Final report for GNE17-160
Project Information
In the state of Maryland, the adoption of winter cover crops (CCs) is very high due to the Maryland Cover Crop Program (MCCP), which provides farmers with incentives to help offset CC expenses. The main goal of the MCCP is to enhance soil N scavenging and reduce nitrate loading into the Chesapeake Bay; the impact of CCs on N cycling in the subsequent cash crop is not emphasized by the program. Farmers are aware that grass CCs increase N fertilizer requirements due to N immobilization. However, farmers may not know how much N is being released or immobilized by their CC and end up applying more or less fertilizer than required by the succeeding main crop. Over-application undermines the goals of the MCCP, while under-application may reduce yields and discourage future CC use. Thus, it is critical to adjust N fertilizer application based on N availability from CC residue decomposition to optimize N use efficiency and corn (Zea mays L.) performance.
This project aimed to tighten N cycling within soil-crop systems by identifying strategies to maximize nitrate leaching reductions and assisting farmers with N management decisions in CC-based no-till corn systems. We found that non-legumes can reduce nitrate leaching by 56% and their effectiveness is enhanced with early planting dates, higher biomass, and in drier years. The decomposition of CC residues was investigated by conducting experiments at various temporal (diurnal to seasonal) and spatial (laboratory to regional) scales to better understand the relative impact of various factors regulating N mineralization from surface CC residues in no-till systems. At the regional scale, the rate of surface residue decomposition was more influenced by residue quality than the climate in the mid-Atlantic and Southeastern US states. The decay rate constants decreased with increasing C:N, residue holo-cellulose concentrations, and lignin:N, but increased with increasing residue carbohydrate concentrations. Among the climatic variables, the decay rate constants were more influenced by air relative humidity and the number of rainy days than by cumulative rainfall, suggesting faster decomposition of surface CC residues in humid environments and in years with frequent rains. Even at the diurnal scale, we found that the rate of decomposition of surface CC residues followed the diurnal pattern of residue moisture (θg) or residue water potential (ψ), thereby highlighting the centrality of residue moisture in regulating decomposition. Our laboratory experiment on dry-wet cycles further confirms these findings. All these results suggest that the accuracy of existing decision support tools can be improved by adjusting decay rate constants based on residue environment (residue ψ and temperature). A 150-d controlled incubation experiment was conducted to understand the interactive effects of residue ψ and temperature on C and N mineralization from surface CC residues. A two-dimensional response surface model was developed and was successfully integrated into existing decision support tools such as the CERES-N (Crop Environment REsource Synthesis). Calibration and validation of the CERES-N model at the regional scale is currently on-going using data from the multi-state inter-site litter-bag decomposition studies conducted in the mid-Atlantic and Southeastern US states.
Once well-calibrated and well-validated, the CERES-N model will be converted into a decision support tool that farmers, agricultural professionals, and researchers can use to predict the longevity of CC residues and subsequent N release in CC-based no-till systems. Such a tool will assist them in making N management decisions and optimize agroecosystem services from CC residues. Therefore, this project will contribute to the increased adoption of CCs as more farmers and agricultural professionals become proficient in the use of this new decision support tool. Findings from this project not only impact the performance of CC-based no-till corn systems at the farm level but also support the goals of the MCCP and NE-SARE through increased cover crop adoption at the state and regional levels, respectively.
The specific objectives of the proposed project are to:
(1) Assess the ability of CCs to scavenge soil N using a meta-analytic approach.
(2) Determine the interactive effect of moisture and temperature on C and N mineralization from surface-left CC residues.
(3) Determine the effect of dry-wet cycles and residue placement on C and N mineralization.
(4) Investigate the factors controlling decomposition and concomitantly, N release from surface-left CC residues in no-till corn systems.
Winter cover crops (CCs) play a critical role in nitrogen (N) management, both as N scavenger while growing and as N supplier to the succeeding main crop while decomposing. The ability of CCs to retain soil N in the fall is largely affected by CC species, growth stage and quality at termination, establishment methods and dates, and accumulated growing degree days. In general, non-legume CCs have faster growth rates, making them excellent at N scavenging and reducing nitrate loading into the Chesapeake Bay as compared to legume CCs. Similarly, early establishment and more accumulated growing degree days maximize CC shoot and root growth, thereby enhancing the N scavenging potential of CCs. The N scavenged by CCs during growth can be released back to the succeeding main crop. Many farmers believe non-legume CCs immobilize soil N and make it less available to the main crop, while legume CCs supply N to the main crop during decomposition. However, they often do not know how much N is being immobilized or released by their CC residues. Farmers, therefore, find it very difficult to manage N for the succeeding main crop. The ability to estimate soil N availability during CC decomposition could support high cash crop yields while minimizing N losses by allowing N management decisions to be tailored to cash crop needs.
