Final report for GNE19-197
Project Information
Saltwater intrusion (SWI) is the landward movement of seawater, which can salinize coastal farmlands and affect crop yields. Germination is an especially vulnerable life history stage for crops. Salt stress reduces a seed’s ability to absorb water (osmotic stress) and causes an ion imbalance within the seed (salt stress), ultimately inhibiting germination and preventing crop production. Therefore, growing standard crops in the face of SWI can risk crop failure. If alternative crops are more tolerant of osmotic and salt stress, they may be more suited to withstand SWI. The objectives of this research were to: (1) Determine the maximum salinity level (osmotic and ionic) for 50% germination of different crop, agricultural weed, and restoration species in a controlled greenhouse experiment; (2) Evaluate the productivity of crops, agricultural weeds, and restoration species in agricultural fields experiencing saltwater intrusion.
In order to meet Objective 1, we used an incubation approach and tested the osmotic and salt stress induced by different salinity levels. We established 10 osmotic and ionic potentials (0, -0.2, -0.5, -0.8, -1.1, -1.4, -1.8, -2, -3, -4 MPa), using polyethylene glycol 8000 and sodium chloride, respectively. Four replicates of 25 seeds of each species were germinated on a filter paper in a 100 x 20 mm glass petri dish that was moistened with 3.2 mL of solution. The dishes were incubated on a diurnal cycle of 30°C for 12 hours light and 20°C for 12 hours dark. Germination counts were made in ~6 hour intervals in the first 48 hours, ~2-4 hour intervals for 2 days, then once daily until there was no additional germination for 14 consecutive days. We considered a seed to have germinated if a radicle is present. Fifty percent germination for each species at each osmotic and ionic potential was calculated based on the germination rate.
In order to meet Objective 2, we measured the effect of salinity on germination in situ. We used established experimental trials on salt-intruded fields in Somerset and Dorchester Counties, MD. The treatments were: (1) a control (agricultural weeds); (2) switchgrass (perennial species); (3) saltmeadow cordgrass (restoration species); and (4) a sorghum-chloride-excluding soybean-barley rotation with each entry point present each year (total of five treatments). Each treatment was replicated four times in a randomized complete block design. Crops were sown in mid-May. Sorghum was planted at a rate of 32,375 seeds per hectare and soybean at a rate of 78,914 seeds per hectare. Switchgrass and saltmeadow cordgrass were hand-planted as plugs at a density of four plants per meter. Final biomass from switchgrass, saltmeadow cordgrass, and control plots was harvested in October. Biomass was collected from three 0.25 square meter quadrats every 5 meters from each plot (12 quadrats per plot). Sorghum was harvested (seed head only) in September and chloride-excluding soybean was harvested in October. Harvested biomass was sorted by species, dried, weighed, and analyzed for carbon and nitrogen using elemental analysis. Two lysimeters were installed in each plot within each block (n=40) to collect pore water samples in order to measure salinity, electrical conductivity, and nitrogen and phosphorus concentrations through time.
Crop species differed greatly in germination tolerance to salt and osmotic stresses, however, there was no significant difference in percent germination between standard and alternative crop species. Standard soybean, chloride-excluding soybean, and quinoa were the only species to germinate in salt stress; standard soybean and chloride-excluding soybean had an equal tolerance during germination at -1.8 MPa (50.0 dS m-1) and quinoa successfully germinated at -0.5 MPa (13.9 dS m-1). However, wheat (standard) and barley (alternative) were the most tolerant of osmotic stress, successfully germinating at -2 MPa (55.6 dS m-1). We also examined germination of sorghum and chloride-excluding soybeans in previously-established field trials for two years on two salt intruded farms on the Eastern Shore of Maryland. One of the farms showed greater germination success (measured as seedling emergence) in chloride-excluding soybean than sorghum despite a small difference in salt stress (Δ -0.05 MPa across the field).
