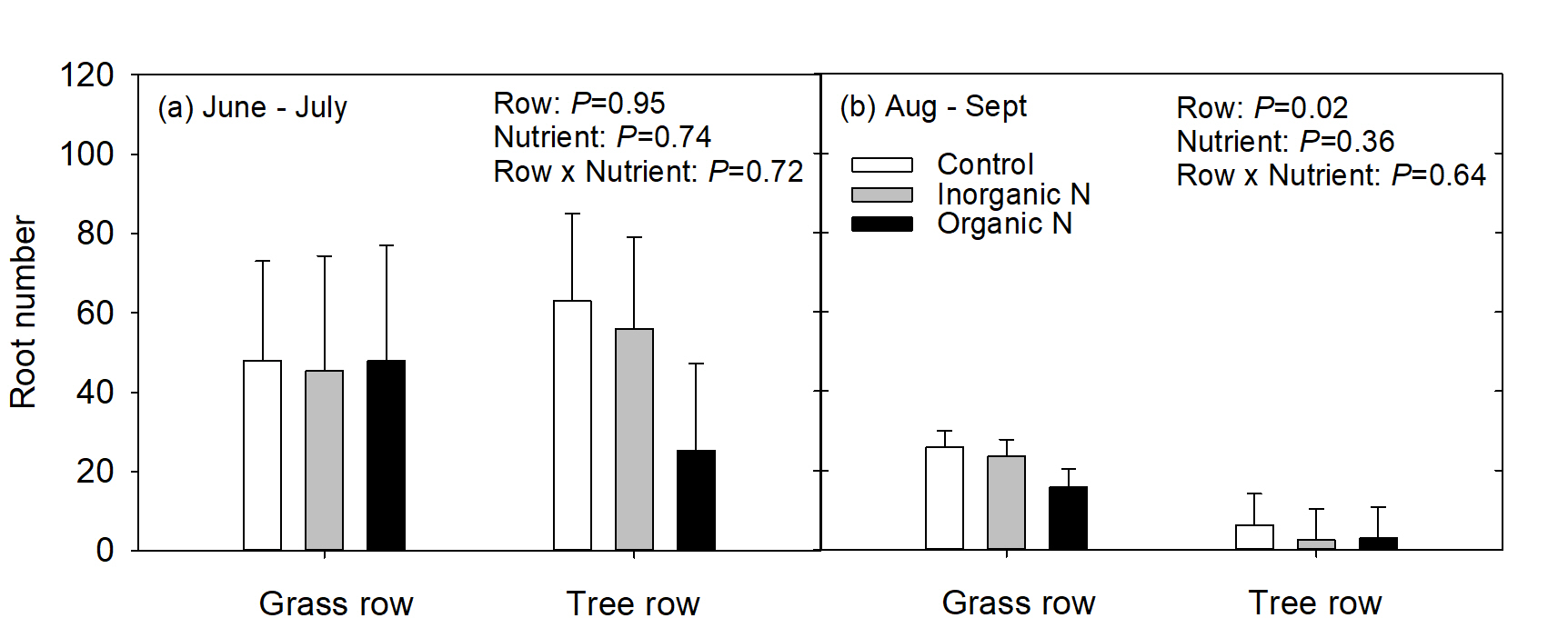
Due to the low numbers of roots and the variable root development between root boxes in 2017, data analysis for 2017 was challenging. Therefore, only data for 2018 will be presented and discussed here. More roots grew in the 1st measurement period from June to July than the 2nd measurement period from August to September (P<0.01). This may be simply due to the extended time that roots were observed in the 1st measurement period; however, a root study of mature apple trees that she conducted in conjunction with this experiment also showed that peak fine root production occurred in July. In June and July, root production (mean root number) was similar in both the grass row and tree row, and nutrient addition had no affect on root number (Fig 3a). In the 2nd measurement period, trees growing in the grass row produced more roots than trees growing in the tree row, but nutrient addition had no effect on root number (Fig. 3b). Production of fine roots is important for nutrient foraging and uptake. Higher root production enhances soil exploration and increases the amount of absorptive root surface area contact with the soil to enhance nutrient uptake.