Cover crop decomposition and subsequent N availability to the main crop depend on the quantity (biomass) and quality (N content, C:N ratio, chemical composition) of CC residue at termination, residue placement, climate, and the activity of decomposer communities. Because of these multiple interacting factors, it is difficult to accurately predict soil N availability from CC decomposition. One approach is to use dynamic simulation models such as CERES-N model to estimate N mineralization from CC residues. So far, the CERES-N model has been shown to work best for incorporated residues but often do not accurately predict N release from surface-left CC residues because moisture and temperature fluctuate greatly in surface CC residues. This model requires data on CC biomass, residue quality, and climate as inputs. Although corn is one of the most common and widespread cash crops, the calculator has not been validated in or calibrated for use in corn systems with surface-left CC residues. Therefore, there is a critical need to calibrate and redefine calculator algorithms to predict soil N availability from surface-left CC residues and adjust N applications to more precisely match corn N demand.
Cooperators
- (Researcher)
- (Educator and Researcher)
Research
I performed a meta-analysis, controlled laboratory, and on-farm experiments to address the objectives of this project. Objective 1 of this project used a meta-analytic approach. The details of the methodology and the findings of the meta-analysis were published in a peer-reviewed journal. In brief, a literature search was performed in the ISI Web of Science database to find articles that compared nitrate leaching between CC and no CC treatments. In total, we found 28 relevant articles that were ultimately included in the analysis. Data were extracted and compiled in an Excel sheet. The multi-level mixed-effects meta-analytic model was performed to investigate the effects of CC on nitrate leaching using the nlme package in R. The effect size of CC on nitrate leaching was calculated as the natural logarithm of the response ratios and the results of the analysis were back-transformed for ease of interpretation. We also performed moderator analysis to investigate the effects of covariates (non-legume category, planting dates, shoot biomass, and relative precipitation) on the mean effect size of non-legumes on reducing nitrate leaching.
To address objective 2, we conducted a 150-d controlled laboratory experiment in a customized air-flow system designed within a CONVIRON E7/2 growth chamber to determine the interactive effects of moisture (Ψ : -10.0, -5.0, -1.5, and -0.03 MPa) and temperature (15, 25, and 35 °C) on C and N mineralization from surface-applied CC residues. Surface soil (0-10 cm) samples were collected from a commercially managed corn field at the USDA ARS Beltsville Agricultural Research Center in Beltsville, MD, USA. Field moist soil was sieved through 4-mm sieve, the roots were manually removed, and the soil was homogenized. The soil was then air-dried, ground, and passed through a 2-mm sieve, then stored at room temperature until the start of the experiment. Cover crop residues used in this experiment consisted of two CC species harvested at different growth stages: (a) late-killed crimson clover (flowering stage), (b) late-killed cereal rye (flowering stage), and (c) early-killed cereal rye (tillering stage). Soil and residues with specific water potential (ψ) were prepared. To prepare microcosms, soils with specific ψ (50 g oven-dried equivalent) were packed in 100 mL plastic beakers (5.0 cm i.d.). Cover crop residues with a given ψ were surface-applied at a rate of 3000 kg ha-1 on soils with the same ψ. Cover crop residues were composed of both leaves and stem mixed in a definite proportion. An un-amended soil as control was kept for each moisture-temperature combination.
Throughout the 150-d incubation period, headspace gas samples were collected and analyzed for CO2 and N2O concentrations using a Varian GC450 gas chromatograph (Agilent Technologies, Santa Clara, CA). The microcosms were also destructively sampled at 15, 30, 60, 100, and 150-d after the initiation of the experiment and analyzed for total inorganic N (NH4+ + NO3-) contents. The microcosms were extracted with 500 mL of 1 mol L-1 KCl (at a 1:10 soil:extractant ratio) after overnight shaking in 1-L plastic bottles. The KCl extracts were analyzed colorimetrically for NH4+ and NO3- contents using a SEAL AutoAnalyzer 3 High Resolution (SEAL Analytical Inc., Mequon, WI). Net N mineralized was calculated for each microcosm by summing the total inorganic N contents and cumulative N2O emissions during that period. To determine the temporal dynamics of C and N mineralization from surface-left CC residues, the total CO2 emitted and net N mineralized from each residue was fitted to first-order rate kinetic model using nlme package in R . The interactive effects of ψ and T on C or N mineralization from surface-left CC residues was modeled by calculating the residue moisture-temperature reduction factor (MTRF). The MTRF at each ψ and T combination was calculated by dividing the total C or N mineralized in 150-d at a given ψ and T conditions by the maximum value (i.e., total C or N mineralized at optimum conditions of -0.03 MPa and 35 °C). Therefore, MTRF represents the normalized or relative amounts of total C or N mineralized from CC residues when compared at optimum conditions. The MTRF ranged between 0 to 1 and allowed comparison across residue types. Response surface equations were developed relating MTRF to residue environmental conditions (ψ and T) for use in computer simulation models.