Our results suggest that it is not worth the money to pay for the higher-price chloride-excluding soybean, which performs poorly in research trials in salt-intruded fields. We also suggest that sorghum is a good alternative crop as it performs moderately well and has low seed and input costs. Overall, our results highlight the complexity of understanding how salt stress shapes germination processes in the real world and suggest temporal windows of low and high stress may have long-lasting consequences for crop performance.
The specific objectives of this research were to:
(1) Determine the maximum salinity level (osmotic and ionic) for 50% germination of different crop, agricultural weed, and restoration species in a controlled greenhouse experiment.
Hypothesis 1: Restoration species will have the highest ionic tolerance, followed by agricultural weeds.
Hypothesis 2: Agricultural weeds will have a higher osmotic tolerance than either crops or restoration species.
Hypothesis 3: Quinoa and barley will have the greatest threshold for salinity of the focal cash crops.
(2) Evaluate the productivity of crops, agricultural weeds, and restoration species in agricultural fields experiencing saltwater intrusion.
Hypothesis 1: Agricultural weeds will produce the greatest biomass in the field experiment.
Hypothesis 2: Porewater concentrations of nitrogen and phosphorus will be lowest under saltmeadow cordgrass and switchgrass.
Unlike other parts of the world that experience soil salinization due to irrigation water naturally high in salts, the Eastern Shore of Maryland soils are salinizing via saltwater intrusion (SWI). The low-lying topography of the Eastern Shore of Maryland (2.7 m above sea level) allows saline water to move into ground- and surface water. The rates of sea level rise in the region are three times the global average (Sallenger et al. 2012), pushing saltwater further inland each year. Although a great deal of research has focused on the effects of salinization on crop germination, yield, and plant performance (Zörb et al. 2019), SWI-induced salinization is a unique phenomenon comprised of high salinity levels and periods of saturation.
Three management strategies available to farmers battling SWI are (1) to resist change, and continue growing the standard crop rotation (corn, soy, and wheat) as long as they can; (2) to adapt to change, and grow salt-tolerant crops economically-appropriate for the region (sorghum, salt-tolerant soybean, and barley); or (3) restore the land by planting restoration species such as switchgrass or salt marsh hay. This research provides the groundwork for assessing the success of an alternative salt-tolerant cropping rotation and restoration planting on farms experiencing SWI.
Cooperators
Research
To determine the maximum salinity level (osmotic and ionic) for 50% germination of different crop, agricultural weed, and restoration species in a controlled greenhouse experiment (Objective 1), laboratory experiments were established at George Washington University (Washington, D.C.). We designed a 9 x 7 factorial experiment to test the effect of osmotic stress on seed germination. We created ten osmotic potentials using mixtures of Polyethylene Glycol 8000 (PEG) and distilled water and ten ionic potentials using mixtures of sodium chloride (NaCl) and distilled water. The ten potentials were the same for osmotic and ionic stress: 0, -0.2, -0.5, -0.8, -1.1, -1.4, -1.8, -2, -3, -4 MPa. Germination of corn (Zea mays), wheat (Triticum aestivum), soybean (Glycine max), salt-tolerant soybean, barley (Hordeum vulgare), rapeseed (Brassica napus), sorghum (Sorghum bicolor), switchgrass (Panicum virgatum), and quinoa (Chnopodium quinoa) in PEG was conducted. Salt marsh hay (Spartina patens) and common ragweed (Ambrosia artemisiifolia) were not included in the germination trial because we were unable to collect viable seeds.
When PEG has a molecular weight greater than or equal to 6000, it cannot penetrate the cell wall of seeds (Carpita et al. 1979, Verslues et al. 1998), thus mimicking osmotic stress. The concentration of PEG used to create each solution was calculated using Eq. 1 (Hardegree and Emmerich 1990).
