Final report for GNE17-158
Project Information
The purpose of this project: was to evaluate the use of an Aerated Fluidized Bed Reactor (aerated-FBR) combined with Constructed Wetland Treatment (CWT), for reclaiming dairy-lagoon wastewater effluent (DW).
A two-step approach was proposed whereby
(a.) ammonium-nitrogen (NH4-N), orthophosphate (PO4-P) and potassium (K) are extracted by precipitation of struvite (MgNH4PO4·6H2O) and K-struvite (MgKPO4·6H2O), using an aerated-FBR
(b.) total suspended solids (TSS), dissolved organic carbon (DOC) and nutrients are removed by CWT to produce irrigation waters.
Research approach The aerated-FBR-CWT method was used to reduce livestock farm operation costs by recycling nutrients as struvite fertilizer and reclaiming DW as irrigation water. In order to evaluate this method, a pilot scale site was built with a 40-L aerated-FBR reactor and three CWT pools, 3000-L each. The pilot site aerated-FBR evaluated: the use of aerated-FBR in continuous mode with a chemical resistant auger, MyCodo, an open source farm management software for optimizing treatment outcome, and the effect of Mg addition to DW on P recovery. Two CWT pools types were tested: submerged vegetated bed (SVB) and free water surface (FWS), were used in the pilot site. The CWT pools used local plants for model wetland and were tested for treating DW with / without aerated-FBR treatment.
Key finding The aerated-FBR treatment method, scaled-up from lab to continuous flow pilot-scale, met the expected recovery rate ~60% dissolved phosphate (P) harvested as struvite fertilizer, with minor apparatus modification. The difference in the scale-up version was the addition of a robust vent for excess foam removal and extra consideration to foam containment (sealed mixer bearing, gas tight reactor lid). For the continuous DW flow, an auger feed was found to perform better than conventional peristaltic pumps as it is less prone for clogging and require minimal maintenance. The micro-controller (Raspberry- Pi) and farm automation open source software (MyCodo) met all the pilot operation needs with durability, precision of treatment application and quality of data logging output.
Finding from CWT evaluation showed that the local plants of Wide/Narrow leaf Cattail, Smooth Cord-grass, Common Reed and Sweet-Flag were able to acclimate and grow in DW, and remained viable with effluent pH 9. The contaminant removal of TSS/COD resulted with a residual 1000 mg/L TSS (62% removal) and 3850 mg/L (30% removal) COD, these were still far from the desired treatment goals of 30/60 mg/L. More work is under consideration to improve CWT outcome by using biochemical applications to lower the pH of DW and reduce pH stress on the CWT pools. Additional factors that hinder CWT performance were high water level (20-inch-deep) and excess heat stress for plants inside the protective rain cover tunnel.
Potential impact Our group is interested in future study opportunities to optimize this approach to the treatments needs of swine manure and other livestock wastewater types.
The aerated-FBR+CWT method scale-up to pilot-site size has kept the expected nutrient recovery and removal rates, with a potential for providing a significant reduction in nutrient deposition from DW. The use of nutrients removal methods for DW is expected to be a viable approach to fight harmful algae bloom in many watersheds in the region.
The viability of the open source farm automation software MyCodo, used to control the aerated-FBR make it a suitable solution for many farm applications such as feed management, irrigation, greenhouse management and more. A farmer training program is being developed in collaboration with local farmers organisations on how to use open source farm automation, using the finding from this pilot scale test.
Note of changing of experiment set-up from swine and dairy farm to dairy only, and expanding work over two growing seasons:
The original proposal for GNE-158 aimed to evaluated the aerated-FBR + CWT set-up both in a dairy and swine farm. During the process of placing the CWT pools at site one, Fulper dairy farm it became apparent that relocating the experiment is not feasible. For this reason, a change of experiment outline was made for conducting the experiment only at the dairy farm site. The rationale behind this approach is that since the overall nutrient content, BOD and TSS were significantly higher for dairy site, conclusions from this work will include the treatment needs required for swine wastewater treatment.
- Assessment of DW properties to determine aerated-FBR-CWT requirements:1. Assessment of DW properties to determine aerated-FBR + CWT requirements. The study location: Fulper farm, Hunterdon County. An on-site study will characterize the DW, including TSS, BOD and nutrient content, in order to design the most suitable CWT
- Produce struvite fertilizer for crop production by recycling nutrients from DW: The aerated-FBR will be used to recover OP and NH4-N from DW by induced precipitation of struvite, to be recycled on-site as fertilizer. The measurement of success for aerated-FBR treatment is >70% PO4-P removed with >60% struvite content in the precipitated salt for DW.