In the 1st measurement period, mean root length (mm) was similar in trees growing in the grass row regardless of nutrient addition; however, in the tree row, roots growing in the inorganic-N patch were shorter than roots growing in the control and organic-N patches (Fig. 4a). In the 2nd measurement period, mean root length was similar regardless of row or nutrient addition (Fig. 4b).
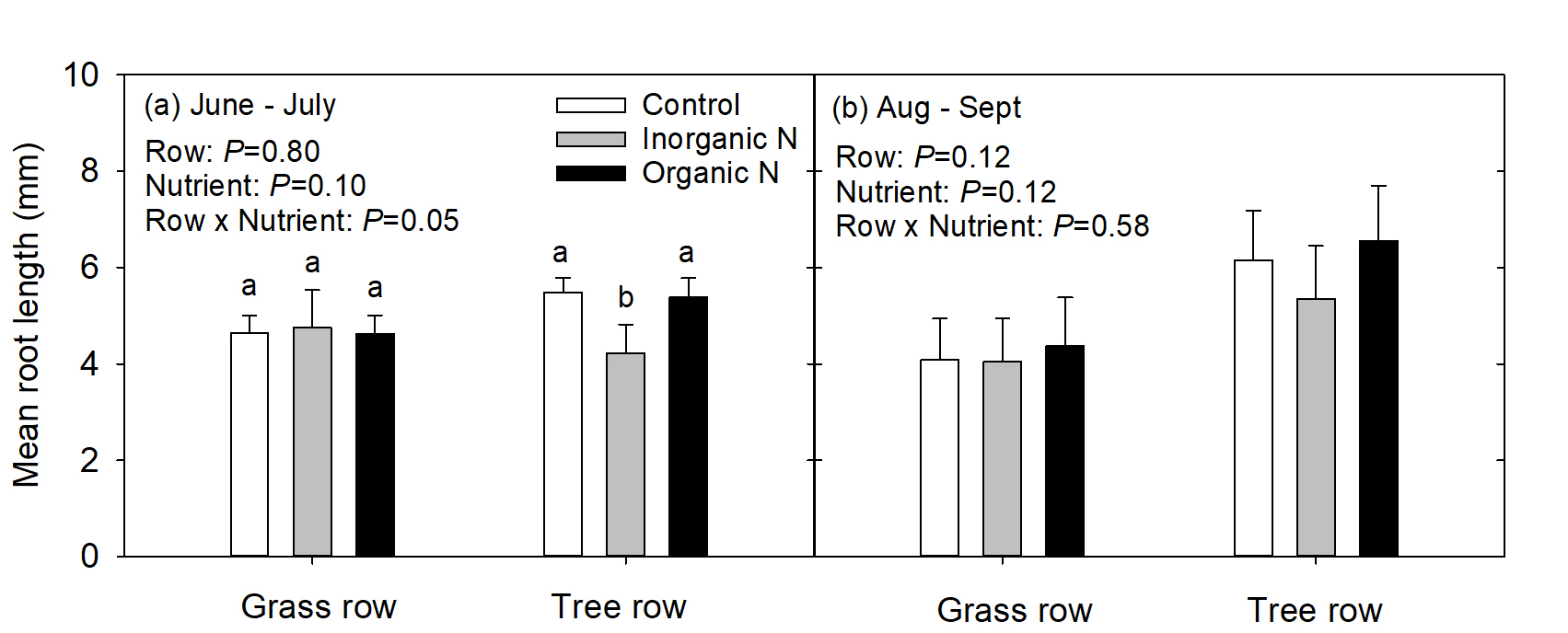
Roots were also categorized into fast- and slow-growing roots based on root tracings. If roots grew more than 3.5 mm per day, the were considered fast growing. If roots grew less than 3.5 mm per day, they were considered slow growing. This growth rate characterization was also supported by previous work conducted on roots of mature apple trees at the Penn State Russell E. Larson research farm (Resendes et al., 2008). During the 1st measurement period for trees with grown in the grass row, slow-growing roots made up 80% of the root system while fast-growing roots only made up 20% of the root system. Similarly, for trees grown in the tree row, 74% of the root system was made up of slow-growing roots while the remaining 26% of roots were fast-growing. These proportions of fast- and slow-growing roots were similar in the 2nd measurement period as well. In June to July, row did not affect the number of fast or slow growing roots (P>0.05, Fig. 5a), but in August to September, there were more slow growing roots in the grass row than the tree row (P<0.01) Fig. 5b). Nutrient addition had no affect on the number of fast- or slow-growing roots (P>0.05) in either the 1st (Fig. 5c) or the 2nd (Fig. 5d) measurement period. This was surprising because in a study in mature apple trees conducted prior to this experiment , addition of mineral N enhanced root production, and total root length (Lavely et al., 2018). Perhaps young trees respond differently to nutrient-rich patches than established trees. This may be a function of prioritizing carbon cost for root system construction for establishment over rapid N uptake.