Objective 3 of this project was addressed by conducting a series of diurnal experiments in fields and a controlled dry-wet experiment in the laboratory. Diurnal variations in red clover and cereal rye decomposition rates were determined by measuring residue CO2-C flux at approximately 0:00, 6:00, 9:00, 12:00, 15:00, 18:00, and 21:00 hours. The CO2-C flux from red clover residues was measured at 4 (June 3-5, 2019), 10 (July 18-20, 2019), and 16 (August 29-31, 2019) weeks after termination. Similarly, the CO2-C flux from cereal rye residues was measured at five (Jun. 14-16, 2019) weeks after termination using a portable EGM-4 infrared gas analyzer system (PP Systems, Amesbury, MA) equipped with a flow-through closed SRC-2 Soil Respiration Chamber (PP Systems, Amesbury, MA). At the time of CO2-C flux measurements, CC residues from an equivalent area (~78 cm2) were cut and transferred to a beaker. The chamber tops were placed on beakers and sealed perfectly to measure CO2-C flux from residues within 300 sec. This method was preferred because it allowed us to capture the actual decomposition rates of surface residues in no-till systems. Following CO2-C flux measurements, CC residues were transferred into plastic zippered bags, sealed, and brought back to the laboratory to measure residue ψ and residue θg within 1-2 h. In addition, we collected surface soil samples (0-5 cm depth, 2-cm diameter probe) from the same place where the residue samples were taken for CO2-C flux measurements. Measurements (residue and soil θg, residue ψ, and residue CO2-C flux) were replicated four times.
During each study period, air temperature and RH were monitored at 75 cm above the soil surface using a T9602-3-D-1 Humidity & Temperature Probe Sensor (Amphenol Advanced Sensors, Mansfield, TX) and recorded at 10 min intervals using a customized Arduino-based datalogger (company, city, state). A 107-Temperature Probe Sensor (Campbell Scientific, Inc., Logan, UT) was inserted inside the CC mulch layer to measure residue temperature. Soil temperature and volumetric moisture content (θv) were measured by installing a time-domain reflectometer TDR-310S probe sensor (Acclima, Inc., Meridian, ID) at a 45° angle beneath the residue layer. Surface CC residue temperature, soil temperature, and soil g were recorded at 10 min intervals using a CR1000 datalogger (Campbell Scientific, Inc., Logan, UT).
The effect of dry-wet cycles (i.e., the effect of rainfall pulse) on residue decomposition was investigated in an 80-d incubation experiment performed in a similar manner as described above. Treatments consisted of a factorial combination of two CC residues (crimson clover or cereal rye) and two residue placement strategies (surface-left or incorporated). A soil-alone control treatment was also included. Microcosms were subjected to four dry-wet cycles in a set of CONVIRON E7/2 growth chambers (Controlled Environment Limited, Winnipeg, MB, Canada) at 25 °C. In each dry-wet cycle, microcosms were dried for 16 d in a drying chamber. At the end of each drying period, 12 mL of double deionized water was quickly added using a pipette to raise θg from 0.02 to 0.26 g H2O g-1. Microcosms were then transferred to an identical high-humidity chamber (hereafter, referred to as a wetting chamber) and maintained at field capacity for 4 d.
The C mineralized from residue treatments was determined by measuring CO2 fluxes throughout the incubation period in a similar manner as described above. Similarly, three microcosms were destructively sampled at the end of each drying and wetting period and analyzed for inorganic N contents. At the end of four dry-wet cycles, microbial DNA was extracted from both soil and residue samples using the Qiagen DNeasy PowerLyzer PowerSoil and PowerPlant kit (Qiagen, Hilden, Germany), per the manufacturer’s recommendations. Prokaryotic 16S rRNA gene and fungal ITS gene regions were targeted using the primers 515F/806R and ITS1f/ITS2r, respectively. Illumina sequencing was performed according to the 16S metagenomic sequencing library preparation manual (Part number 1504423 rev. B, Illumina, Inc.). Samples were pooled; the amplicon library size was verified using a Bioanalyzer 2100 DNA chip (Agilent Technologies). The library was quantified using qPCR and the final library was run on an Illumina MiSeq using a 600-cycle v3 cartridge.
Objective 4 of this project was addressed by conducting multi-state inter-site litter bag decomposition studies in farmers' fields. We established a network of CC adopters across the mid-Atlantic and Southeastern US states. At each farm site, the experiment was conducted in a strip-plot design with CC and no CC as adjacent treatments. There were two subplots within each strip-plot. Farmers were required to leave a strip of bare soil at the time of CC planting or terminate a strip of CC within 10 d after its planting using herbicide. All other CC and cash crop management decisions were dictated by the farmers. They were free to plant any CC species and mixture composition and have flexibility in their preferred CC planting and termination dates/methods. The only requirement to get enrolled in this project was to leave the CC mulch layer on the soil surface and plant subsequent cash crops using no-till methods. In total, CC decomposition studies were performed in 105 no-till corn fields during 2016-2019 across five states (Fig. 1): Georgia (GA), North Carolina (NC), Delaware (DE), Maryland (MD), and Pennsylvania (PA).