- Implement CWT to meet primary treatment water discharge requirements: While traditional DW treatment does not contribute to farm water balance, CWT can reclaim the bulk of effluent water for irrigation by removing remaining nutrients and contaminants. The current standard for primary effluent treatment is 30/30 mg/L BOD/TSS. CWT will need to reduce the BOD/TSS concentrations accordingly if the water is to be reused. The assumption is that if a consistent and stable CWT system can be constructed to remove nutrients, BOD/TSS requirements can be attained by expanding the system in a series of CWT structures. The measurement of success for CWT is therefore meeting the effluent discharge requirements, making treated DW usable for crop
- Industry outreach: Dissemination: The aerated-FBR-CWT method will be promoted by hosting on-site tours of the treatment facility for local producers, high school students and researchers at the RU-NB farm site, and by attending agronomy expo
- STEM outreach: Undergraduate student research: To encourage more students to engage in STEM-related sustainability research, undergraduate students will be directly involved in the project. These students will be supported by National Science Foundation (NSF) Research Experiences for Undergraduates (REU), and Garden State-Louis Stokes Alliances for Minority Participation (GS-LSAMP) programs at Rutgers University-Newark (RU-N). The students will conduct on-site research at the RU-NB farm, compile reports, and present results at local
- Scientific and public outreach: Findings will be published in scientific peer-reviewed journals as part of Mr. Rabinovich’s doctoral dissertation. Results will be presented at national meetings, such as the American Chemical Society (ACS) meetings. Presentations at local events will inform the general public.
Environmental challenges of DW:
Dairy cows generate ~20 gallon/day-animal (gal/d-a; estimated for Holstein cow) of DW(1), with high concentrations of dissolved nutrients and other components. DW is the sum of flush effluents from milking parlors, yards and stalls which is stored in wastewater lagoons. High levels of PO4-P, NH4-N and TSS generate harmful BOD(2), and decompose into contaminants such as NO3-N(3). The common treatment practice is applying DW onto fields as fertilizer using cannon sprinklers or spraying vehicles(4). DW can impact agricultural soil, groundwater and surface water, and its odor can be a nuisance. The high NH4-N content in DW is converted into NO3-N by nitrification, resulting in nitrate contamination of groundwater through leaching. Particulate PO4-P is susceptible to aeolian transport which introduces it to open water sources and contributes to eutrophication.
Sustainability issues being addressed:
Many livestock farms also grow a portion of their livestock feeding crops on site, and maintaining soil fertility is a significant component of operation costs. Spraying DW over crop fields is an acceptable, but less sustainable approach to waste disposal. Moreover, in events of high rainfall and rising groundwater table, the constant need to dispose the lagoon effluent can be costly in and labor-intensive.
In addition to environmental problems, the spraying method for disposing DW also presents agronomic disadvantages. Non-composted DW is a lower-grade fertilizer compared to compost and mineral fertilizers with regards to nutrients chemical stability and provides an imprecise nutrient application(5). The higher volatility and bio-availability of NH4-N, compared to PO4-P in soils often results in excess P application to meet the crop's N demand, or the need for additional fertilizer. In addition, excess water is applied via spraying. Therefore, DW spraying has low water preservation and fertilizer application efficiencies.
Aerated-FBR-CWT reclaims key nutrients, PO4-P and NH4-N, as struvite fertilizer and produces treated water for targeted irrigation. The 1:1:1 ratio of N:P:Mg distinguishes struvite as a PO4-P, NH4-N and magnesium (Mg) a macronutrient(6). Application of Mg benefits the soil structure by reducing swelling and the exchangeable sodium ratio in clay soils(7). Production of struvite combined with irrigation water maintains the regional mass-balance of these resources as less fertilizer and water are required from outside the farm resource-cycle.
Reclamation of DW:
Preliminary work used a bench-scale aerated-FBR to reclaim struvite from swine and dairy lagoon wastewater. Results showed 64% PO4-P removal for dairy. P removal was achieved through Mg addition and increasing the pH to 9. Solids collected from aerated- FBR has high struvite/K-struvite content (20-60%), mixed with calcite (CaCO3). Use of CWT to treat wastewater is well established with two major treatment concepts of submerged and open water flow, with variations depending on local climate, fauna and biota. The research group is currently working on testing an adaptation to dairy CWT treatment that aims to reduce both TSS and BOD levels in DW to meet 30 mg/L discharge standards.