ψ |
Eq. 1 |
Where, ψ is the water potential in MPa, PEG is grams of PEG per gram of water, and T is temperature in degrees Celsius. In this study, T=25°C because seeds in solution were kept in an incubator set to 30°C and 20°C for equal amounts of time. The concentration of NaCl was calculated using Eq. 2 (Lang 1967).
![]() |
Eq. 2 |
Where, ψ is the water potential in atm, T is temperature in Kelvin, and R is the ideal gas constant . In our case, T=25°C as the solutions were in an incubator for equal amounts of time at both 30°C and 20°C. To mimic field conditions where seeds are regularly saturated with saltwater, we placed 25 seeds per species on one 85 mm Grade 1 Whatman filter paper, 11 μm pore size, in 100 x 20 mm glass petri dishes and moistened with 3.2 mL of deionized water or PEG solution. Each species x solution combination was replicated four times (total of 100 seeds per species x solution combination). The petri dishes were tightly covered and wrapped with parafilm to prevent evaporation and were incubated in a Percival Incubator on a diurnal cycle of 30°C for 12 hours light and 20°C for 12 hours dark. If the filter paper appeared to be drying or there visibly was no solution in the petri dish, we replaced the filter paper and added 3.2 mL of solution to the petri dish. Due to the large seed size of corn, larger petri dishes and filter paper were used (150 and 125 mm diameter, respectively), and 7.8 mL of solution was added to fill the larger volume. Germination counts were made in ~6 hour intervals in the first 48 hours of experiment initiation and then in ~2-4 hour intervals for the following two days. After four days, a daily single count was made until there was no new germination observed for 14 consecutive days. A seed was considered germinated, if a radical was present.
We used germination data to calculate the time to 50% germination (Eq. 3).
Eq. 3 |
Where, t50 is the median germination time, N is the final number of germinated seeds, Ni and Nj are the total number of seeds germinated in adjacent counts at time Ti and Tj, respectively (Farooq et al. 2005). The time to 50% germination standardizes germination rates so that they are comparable among studies (Scott et al. 1984, Ranal and Santana 2006). Within a species seed lot or across different species, seeds can germinate at varying rates, and data is not normally distributed, making it challenging to draw comparisons. Time to 50% germination is a measurement that provides a central tendency to the data, which is comparable to the mean of normal distribution (Scott et al. 1984, Ranal and Santana 2006).
To evaluate the productivity of crops, agricultural weeds, and restoration species on agricultural fields experiencing saltwater intrusion (Objective 2), we used recently established experimental trials on salt-intruded fields in Somerset and Dorchester Counties, MD. Our study sites are near Crisfield, Maryland in Somerset Co. (37.983436° N, -75.854527° W), Princess Anne, Maryland in Somerset Co. (38,202655° N, -75.692893° W), Quantico, Maryland in Wicomico Co. (38.374376° N, -75.742253° W) and Cambridge, Maryland in Dorchester Co. (38.5632° N, -76.0785° W); farm fields in the region have been no-till for at least 40 years (Huggins and Reganold 2008). Saltwater moves onto agricultural fields via hydrologically connected ditch networks and the groundwater table (Tully et al. 2019). The extensive agricultural ditch network designed to drain excess water from farms often serves the reverse purpose by acting as a conduit for saltwater to reach the fields during high tides and storms (Bhattachan et al. 2018, Tully et al. 2019).
Experimental plots were established in a randomized complete block design with four replicates per treatment at farms in Somerset Co., Dorchester Co., and Wicomico Co. Treatments consist of: (1) a natural recruitment control (colonized by species in the seed bank, predominantly agricultural weeds and locally sourced species); and (2-4) a sorghum-salt tolerant soybean-barley rotation with each entry point present each year (total of three plots per block); (5) switchgrass; and (6) salt marsh hay. Each plot was 3 m wide by 20 m long and established within 2 m of the field edge with evidence of SWI. Plots were made intentionally long so as to span a natural salinity gradient, from high salinity near the field edge (0-5 m) to low salinity towards the center of the field (15-20 m). There were 0.5-1 m buffers between each plot and four replicates of each treatment for a total of 24 plots per farm.