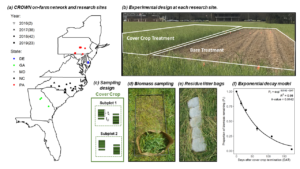
Decomposition kinetics of the aboveground CC biomass was determined using nylon mesh litter bags (26 by 60 cm dimensions, 1-mm mesh size). Just prior to termination, CC biomass was collected by clipping two separate 1 m2 areas in each of the two subplots. The fresh weight of CC biomass from each subplot was recorded and was subsequently used for filling 12 litter bags. In total, 24 litter bags were prepared at each farm site which provided four sets of six litter bags. Out of six litter bags, one bag was retained on the same day and then dried at 60 °C for at least two days to determine residue θg. The remaining five bags were deployed on the soil surface using landscape staples and were collected at approximately 14, 30, 60, 90-120 d after CC termination, and finally at corn harvest at physiological maturity. Retrieved litter bags were oven-dried at 60 °C for at least two days and the weights were recorded after scraping any soil adhered to the outside of the bags. Residues from each litter bag were ground to pass through a 1-mm sieve. Soil contamination was determined by ashing 1-g subsample of ground residue at 550 °C for 4 h. The residue weights were then adjusted to an ash-free basis. We used non-linear mixed-effect models to model CC residue decomposition as a function of the proportion of initial ash-free CC dry weight remaining in litter bags over time. A single-pool first-order exponential decay model was fitted separately for each site-year using the R nlme package to determine residue decay rate constants (i.e., k-values).
A 1-g subsample of ground residue from each bag was analyzed for tissue C and N concentrations using dry combustion via Leco TruMac CN Analyzer (LECO Corporation, St. Joseph, MI). A 5-g subsample from time 0 bag from each subplot was analyzed for residue C chemistry [carbohydrate, holo-cellulose (cellulose plus hemicellulose), and lignin concentrations] via near infra-red reflectance spectroscopy (NIRS) using a scanning monochromator (model 6500; FOSS NIRSystems, Silver Spring, MD). Soil texture was determined using the hydrometer method. Climatic data were imported from the in-house weather API (Application Programming Interface) developed for this on-farm project. Our weather API contains hourly weather data generated by the North American Land Data Assimilation System Phase-2 (NLDAS-2) and hourly rainfall data generated by Multi-Radar Multi-Sensor System (MRMS). The hourly data were summarized to calculate cumulative rainfall, the number of rainy days, mean daily air temperature, and mean daily RH.
Simple linear regressions were fitted between k-values and individual controlling factors to show their independent effect on CC residue decomposition. To account for non-normality and heteroscedasticity of the residuals, the k-values were naturally log-transformed before performing regression analysis. We performed a correlation analysis to assess collinearity among factors controlling k-values. Three stepwise multiple linear regression models were fitted to best predict log-transformed k-value: model I included only intrinsic factors [latitude, soil (sand and clay content), and climate (cumulative rainfall, number of rainy days, mean daily air temperature, and mean daily RH)]; model II included only extrinsic or management factors [CC quantity (biomass) and quality (C:N, residue carbohydrate, holo-cellulose, and lignin concentrations, and lignin:N)]; and model III included both intrinsic and extrinsic factors. The best subset of predictors among intrinsic and extrinsic factors in predicting k-value was identified using the Bayesian Information Criterion (BIC) penalty for each added predictor in the model. Models with the lowest BIC value were selected. Finally, commonality analysis was performed to assess the relative contribution of the factors selected via stepwise multiple linear regression procedure in explaining the model total variance, i.e. R2. In commonality analysis, the contribution of each regressor was assessed in terms of unique effects and in the presence of other independent variables, i.e. common effects.
Objective 1: The effect of cover crops (CCs) on nitrate leaching reductions and crop yields was investigated using a meta-analytic approach (For full details: R. Thapa, S.B. Mirsky, and K.L. Tully. 2018. Cover crops reduce nitrate leaching in agroecosystems: a global meta-analysis. Journal of Environmental Quality. 47:1400-1411.). We found that non-legumes consistently reduced nitrate leaching by 56% compared to no cover crop controls (Fig. 1). Legumes and mixtures, however, can reduce nitrate leaching during growth, but release N quickly following termination as a result of low C/N ratio in their residues. The effect of non-legumes in reducing nitrate leaching was less impacted by soil texture, soil organic C, and soil tillage. The most influencing variables on the effectiveness of non-legumes in reducing nitrate leaching were CC planting dates, shoot biomass, and relative precipitation (precipitation relative to the long-term normal precipitation for a particular site). We found that delaying CC planting dates reduced the ability to reduce nitrate leaching, probably because of the shortened growth period and concomitantly decreased biomass (shoot and root) yields and N uptake as compared to early-planted cover crops. Supporting this hypothesis, we found that the efficacy of non-legumes in reducing nitrate leaching was positively correlated with the shoot biomass at termination. The quadratic curve provided the best fit: nitrate leaching reductions with non-legumes plateaued at biomass levels of 2-4 Mg ha-1. We further found that the effectiveness of non-legumes in reducing nitrate leaching decreased with increasing relative precipitation, suggesting greater efficacy in dry years as compared to wet years. Overall, this meta-analysis confirms many prior studies showing that non-legumes are an effective means to reduce nitrate leaching and should be integrated into cropping systems to improve water quality.