Through the first work season of the current pilot project through 2018, A 40-Liter pilot scale aerated-FBR and three CWT pools, ~3000-Liter each, were placed at the Fulper farm site. The aerated-FBR was tested and modified to meet the needs of continuous operation. An open code farm management software was implemented into the reactor microcontroller and evaluated. The CWT were populated with native wetland plants and their viability was tested in the present of effluent from the aerated-FBR outflow.
Cooperators
- (Researcher)
- (Researcher)
- (Researcher)
Research
In order to meet the project objectives, the performance of the two-step aerated-FBR + CWT approach was evaluated in pilot-scale at a dairy farm site. This treatment included applying struvite recovery, followed by contaminant removal for wastewater. Overall, DW includes all the liquids generated in the farm yard, stalls and milking parlor by livestock and maintenance procedures. These are drained to a separation pool with a two- chamber cell connected by an overflow canal. The bulk of the solids sink to the bottom of the first cell, while effluent overflows to the secondary cell. and from there the effluent is drained into the storage lagoon.
Figure 1: Study site, dairy lagoon
The two-step treatment method used aerated-FBR + CWT applied in a continuous flow canal system. DW was pumped into the aerated-FBR and from there, the outflow was directed by gravity into the CWT canal for primary wastewater treatment. A clear tunnel cover was used to cover the CWT and prevented rain addition from diluting the measurement of DW treatment.
DW nutrient removal using aerated-FBR:
Effluent from the lagoon was pre-filtered using a crushed rocks matrix filter (55-gallons barrel filled ~1/2 way with a ¾” crushed rocks), using submerged seepage pump. From there, DW was pumped into the aerated-FBR reactor with an auger pump (Figure 2). The DW level in the rock matrix filter was kept constant with a vertical floating switch (LVN-60, Omega) that control the submerged seepage pump (the seepage pumps used another float switch to protect from low DW level in the lagoon). A drain valve was used to empty the filter for maintenance and removing bio-films periodically by applying one gallon of household bleach and flushing the reactor/ filter system several times to remove bleach traces.
For the auger pump, a 3-inch diameter chemical resistant PVC auger fed the DW into the FBR (Custom built), using 24 VDC;54-rpm gear motor (5LAG3, Dyton) with a pulse width modification (PWM) control board (Cytron, 30A 5-30V). The auger was designed with inner radius of 1.25-inch and 1.5-inch helix opening for proper DW uptake at low rpm. A sprocket ratio of 4:1 between gear motor (60T) and auger (15T) gave better sensitivity with DW uptake and prevented engine wear. When set to 36% power output, the auger pumped DW at 0.6 L/minute.
The reactor pH was logged using a raspberry Pi micro-controller. The Raspberry pi system used MyCodo software(8), an open code platform that allowed to collect data and apply agricultural environment control. The Mycodo feedback setting collected analogical sensors inputs such as pH, oxidation reduction potential (ORP), temp (T), etc., using a data isolation mount (Tentacle T3, WhiteBox) with appropriate digital signal convectors (Atlas scientific). The Mycodo program then governed power relay system (8X5vdc-relay, Sainsmart) to adjust pH in the aerated-FBR to meet nutrient recovery needs using peristaltic pumps (Fisher Scientific FH100).