Porous cup lysimeters (22 mm diameter; Soil Solution Access Tubes, Irrometer Riverside, California, USA) were installed to 60 cm depth at 5 m (near salt source) and 15 m from the edge of the plot (far from salt source). Lysimeters were installed using a soil probe and a slurry made with the deepest soil was poured into the hole before inserting the lysimeter to ensure good soil contact. Finally, lysimeters were sealed at the soil surface with a bentonite/clay mixture to avoid preferential flow of water down the side of the tube. Pilot studies confirmed that soil solution collection was only possible following rain events that were greater than or equal to 6 mm, thus soil solution was only collected following rain events of this level.
Switchgrass and salt marsh hay were hand planted as plugs at a density of 4 plants m-2 June 2018 for a total of 240 plants per plot. Switchgrass plugs were grown in the University of Maryland Research Greenhouse complex (var. Kanlow). Salt marsh hay plugs were purchased from Environmental Concern (Cambridge, MD).
Prior to planting, plots were sprayed with glyphosate [N-(phosphonomethyl) glycine] (~0.91 kg active ingredient per acre) in early-May 2019. Sorghum (var. Dekalb DKS 2805) and salt-tolerant soybean seeds (Pioneer P42a52x; Cl--excluder) were sown in early-June 2019 using a 1.52-m Tye drill. Sorghum was planted in 38.1 cm rows at a rate of 197,600 seeds ha-1 and salt-tolerant soybean was planted in 19 cm rows at a rate of 481,650 seeds ha-1. Sorghum received 84 kg ha-1 as urea and salt-tolerant soybean received 67 kg ha-1of K in the form of potash (K2SO4) in early-June 2019. Due to poor germination as a result of heavy rainfall, sorghum and salt-tolerant soybean were re-sown, with the same seeding practice, in late-June 2019. Barley (var. Throughbred) was planted in 19 cm rows at 1,077 kg ha-1 in mid-October.
Switchgrass, salt marsh hay, and control plots (agricultural weeds) were harvested early-October 2019. Aboveground biomass was collected along the midline of the plots from three 0.25 m2 quadrats within each 5 m segment of the plot (i.e. 0-5, 5-10, 10-15, 15-20 m) for a total 12 quadrats per plot (0-5 m is closest to the saltwater source and 15-20 m is furthest from the saltwater source). Sorghum (seed head only) was harvested in early-October 2019 and salt-tolerant soybean was harvested in late-October 2019, by running the biomass through a thrasher. Switchgrass, salt marsh hay, and agricultural weed biomass were sorted by species, dried, and weighed.
Objective 1. Across all 9 species, we observed variable tolerance to osmotic stress (Fig 1). Barley and wheat were the only species to germinate at osmotic stress levels below -1.1 MPa. However, there was no significant difference between wheat and barley percent germination at every osmotic stress level. Surprisingly, standard soybean and salt-tolerant soybean had very similar germination responses to osmotic stress. Quinoa was able to germinate to -0.8 MPa (osmotic stress) but did not have a higher percent germination at -0.8 MPa than corn, sorghum, and barley. When grouped, we found no difference in time to 50% germination between standard crops and alternative crops at any osmotic stress level.
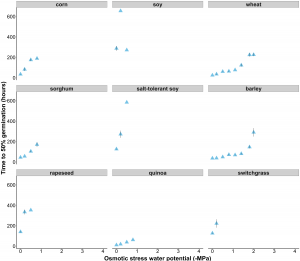
Salt-tolerant soybean, soybean, and quinoa were the only species able to germinate under ionic stress levels. Salt-tolerant soybean was able to germinate at high ionic stress levels (-2 MPa; 36.9 ppt), which is equivalent to levels found in seawater. Standard soybean was also surprisingly tolerant of ionic stress; it was able to germinate at -1.8 MPa (32.9 ppt). Standard soy and salt-tolerant soybean required significantly more hours to reach 50% germination at -0.2 MPa and -0.5 MPa compared to quinoa (Fig 2).