Figure 5. LS means for the number of fast- or slow-growing roots produced by trees in response to the grass or tree row in June to July (a) and August to September (b) 20189- and in response to addition of control (water), inorganic N (urea), or organic N (ground apple leaves) in a nutrient-rich patch.
PLFA analysis did not detect differences in extramatrical hyphal biomass of AM fungi regardless of row or nutrient addition in either measurement period (P>0.05, data not shown). Similarly, root length colonization was not affected by row or nutrient addition (P>0.05, data not shown). Although enhanced AM fungal biomass and root length colonization were expected with the addition of N in young apple trees, particularly with the organic-N addition, marginal fungal responses have been previously observed in mature apple trees (Lavely et al., 2018). It may be that apple trees do not rely heavily on mycorrhizal fungi for nutrient acquisition but rather allocate energy for root construction over mycorrhizal partners. It may also be that beneficial bacteria play an important role in nutrient cycling and availability for roots in this system which went undetected in this experiment.
Soil temperature and soil moisture were similar regardless of row at both 15 and 30 cm depths (P>0.05; Fig. 6a and b). Soil temperature was higher at 15 cm than 30 centimeters during the root measurement period. Soil moisture was similar at both measurement depths.
Figure 6. Soil temperature (a) and percent soil moisture (b) at 15 and 30 cm depths.
Tree circumference increased over time as expected (P<0.01). Trunk circumference of trees in the tree row or grass row did not differ at planting (TR=1.55 ± 0.03 cm, GR=1.59 ± 0.04 cm; P=0.51 ) or at the end of year one when trees were dormant (TR=7.12 ± 0.14 cm; GR=7.22 ± 0.14 cm=; P=0.62). At the end of year two, tree circumference was higher (P=0.02) in the grass row (10.68 ± 0.20 cm) than in the tree row (10.04 ± 0.20 cm).
Trees had fewer blossom clusters per tree in year one (Total blossom clusters=15.0) than year two (Total blossom clusters=158.0) of this study (P<0.01). The effect of planting location (TR or GR) had no effect on blossom clusters (P =0.66). In year one of the study, average shoot length per tree did not differ in response to planting location (TR=14.66 ± 0.61 cm, GR=14.66 ± 0.41 cm; P=0.77). Average shoot length was greater in year two than in year one (P<0.01), and trees in the grass row had higher average shoot length (GR=36.36 ± 0.79 cm) than trees in the tree row (TR=31.43 ± 1.03 cm; (P<0.01).