Figure 2: FBR Setup
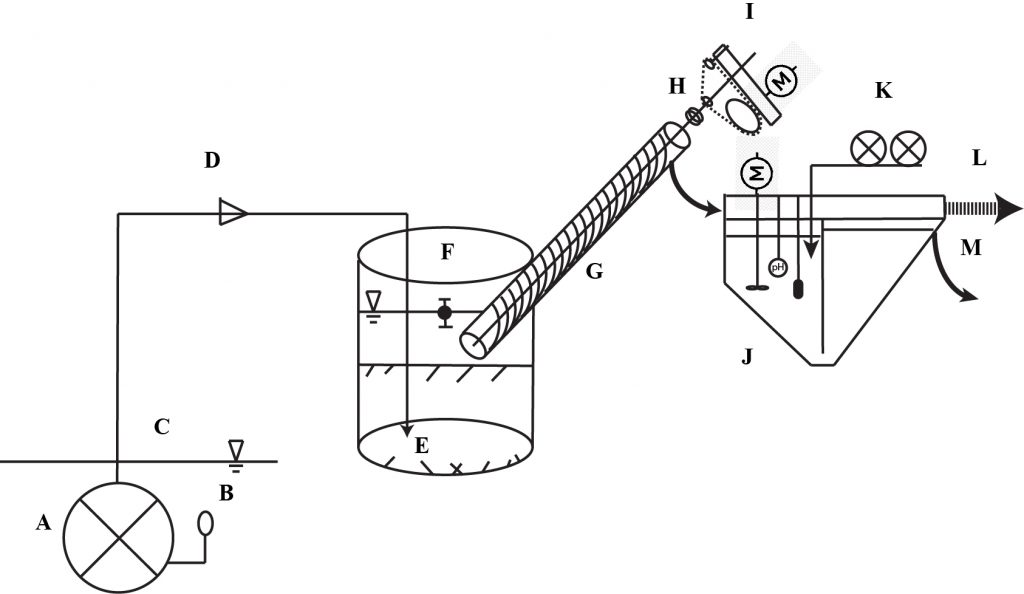
Auger design criteria:
For compact placement, the maximum recommended helix angle was 450 for an auger pump, and for a single helix auger, a pitch (span of one helix circumvent) of 1*R-auger was needed. PVC material was chosen for durability, low cost and chemical resistance to acids and bases. The table below shows the recommended sizing for auger building based on auger modeling recommendations. For centering of the auger a PE bearing with stainless steel balls was used, although its more costly, it does not require any maintenance and is chemical resistant. To fabricate the auger helix, PVC discs were cut to the desired in/out diameter and glued together with high density/ chemical resistant PVC cement (e.g. Weld-On 724), and the helix was stretched over the inner PVC tube. The helix+axis was then cemented together with the same PVC cement. Test on site auger lasted +6-month and its structure flexibility prevented excess wear of auger.
Sizing table for one helix auger at 45 degrees (units in inch) | |||||||||
Inner PVC pipe size (in) | 1/2 | 3/4 | 1 | 1-1/4 | 1-1/2 | 2 | 2-1/2 | 3 | 4 |
Actual inner diameter | 0.84 | 1.05 | 1.315 | 1.66 | 1.9 | 2.375 | 2.875 | 3.5 | 4.5 |
Optimal outer diameter | 1.53 | 1.91 | 2.39 | 3.02 | 3.45 | 4.32 | 5.23 | 6.36 | 8.18 |
Approximated available diameter | 1.5 | 2 | 2.5 | 3 | 4 | 4 | 5 | 6 | 8 |
Helix pitch | 0.76 | 0.95 | 1.20 | 1.51 | 1.73 | 2.16 | 2.61 | 3.18 | 4.09 |
Target hole saw in | 0.87 | 1.09 | 1.37 | 1.70 | 1.98 | 2.47 | 2.99 | 3.64 | 4.68 |
Target hole saw out | 1.52 | 2.02 | 2.53 | 3.02 | 4.04 | 4.06 | 5.07 | 6.08 | 8.11 |
For hole saw add ~1/8 inch to hole-saw size | |||||||||
Recommended PVC sheet | |||||||||
0.063 | 0.063 | 0.063 | 0.063 | 0.063 | 0.063 | 0.125 | 0.125 | 0.125 |
Struvite fertilizer production
Nutrients recovery was facilitated by the precipitation of struvite from DW to remove PO4-P. The mineral struvite is made up of Mg2+, PO43- and NH4+ ions in a 1:1:1 ratio. Struvite precipitation can be described by the following reaction (For K-struvite, substitute NH4 with K):
Mg2+ + NH4+ + PO43- + 6H2O = MgNH4PO4·6H2O
Struvite has a low solubility product with Ksp=10-13.26, indicating that mineral dissolution is low, contributing to its properties as a slow-release fertilizer. For DW both the Mg (~0.01M) and PO4-P (~0.0015 M) concentrations tend to be consistently lower than NH4- N (~0.033M), making them the limiting factor for struvite formation. Another factor affecting struvite formation is the high calcium concentration of DW that can promote the formation of other PO4-P minerals such as hydroxylapatite (Ca5(PO4)3OH). This may result in an inferior fertilizer with regard to P availability in soil solution, due to the extremely low solubility of hydroxylapatite (log Ksp= -44.33) compared to struvite.