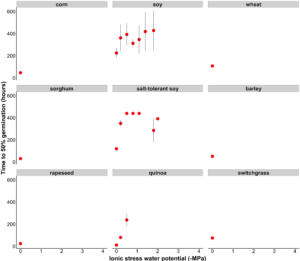
In-field sorghum germination was better explained by salt stress than osmotic stress alone (Fig 3A), suggesting that sorghum germination was more sensitive to sodium than chloride toxicity than water availability (e.g. inundation and drought). Previous research shows that sorghum has developed strategies to adapt to drought conditions (i.e., deep and extensive rooting), which aid in its success growing in semi-arid and arid regions. In contrast to sorghum, the decline in chloride-excluding soybean germination in-field was better explained by increasing osmotic stress rather than salt stress (Fig. 3B), suggesting that germinating chloride-excluding soybeans were more sensitive to reduced water availability than sodium and chloride toxicity. This is likely due to the fact that the cultivar planted was bred to exclude chloride (Pioneer Co®), making it more resistant to changes in ionic strength.
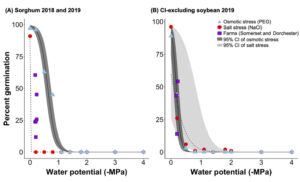
Objective 2. Harvestable biomass (kg ha-1) of switchgrass, salt marsh hay, sorghum, and salt-tolerant soybean was dried and weighed. Interestingly, at the farm in Crisfield, Maryland, switchgrass biomass increased as we moved further from the saltwater source. Sorghum at the farm in Crisfield consistently had greater biomass compared to the farm in Cambridge, Maryland (Fig 4).
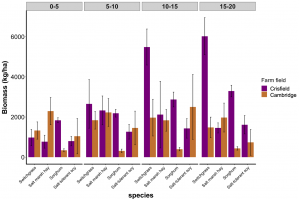
Objective 2. We wanted to determine the salinity of the porewater in established field trials and it's effect on percent germination. In 2019, Dorchester farm there was a significant difference in field germination between species, where percent germination of chloride-excluding soybean was two times higher than sorghum (p =0.001). Sorghum germination was depressed in general that year at Dorchester farm; sorghum germination in 2019 was half of the level of 2018 at the site (p = 0.0005; Table 1A & B). At Somerset farm, sorghum germination did not significantly differ between 2018 and 2019. Nor did percent germination differ between sorghum and chloride-excluding soybean at Somerset farm in 2019 (Table 1B). There was no significant effect of distance from plot edge on either species on either farm in either year.
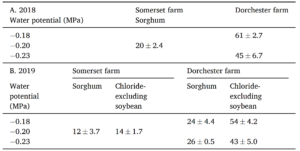
Objective 2. Determine the concentration of nitrogen in lysimeters from fields. This is part of an on-going research project. Here, we are presenting data from three farms (BN = Dorchester Farm; GR = Somerset Farm). The show large differences in dissolved inorganic nitrogen concentrations from different species types. We see fairly high nitrogen concentrations under soybeans (Fig 5).
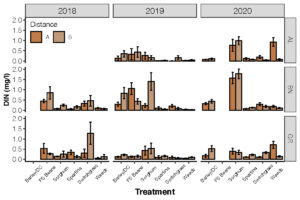
We were curious to document how quinoa would germinate under the same salinity conditions imposed on the standard and alternative crop species because quinoa is a halophyte and tolerant of salinity levels approaching those of seawater (Adolf et al. 2013, Adolf et al. 2012, Shabala et al. 2012). Furthermore, quinoa can grow in drought-prone and marginal soils (Jacobsen et al. 2003, 2005, 2007, Sun et al. 2014) such as those found on the Eastern Shore, MD. However, we did not include quinoa in the field experiment because our farmer partners stated they would not plant quinoa due to the cost of new equipment (e.g. new seed plates) and the non-existent market. Further research is needed to assess if there could be a market for quinoa in the region and if it would be advantageous for farmers to plant quinoa on their salt-intruded farm fields.