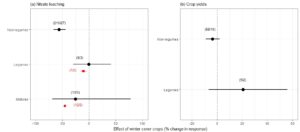
Objective 2: Besides nitrate leaching reductions, CC residues can supply all or part of the N required by the subsequent cash crops. The N mineralized or immobilized during the decomposition of CC residues in no-till systems is highly influenced by residue water potential (ψ) and temperature (T). At all ψ and T combinations, the temporal dynamics in C and N mineralized from surface-left CC residues were adequately described by first-order rate kinetic models. Early-killed cereal rye and crimson clover (low C:N ratio) residues decomposed quickly and mineralized N, whereas, late-killed cereal rye residue (high fiber content and C:N ratio) immobilized N. Irrespective of residue type and kill dates, the normalized values of C and N mineralized from surface-left CC residues increased exponentially with increasing ψ from -10.0 to -0.03 MPa. Increasing T from 15 to 35 °C further amplified the effect of ψ, suggesting a strong interactive effect of ψ and T on C and N mineralization from CC residues. This was probably due to greater enzymatic breakdown of polymers at higher T, greater solubility and diffusivity of the substrate at higher ψ, and hence, greater substrate availability for microbial decomposition. Mathematical equations were developed to describe these interactive effects (Fig. 2). These equations were successfully integrated into the existing computer simulation models (e.g., CERES-N) to simulate the effect of residue environment on decomposition and N mineralization from surface-left CC residues in no-till systems. Calibration and validation of the CERES-N model is currently under-going.
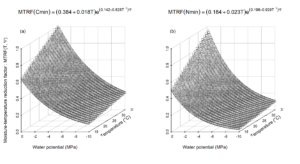
Objective 3: Our results showed that surface-left CC residues in no-till fields experienced extreme diurnal fluctuations in moisture (i.e., 𝜓) and temperature as compared to the underlying soils. The decomposition of surface-left CC residues also showed distinct diurnal patterns that were strongly related to residue θg or ψ (Fig. 3). At a diurnal scale, residue CO2-C flux increased during the nighttime from 18:00 to 06:00 h when residues gain moisture from the air and decreased during the daytime from 06:00 to 18:00 h when residues lost moisture to the air via evaporation. Increases in residue temperature, however, decreased residue CO2-C flux because microbial activity was limited by moisture availability at elevated temperatures in fields. Therefore, we conclude that the decomposition of surface-left CC residues in no-till fields is strongly influenced by residue ψ, which in turn depends on environmental conditions (air relative humidity, temperature, rainfall, and dew) and residue water retention properties.
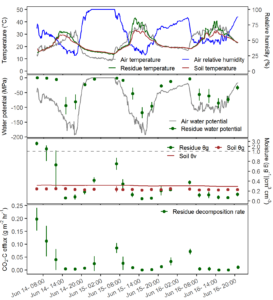
The effect of dry-wet cycles on microbial community structure, microbial diversity, and microbial processes related to C and N cycling under different residue management strategies was determined in an 80-d laboratory study. We found that residue of crimson clover had higher water storage capacities than cereal rye, and the rate at which water was lost from surface-left residues increased after each successive wetting event. Rapid drying of surface-left CC residues quickly suppressed CO2 fluxes, which increased immediately upon rewetting (Fig. 4). This result further highlights the centrality of residue moisture in controlling decomposition. As compared to surface-left CC residues, incorporated CC residues maintained water content for a longer duration and were more colonized by soil prokaryotes and saprophytic fungi. Thus, significantly more C and N were mineralized from incorporated CC residues than from surface-left residues (p < 0.05). Micro-climatic conditions that keep CC residues moist for a longer duration, such as frequent light rains, promote decomposition of surface-left residues in no-till fields.
Our results demonstrate the strong influence that residue chemistry has on soil prokaryotic and fungal diversity, community structure, and functionality. While crimson clover-amended soils (N-rich environments) were dominated by copiotrophic taxa, oligotrophic taxa were associated with cereal rye-amended soils (N-poor soil environments). On the other hand, residue location may have a greater effect than residue type on determining residue microbiota, particularly prokaryotes. Fungi were observed to be the primary decomposers of surface-left CC residues, which was probably due to their ability to form extensive hyphal networks that allow them to exploit resources from both soil and surface residues. Based on these results, we conclude that the frequency of dry-wet cycles, residue type, and residue location will differentially affect residue water dynamics (abiotic) as well as soil and residue microbiota (biotic), which in turn significantly affect microbial processes related to C and N cycling in cropping systems.