Adding supplementary Mg to the effluent as magnesium chloride hexahydrate (MgCl2·6H2O) can promote the formation of mostly struvite salts and reduce the apatite content. The rate of precipitation is also driven by pH, where the reaction time, represented as hydraulic retention time (HRT), was the time required for nutrient removal to reach treatment goals at pH 9. The pH of DW ranges from 7.5-8, depending on the depth of the lagoon, DW type (open lagoon, closed tank) and the time of year average temp. To meet the pH 9 requirements in the aerated-FBR, DW pH was raised by carbon dioxide stripping with aeration to pH 8.2-8.5 and supplemented with sodium hydroxide (NaOH) titration to pH 9.
At an inflow rate of 10 gallons/hour the aerated-FBR had ~60 minutes HRT allowing enough time for precipitation, and the ability to treat up to 240 gallons of DW per day. The main chamber generated the bulk of struvite crystallization as incoming DW became saturated and interacted with struvite colloids. For initial struvite nucleation, 0.1g/L (4 g/reactor-run) of fine-grained struvite powder was used as seeding for struvite crystal nucleation, acting as a template for crystal growth and precipitation. Aeration at a 45 cu.ft. air per cu.ft. DW-hour raised pH up to ~pH 8.5 by CO2(g) stripping, using a linear diaphragm air compressor (Thomas, 2-cu.ft/min). To further induce precipitation, 2.5N NaOH, 0.2 M MgCl2·6H2O solutions were added using two peristaltic pumps based on the experimental treatment. The pH of DW was measured in the reaction chamber using an inflow pH electrode (self-cleaning, Cole-Parmer). The main chamber used an overhead mixer (Arrow 1200) with a PTFE coated mixer shaft and a 4-inch blade, generating a circular flow promoted crystallization and generated suspended particles, preventing struvite sedimentation on the reactor walls. A narrow-slit passage connected the continuous flow stirred-tank reactor chamber (CSTR) and fluidized bed (FB) chambers in the aerated-FBR. The FB conical prism reduced the DW flux from an average of 20 cm/min at the entry slit, to an average 2 cm/min at the point of exit. As struvite particles velocity slowed, they cease to be suspended and vertical settling transported the crystals downward. This circular motion of FB separated the suspended salt solids from the DW effluent leaving the reactor, and enabled the crystals to undergo an extended growth period and to be harvested. The FB formation was set by adjusting the mixing speed of the aerated-FBR overhead stirrer to ~400 rpm.
Below, a sample database output for the aerated-FBR system:
Data from the aerated-FBR MyCodo data-logger for a one month run, showing the rate of OH application, ambient air temperature and average reactor pH over time. The Raspberry pi micro controller reports CPU temp to determine potential damage to the instrument.
DW primary treatment with CWT:
CWT allowed microbial, flora, chemical and mechanical processes to remove contaminants from DW. Our CWT system used a three-cell in an open canal setting. Cells were: 1. Free Water Surface (FWS) 2. Vegetated Submerged Bed (VSB) cells settings. The HRT requirement for each cell was two days, for a total HRT of six days. Each CWT cell consisted of a wood beam frame with plywood support and 45-micron synthetic rubber (EPDM) liner for effluent containment. The total DW mass in the CWT is the total volume times the cell porosity. For our HRT assessment, we used estimated porosity values of 0.7, 0.3 and 1 for the FWS (emerged plants), VSB (submerged flow with plant cover) and open FWS (submerged plants) pools respectively. Our three-cell canal combined dimensional size was 1.2 m (4 ft) wide, 0.6 m (2 ft) deep and 12 m (40 ft) long. The total pore volume of the CWT canal was ~5800 L, and with a DW intake of up to 908 L/day (240 gallons) coming from the aerated-FBR, met the 6-day HRT needs. The CWT cells are placed on an operational space near the aerated-FBR.
CWT cell 1: Vegetated Submerged Bed (VSB): The first CWT cell was a VSB cell filled with local river pebbles (non-carbonate rock), roughly 1-3 inch in diameter. The top 6 inches of the cell was sandy loam / pea pebble with vegetated wetland plants. The flow of DW through the cell served two purposes: a. remove BOD and TSS by sorption/ metabolism of bio-films activities; b. allow for aerobic respiration to lower pH of effluent coming from aerated-FBR at ~pH 9.