Literature cited:
Lavely EK, Zhang J, Adams TS, Bryla DR, Deforest JL, Marini RP, Crassweller R, Eissenstat DM (2018) Root and mycorrhizal fungal foraging responses to increased fruit removal in apple trees
Resendes ML, Bryla DR, Eissenstat DM (2008) Early events in the life of apple roots: variation in root growth rate is linked to mycorrhizal and nonmycorrhizal fungal colonization. Plant Soil 313:175-186
This research suggests that young apple roots may grow more when planted in an old grass row middle in August to September than when planted in an herbicide strip where apple trees were planted previously. Trees grown in the grass row not only produced more roots during that time period, but they also produced more slow-growing roots. Enhanced production of slow-growing roots may allow these small root systems to explore more soil with greater soil surface contact compared to a root system that has fewer, faster-growing roots. However, manipulating root system growth through localized fertilization may have little affect on root system structure as was shown in this study. This research also suggests that roots of young apple trees may depend more on their roots for nutrient foraging than their AM fungal partners.
These results highlight that there is still a need to learn about basic root physiology, particularly of young trees as they seem to differ from previous studies on mature apple trees. This research suggests that there are more opportunities tailor nutrient management strategies to roots in various soil health conditions. Interestingly, there is not strong evidence to support the influence of AM fungi on root production or nutrient foraging of young apple trees. While maintaining healthy soils is important for ecosystem health, there may not be a strong benefit to using pre- or post-plant mycorrhizal inoculation products as has been suggested. However, this should be further evaluated.
Education & Outreach Activities and Participation Summary
Participation Summary:
A tour of the Penn State research farm was given to the Young Grower Alliance, and 2 tree fruit researchers and educators were present. I presented information about my research experiment to about 8 tree fruit growers and answered questions about soil health. I also presented my research to a group of about 10 undergraduate and graduate students enrolled in a tree fruit production class. We discussed apple replant disease, soil health, and mycorrhizal colonization in newly planted apple trees.
Project Outcomes
This project illustrates an important approach to investigating root growth in individual roots of young trees and the challenges researchers and growers face to enhance the sustainability of perennial agricultural systems. There are logistical and biological challenges to investigating part of the plant that is underground, and roots are difficult to manipulate. There is a lot of fruit production knowledge. Growers understand how to produce high quality fruit by manipulating the aboveground part of the trees; however, the belowground contribution is often unpredictable and may not be considered from an economic perspective. Therefore, basic knowledge on how to manipulate or enhance efficacy of individual roots and whole root system responses is still needed
Future opportunities that could contribute to sustainability may include tailoring management strategies to target roots at a particular life stage and root developmental stage. For example, if a tree is producing more structural roots, such as pioneer roots, than fine absorptive roots, delaying fertilizer application until more fine roots have been produced would enhance nutrient uptake efficiency. This could minimize monetary waste for a grower and help minimize nutrient pollution through runoff as plants have a higher likelihood of capturing nutrients when fine root are present. This change in the proportion of structural to absorptive roots also has ecological impacts on soil microbes. For example, pioneer roots often do not support mycorrhizal fungi or may only do so for approximately 2 weeks until secondary development occurs in woody roots which inhibits colonization by arbuscular mycorrhizal fungi. This may affect young trees more than mature trees since the proportion of structural pioneer roots to absorptive roots in young trees than established apple trees.
This project has provided invaluable experience both scientifically and professionally. To our knowledge, observing individual roots of newly planted apple trees with root boxes had not occurred in a field setting. My awareness of the effects of agricultural practices on soil health was heightened. The observation of different soil characteristics as a result of grass or a relatively small herbicide strip reflects how some agricultural practices have a large and lasting affect at different landscape scales.
My future research interests include investigating root system physiology and management of young trees. I am also very interested in the application of pre- or post-plant inoculum on health of individual roots, microbial communities, and the host plant. Although it has been a challenging part of this project, it is important to understand root-microbe interactions across molecular and physiological techniques. These interactions are an important part of a healthy ecosystem. I am interested in exploring key players in the bacterial community as well that may affect root health, nutrient availability in agricultural systems, and management of apple replant disease or other soil-borne diseases.
I am currently collaborating with Dr. Rob Crassweller to evaluate the effects of a mycorrhizal inoculum on fine root development in apple trees. I am also interesting in investigating the efficacy of root colonization of non-native AM fungal species compared to native AM fungi.