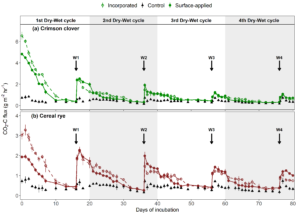
Objective 4: Our multi-state inter-site litter bag studies provided new insights into the factors controlling CC residue decomposition in no-till corn systems in the mid-Atlantic and Southeastern US states. The residue decay rate constants (i.e., k-values) increased along the latitudinal gradient. As latitude increases, climatic variables that keep CC surface residues moist for prolonged periods also increase. Among climatic variables, the mean daily RHair and number of rainy days had a stronger influence on k-values than cumulative rainfall. These results indicate faster decomposition of CC surface residues in humid environments and in site-years with more frequent rain events. Mean daily air temperature, on the other hand, had no significant effect on k-values. A clear separation of k-values between low and high latitude sites was observed at CC biomass < 4000 kg ha-1, above which the inter-site differences on k-values caused by climate were less obvious. Above 4000 kg ha-1, as CC residue thickness increased, the top residue layers likely maintained the optimal water content for decomposition at the soil-residue interface for a longer duration, thereby compensating for the decrease in k-values due to a decrease in the proportion of biomass that is in contact with the soil. We further demonstrated that the k-values decreased with C:N, residue holo-cellulose concentrations, and lignin:N, but increased with residue carbohydrate concentrations. All these results suggest that CC surface residue decomposition in no-till corn systems was limited by moisture and N availability to decomposing microorganisms. The overall best model that has CC C:N and residue holo-cellulose concentrations as quality variables and mean daily RHair and number of rainy days as climatic variables provided the best predictive model for k-values (70% variance explained). This empirical model can be used to predict the longevity of CC residues in no-till corn systems and assist farmers and land managers with CC residue management decisions to better achieve targeted agroecosystem services under present and future climate change scenarios. Results further suggest that CC residue quality has greater control over k-values than does the climate in mid-Atlantic and Southeastern US states. Based on our findings, we conclude that residue C chemistry (residue holo-cellulose concentrations) and climatic (RHair and rainy days) variables, not commonly considered in CC residue decomposition models, controlled k-values. Thus, currently available proceed-based or mechanistic models predicting CC surface residue decomposition in no-till systems need updating.
Provisioning of agroecosystem services from cover crops (CCs) depends on the quantity and quality of CC residues and the rate at which they decompose (i.e., k-values). While the quantity and quality of CC residues at the time of termination depend on CC management decisions (CC species selection and mixture composition, CC planting and termination dates/methods) and climate during CC growth period, the longevity of CC residues during the cash crop growing season depends on the rate of decomposition. We found that non-leguminous CCs are excellent at nitrate leaching reductions (on average by 56%). Policy-makers should encourage growers to plant CCs early to achieve higher shoot and root biomass for optimal N scavenging. The decomposition of CC residues in no-till systems depends on: CC residue quantity and quality, micro-climate (residue moisture or water potential and temperature), and the activity of decomposer communities. Our multi-state inter-site litter bag studies suggest that k-values decreased with increasing biomass, C:N, residue holo-cellulose concentrations, and lignin:n, but increased with increasing residue carbohydrate concentrations. Among the climatic variables, we found greater sensitivity of k-values to micro-climatic variables that keep residues moist for longer duration such as humid environments and frequent rains. The empirical model was developed to predict the longevity of CC residues in no-till fields using data on residue quality and climatic variables in the mid-Atlantic and Southeastern US states.
Surface residues in no-till fields are exposed to solar radiation, rain, dew, wind, and other micro-climatic conditions. As a result, the moisture or water potential (ψ) of the surface residues fluctuates dramatically at a diurnal scale in response to changes in the ψ gradient between surface residues and the air above it. Moreover, we observed that the decomposition of surface residues in no-till fields also exhibited diurnal patterns that are highly linked to residue ψ. Our experiment investigating the effect of dry-wet cycles on C and N mineralization of CC residues also highlighted the centrality of residue moisture on controlling decomposition. Therefore, we developed a two-dimensional response surface model that can be used to adjust k-values based on residue ψ and temperature for use in existing residue decomposition models such as CERES-N. The calibration and validation of CERES-N model is currently ongoing.
Education & Outreach Activities and Participation Summary
Participation Summary:
During 2018 and 2019, I conducted a workshop/ field day at the Kent County UMD Extension office, Eastern MD. Around 40 farmers/year participated in the workshop where I laid out the objectives of this on-farm research. I demonstrated the methodology of biomass sampling, soil core sampling, cover crop decomposition assay using nylon litter bag, and soil water monitoring using custom-developed soil water sensors. Many farmers in the workshop were highly interested in using the models validated with data from this project.
Two field days have been conducted so far to talk about our on-farm research efforts. Both field days were conducted in research farms at the USDA ARS Beltsville Agricultural Research Center (BARC), Beltsville, MD in co-ordination with the 2018 ASA-SSSA-CSA tri-society meeting and 2019 Northeast Cover Crop Council (NECCC) regional meeting. Participants in the field days were members of the organic and cover crop societies. During an on-farm demonstration, I had the opportunity to demonstrate the use of litter bags to assess cover crop decomposition and the use of custom-developed soil water sensor technology for monitoring the soil water in systems with and without cover crops. We had more than 100 participants at each event including farmers, researchers, ag. professionals, and extension agents.