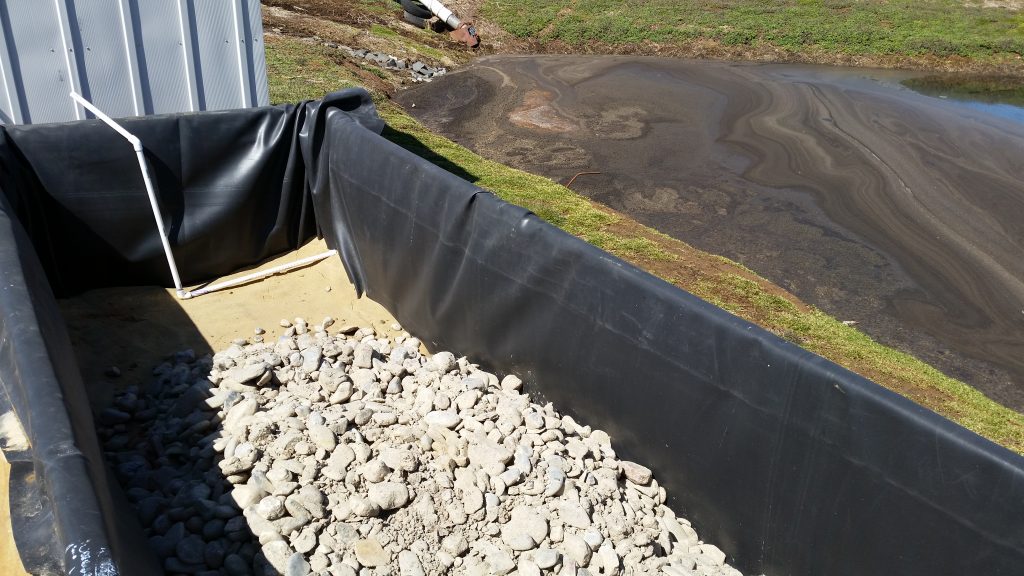
A combination of salt tolerant plants was evaluated for the VSB, including: Common Reed (Phragmites australis), Swamp Rose Mallow (Hibiscus moscheutos), Smooth cordgrass (Spartina), Alkali bulrush, Inland saltgrass, Alkaline milkweed, Soft Stem Bulrush (Scirpus validus and Common Rush (Juncus effusus). For the purpose of this study a population establishment observation accessed which plants species retained vitality over the experiment period. The parameters monitored were overall survival rate, growth and stress symptoms (leaf bleaching, dwarfish plants, survival rates).
CWT cell 2-3: Free Water Surface (FWS):
The FWS was a fully vegetated water pool with a 6-10 inch turf soil bed to support salt / pH tolerant emerging water plants: Common Reed (Phragmites australis), Cattail (Typha), Pickrel Rush (Pontederia cordata) , Sweet Flag (Acorus americana) and populated with floating Lemna minor (Duckweed) plants. The CWT was discharg through a 0.75-inch flexible PVC tube at the base of the rear wall of cell three.
Analytical methods:
To evaluate the efficiency of aerated-FBR-CWT, samples of DW effluent was analyzed prior and after treatment. The quality of fertilizer produced at the FB-reactor was evaluated based on the struvite content in the fertilizer. Prior to this study, ICP-OES analysis was used to quantify the ionic content of nutrients and pollutants. Samples were taken from DW at entry to the aerated-FBR, entry to the CWT and outflow from CWT pools 1-3 (P1,P2,P3) . Each sample was centrifuged and filtered to separate solids (0.45μm, glass fiber 25mm), then acid digested prior to analysis. Treated samples were analyzed for COD, NH4-N, NO3-N using DR3900 spectrometer (Hach) analysis kits and for PO4-P, using ICP-OES (Agilent). TSS analysis of samples was done by filtering 50 ml DW samples diluted 1:10 (1.5 μm, glass fiber 47mm, Hach) and evaporating in a furnace at 105 deg-C. The pH and effluent temperature at the FB-reactor entry, and the CWT entry and outflow were measured at the time of sampling. Solids collected from aerated-FBR were analyzed with XRD for mineralogy and with ICP-OES for elemental composition
Phosphate recovery from DW
The aerated-FBR treatment recovered up to 60% of dissolved phosphate from DW at HRT 60 min / pH 9, with 5mM Mg addition. The addition of 5mM Mg (1-g MgCl2*6H2O/L) Also improved the recovery of free PO4-P.
The figure below show the effect of Mg addition (A) on P recovery in a 7 hour run of the aerated FBR system, and P recovery form the aerated-FBR over a one-month run with 2-week sampling period (B).
Optimizing the addition of Mg was significant because it is a costly addition to the aerated-FBR process and it may increase the salinity stress in the CWT pools. The addition of 5mM was found to be sufficient for P recovery as struvite, however further study could look into method to decrease the application of Mg in the FBR process. The recovery of P from DW with the aerated-FBR has decreased over time in the continuous run, from 60-50% in the fist weeks to 10-0% in the last week, this is likely due to pH input error in the last few days of operation, resulting in actual pH drop from pH-9 optimum to pH ~8.6 and poor P recovery. The great fluctuation in dissolved P concentration correlated with DW lagoon maintenance were the lagoon was over-filling with major rain events (very low P) and drained (Very high P).
Identification of recovered fertilizer
Analysis of solids collected from aerated-FBR confirmed that the samples were mostly calcite(80-60%)/struvite(40-20%) with minor (5-10%) K-struvite components
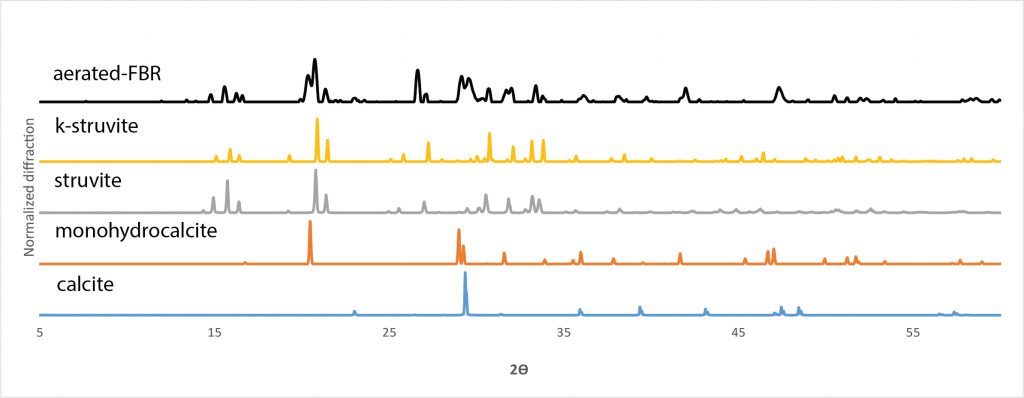
Constructed Wetland Treatment
Evaluation of CWT method was done with and without the aerated-FBR nutrient recovery treatment. The treatment results for 2-4 day HRT in CWT did not meet the treatment goals of 30/30 mg/L for TSS and BOD (~60 mg/L COD). However result showed that CWT did have a significant reduction of contaminants. Over a 1-month continuous run of either CWT, or aerated-FBR +CWT the removal rate was 60-65% for TSS and 30-35% for COD. the most significant reduction was at DW transition from VSB to 1st FWS pool and no significant COD or TSS removal accrued between 1st and 2nd FWS-CWT pools. This indicates that simply extrapolating the pools may not result in an improved treatment outcome. A modified combination of biochemical + CWT treatment is being considered to improve the CWT treatment outcome.
COD results showed that both CWT treatment (A) and CWT with aerated-FBR treatment (B) had a significant portion of COD removed over the test period.
TSS results showed that both CWT treatment (A) and CWT with aerated-FBR treatment (B) had a significant portion of COD removed over the test period.
Nutrients removal One of the goal of the CWT/aerated FBR treatment was to reduce the deposition of total phosphate species (PO4-P, Organic P and colloidal P) from DW. The treatment achieved ~60% removal for CWT and 50% removal for CWT+aerated FBR over a one-month continuous run.
Our work on the pilot farm-site found that there is a good potential for using the aerated-FBR + CWT system to recover nutrients and clarify DW. One of the key components to operate such treatment is a cost effective approach and minimizing labor input. The MyCodo farm automation software was found to be very reliable over the 1.5-year test period and is now a standard research component we use for our work. Establishing the best management practice for CWT to treat aerated-FBR effluents with high pH require further study, but both narrow-leaf cattail, wide-leaf cattail and smooth cord-grass plants showed good tolerance for high pH. Further research will look into way to lower effluent pH by promoting aerobic respiration as a pre-treatment for CWT.
Education & Outreach Activities and Participation Summary
Participation Summary:
The finding were presented at:
- Poster session presentation at the Our Farm Our Future national SARE and NCAT, ATTRA meeting, Saint Louis Missouri on April 2018.
- Two on-farm tours (July, September 2018) at Fulper farm (NJDEP, General public field day). First farm tour included members of the Bureau of Environmental Analysis, Restoration and Standards and local NRCS personnel (~8 participants). Second farm tour included local members of the farming community, NJDEP, Academic faculty from Rutgers, NJIT and CCNY and USDA personnel (total ~25 participants).