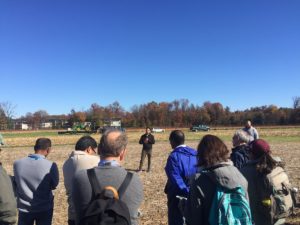
I have presented my research findings to my peers, research scientists, and farmers at regional and national conferences. Below are the titles of my talks.
- Thapa R., S. Mirsky, K.L. Tully, M.L. Cabrera, H.H. Schomberg, C. Reberg-horton, J.W. Gaskin, D. Timlin, E. Sweep, B. Davis, S. Seehvaer, E.H. Henriquez Inoa, and A. Poncet. 2019 ASA-CSSA-SSSA International Annual Meeting, San Antonio, Texas: Nov 10-13, 2019. Title: Cover crop decomposition in no-till corn fields: controlling factors. (poster)
- Thapa R., S. Mirsky, K.L. Tully, M.L. Cabrera, C. Dann, H.H. Schomberg, D. Timlin, and C. Reberg-horton, 2019 ASA-CSSA-SSSA International Annual Meeting, San Antonio, Texas: Nov 10-13, 2019. Title: Effect of temperature and residue water potential on C and N mineralization from surface-applied cover crop residues. (poster)
- Thapa R., S. Mirsky, K.L. Tully, M.L. Cabrera, H.H. Schomberg, C. Reberg-horton, J.W. Gaskin, D. Timlin, E. Sweep, B. Davis, S. Seehvaer, E.H. Henriquez Inoa, and A. Poncet. 2019 Northeast Cover Crop Council (NECCC) Annual Meeting, College Park, MD: Nov: 7-8, 2019. Title: Cover crop decomposition in no-till corn fields: controlling factors. (poster)
- Thapa R., S. Mirsky, K.L. Tully, M.L. Cabrera, C. Dann, H.H. Schomberg, D. Timlin, and C. Reberg-horton, 2019 Northeast Cover Crop Council (NECCC) Annual Meeting, College Park, MD: Nov: 7-8, 2019. Title: Effect of temperature and residue water potential on C and N mineralization from surface-applied cover crop residues. (poster)
- Thapa R., S.B. Mirsky, K.L. Tully, H. Schomberg, S.C. Reberg-Horton, J.W. Gaskin, M. Cabrera, E. Sweep, and N. Richards. 2018 Northeast Cover Crops Council (NECCC) annual meeting, State College, PA: Nov 15-16, 2018. Title: Cover crop decomposition in no-till corn fields: controlling factors. (poster)
- Thapa R., S.B. Mirsky, and K.L. Tully. 2018 ASA and CSSA annual meeting, Baltimore, MD: Nov 4-7, 2018. Title: Cover crops reduce nitrate leaching in agroecosystems: a global meta-analysis. (oral)
- Thapa R., S.B. Mirsky, K.L. Tully, H. Schomberg, S.C. Reberg-Horton, J.W. Gaskin, and M. Cabrera. 2018 ASA and CSSA annual meeting, Baltimore, MD: Nov 4-7, 2018. Title: Cover crop decomposition in no-till corn fields: controlling factors. (oral)
- Thapa R., S.B. Mirsky, and K.L. Tully. 2017 Northeast Cover Crops Council (NECCC) annual meeting, Ithaca, NY: Nov. 9, 2017. Title: Cover crops and nitrate leaching. (poster)
- Thapa R., H. H. Poffenbarger, K.L. Tully, V. Ackyord, M. Kramer, and S.B. Mirsky. 2017 ASA-CSSA-SSSA annual meeting, Tampa, FL: Oct 24, 2017. Title: Biomass production and nitrogen accumulation by hairy vetch-cereal rye mixtures: a meta-analysis. (oral)
I also gave two guest lectures to students in the UMD Dept. of Plant Science and Landscape Architecture to highlight the role cover crops play in improving agricultural sustainability. During these lectures, I presented some of the findings from this project.
- Thapa R. Nov 5, 2019. Title: Cover crops for agricultural sustainability. (Invited guest lecture in ‘PLSC 303 - Global Food Systems’ class at UMD-PSLA).
- Thapa R. Nov 5, 2018. Title: Managing soil health with cover crops. (Invited guest lecture in ‘PLSC 405 - Agroecology’ class at UMD-PSLA).
I have six manuscripts that have been either published, submitted, or are under internal review in peer-reviewed journals. The articles are:
- Thapa, R., H. Poffenbarger, K. Tully, V.J. Ackroyd, M. Kramer, and S.B. Mirsky. 2018. Biomass production and nitrogen accumulation by hairy vetch-cereal rye mixtures: a meta-analysis. Agronomy Journal. 110(4): 1197-1208.
- Thapa, R., S.B. Mirsky, and K. Tully. 2018. Winter cover crops reduced nitrate leaching in agro-ecosystems: a meta-analysis. Journal of Environmental Quality. 47(6): 1400-1411.
-
Thapa R., K.L. Tully, M.L. Cabrera, C. Dann, H.H. Schomberg, D. Timlin, C. Reberg-Horton, J. Gaskin, B. W. Davis, and S.B. Mirsky. In review. Effects of moisture and temperature on C and N mineralization from surface-applied cover crop residues. [Submitted to a peer-reviewed journal on 21 July 2020]
-
Thapa R., K.L. Tully, M.L. Cabrera, C. Dann, H.H. Schomberg, D. Timlin, J. Gaskin, C. Reberg-Horton, and S.B. Mirsky. In review. Cover crop residue moisture content controls diurnal variations in surface residue decomposition. [Submitted to a peer-reviewed journal on 02 August 2020]
-
Thapa R., K.L. Tully, N. Hamovit, S.A. Yarwood, H.H. Schomberg, M.L. Cabrera, C. Reberg-Horton, and S.B. Mirsky. In internal review. Microbial processes and community structure as influenced by cover crop residue type and location during repeated dry-wet cycles.
- Thapa R., K.L. Tully, M.L. Cabrera, B.W. Davis, D. Fleisher, J. Gaskin, R. Hitchcock, A. Poncet, C. Reberg-Horton, H.H. Schomberg, S.A. Seehaver, D. Timlin, and S.B. Mirsky. In internal review. Cover crop residue decomposition in no-till corn systems: Insights from multi-state inter-site litter-bag studies.
Project Outcomes
A journal article focused on the first objective of this project, "Cover crops reduce nitrate leaching in agroecosystems: a global meta-analysis, has been highlighted in the 2019 USDA Annual Report. Findings from this meta-analysis will help shape policies and better inform farmers to maximize nitrate leaching reductions with CCs. This project (objectives 2-4) also aimed at better understanding the decomposition and N mineralization kinetics of CC residues in no-till cropping systems. Various insights were made that will be key to improving the performance of existing decision support tools (such as the UGA Cover Crop N Availability Calculator which is based on the CERES-N model) in accurately predicting the decomposition and N mineralization of surface CC residues in no-till systems. Such tools can be used by farmers, agricultural professionals, and researchers to simulate the longevity of surface CC residues and maximize ecosystem services provisioning. For example, farmers can better manage N in CC-based no-till cropping systems, thereby achieving greater economic profitability through reduced N inputs and high cash crop yields while minimizing negative environmental impacts due to N losses from over-application. Moreover, the ability to simulate the persistence of surface CC residues during the growing season will help farmers to better manage water and weeds in CC-based no-till systems. Therefore, this project will likely change the attitude and behavior of farmers towards CCs and hence, increase CC adoption in the mid-Atlantic US. Since CCs provide a broad range of ecosystem services, our project has promoted the future sustainability of our cropping systems.
Cover cropping has long been established as a best management practice for achieving agricultural sustainability. Cover crops (CCs) are often referred to as 'service crops' because of their potential to provide multiple ecosystem services such as reducing nitrate leaching, fixing atmospheric N, weed suppression, protecting soil from wind and water erosion, breaking pest and disease cycles, sequestering soil organic C, promoting soil microbial properties, and enhancing soil health. We observed that the provisioning of ecosystem services depends on the initial quantity and the persistence of CC residues in no-till systems. Nitrate leaching reductions with non-legume CCs can be enhanced if the CCs are planted early, accumulate at least 2-4 Mg ha-1 biomass, and in drier years. The persistence or longevity of CC residues in no-till systems depends on the rate of decomposition which in turn is influenced by residue quality (C:N, residue C chemistry), micro-climate (residue θg or ψ and temperature), and the activity of decomposer communities. Through several research approaches, we consistently observed that the decomposition of surface CC residues was more influenced by residue θg or ψ than temperature. In fact, the residue θg or ψ changes more dramatically and diurnally and consequently, results in the diurnal change in residue decomposition rates in no-till systems. The most interesting finding was the strong influence of RH of the air and the number of rainy days on the rate of decomposition of surface CC residues as compared to cumulative rainfall and temperature. For the first time, we realized that the residue ψ needs to be accurately modeled based on easily available weather data. For promoting cover crop adoption among farmers and hence, achieving agricultural sustainability, existing decision support tools need to be improved so that farmers can optimize the short-term benefits of cover cropping. Our next step will be to calibrate and validate the improved version of the surface CC residue decomposition model at a regional scale using data from the multi-state inter-site litter-bag decomposition studies in the mid-Atlantic and Southeastern US. Once calibrated and validated, the model will be converted into a decision support tool that farmers can use to predict the longevity of CC residues and manage N in their CC-based no-till cropping systems. In the future, we plan to test the applicability of the tool for N management recommendations in farmers' fields.