- A national meeting of the American Chemical Society (ASC) through an oral presentation session (Fall 2018) with ~15 researchers from the environmental and geochemical research community.
- Princeton agricultural society October meeting 2018. Ongoing work was presented to ~25 members of the Agricultural society.
- Environmental engineering course at City College of New York (Dec 2018), work was presented to a class of 35 college students.
- Soil Science Society of Israel annual meeting, 6.2019, Ben Gurion University, Sedeh Boker, Israel. The aerated-FBR system was presented to a panel of 40 researchers and service providers.
Future presentation are scheduled for:
- Present finding from current study and a follow-up study on P response for struvite/calcite fertilizer at NEPPSC meeting 1.2020
- Present the farm automation method at NOFA-NJ annual meeting 2.2020
Research papers:
Research work from aerated-FBR + CWT treatment is being processed into a peer reviewed and open source scientific publication
Technical training materials:
- A training webinar is currently being produced to assist implementing the micro-controller developed for GNE-158 into general agricultural sustainability practices.
- A best management recommendations document is currently being prepared for applying aerated-FBR and CWT for DW treatment.
Follow up research collaboration:
- A Follow up study is looking into the application of struvite/calcite fertilizer from aerated-FBR in tropical soils at the Universidade Federal de Viçosa, Brasil.
- A local study is looking into crop P response with Corn, Soy and Rye for aerated-FBR solids, Rutgers Agricultural extension, New Brunswick NJ
Project Outcomes
This project has promoted the awareness of DW producers, DW service providers and Environmental Sustainability policymakers to the potential of reducing nutrients deposition from small livestock operations using the aerated-FBR + CWT method, through on-site demonstrations in farm site tours and by presenting this work to a wide crowd of researchers and the local agricultural community.
An in-depth analysis of the potential economic impact of the aerated-FBR method is currently being evaluated.
The outcome of the study objectives were:
Objective 1: Assessment of DW properties to determine aerated-FBR-CWT requirements:
The Pilot scale site was able to demonstrate the feasibility of using a low cost open source reactor to automate nutrient recover from DW. The use of 5mM Mg and HRT of 1-hour was found to suffice for P recovery.
Objective 2: Produce struvite fertilizer for crop production by recycling nutrients from DW:
The aerated-FBR has produced a struvite-calcite fertilizer with high plant-available P content. The use of this fertilizer for growing corn, rye and soy in a three season crop cycle in currently being evaluated. A potential application of the struvite/calcite fertilizer as a lime source for low soil pH is being evaluated.
Objective 3:Implement CWT to meet primary treatment water discharge requirements:
The pilot CWT method hes significantly reduced the levels of TSS and COD, however the treatment values were still high and far from the effluent discharge requirement for TSS and COD. Further study is needed to improve the CWT outcome. The use of CWT to treat DW is favorable to other treatment method since it is very cost effective and require very little labor input to operate.
Objective 4: Industry outreach: Outreach activity generated from this project has interacted with 30 researchers, 45 farmers and 25 service providers from the U.S, Canad, Isreal and Brasil. A training program for open source farm automation will expand the use of precision farming in farm operation. The system is currently n the designing stage to be installed in an ag-extension research site and in one new nursery farm site. 12 local farmers and 2 service providers has expressed interest in installing this system in their farm.
Objective 5: STEM outreach: One undergraduate student worked through REU, a summer research program at Rutgers University on developing a research project looking into improving DW nutrient recovery with bio-char treatment.
Objective 6: Scientific and public outreach: Publication and presentation: Result from this study are under preparation to be published in a peer reviewed journal. This work was presented at the SARE OFOF national meeting, local and regional meeting and in an international forum.
The work done on this project has expanded our research group awareness to the unique sustainability needs of nutrients recovery for livestock producers. As a group We previously worked on the geochemistry of nutrients recovery from crop and municipal waste. The complexity of the livestock waste was a changeling and an educating experience. As an expansion of this work, We are currently collaborating with a Brazilian team on evaluating the use of the recovered nutrients in tropical soils, Evaluating locally the use of the recovered nutrients as a phosphate source for Soy, Rye and Corn, and evaluating enhanced phosphate recovery by combining a biochemical treatment with the aerated-FBR-CWT system.
Information Products
- Reclamation of Nutrients and Irrigation Waters from Livestock Wastewater (Conference/Presentation Material)