Markets on the Eastern Shore, MD support the cultivation of sorghum, salt-tolerant soybean, and barley, all of which past research indicated should have a higher salt tolerance than corn, soybean, and wheat (Rani et al. 2012, Munns et al. 2006, Munns and Tester 2008). Overall, there was no significant difference in percent germination between the standard and the alternative crop species under osmotic or salt stress in controlled environmental conditions. Under field conditions, germination success was two times higher in chloride-excluding soybean than sorghum. The in-field germination patterns of sorghum and chloride-excluding soybean were explained by increasing salt stress and osmotic stress, respectively.
Further, the fact that sorghum and chloride-excluding soybean show sensitivity to different stressors associated with saltwater highlights the complexity and challenges of identifying crop species capable of germinating on salt-intruded farm fields. Farmers facing saltwater intrusion have to decide whether to continue growing the standard crops or begin planting alternative crop species. Understanding the germination success of crop species experiencing saltwater intrusion is critical to developing informed farm management strategies in coastal agricultural regions. This research provides the groundwork for assessing the success of an alternative cropping rotation on farms experiencing saltwater intrusion.
Education & Outreach Activities and Participation Summary
Participation Summary:
On 11 Nov 2019, I presented preliminary data at the SSSA-CSSA-ASA meeting in San Antonio, Texas.
I wrote a blog post for the Sustainable, Secure Food blog, sponsored by ASA and CSSA, for my presentation at the SSSA-CSSA-ASA meeting.
Saltwater intrusion stakeholder meeting was held on 14 Feb 2020 (22 attendees). This audience consists of farmers, landowners, natural resource managers (Maryland Department of Natural Resources, Natural Resources Conservation Service, Soil Conservation District), and Extension agents. This meeting included a Public Q&A for press, local residents, etc and was well-attended (24 additional attendees).
My data was presented at the Delmarva Soil Summit in Georgetown, Delaware, which allowed farmers, scientists, and other relevant stakeholders to learn about the work I’m conducting.
My research was presented at a stakeholder meeting in 2020 where our farmer participants were able to hear how my work has been progressing on what my findings are.
My research, The effects of saltwater intrusion on germination success of standard and alternative crops was published in Environmental and Experimental Botany.
Project Outcomes
The purpose of this project is to examine the effect of saltwater intrusion (SWI) on plant germination and productivity. Saltwater intrusion, the landward movement of sea salts, is driven by sea level rise, frequency and intensity of storms and tides, drought, groundwater withdrawals, and connectivity to saline water bodies. We have observed extensive SWI on coastal farms in the Lower Eastern Shore of Maryland. As the standard corn-full season soybean-wheat rotation holds little promise as SWI moves inland due to their low salt tolerance, farmers across the Atlantic Coastal Plain face a tough decision: continue farming with the standard three-year crop rotation, switch to salt-tolerant crops, or abandon their fields and way of life. My research is testing the salinity thresholds of standard and salt-tolerant crops, as well as restoration species. This data will help inform farmer management decisions given economic and environmental objectives and tradeoffs.
This research served as the springboard for many other projects to try to help farmers and landowners on MD's Eastern Shore adapt to saltwater intrusion. We are working on several new initiatives to investigate optimum restoration species for American Black Duck for salt-damaged lands. We also applied for a Foundation for Food and Agriculture Research (FFAR) Seeding Solutions Grant to investigate the potential for new crops in the region. We are also working to develop perennial species that could serve as poultry bedding.
This project has highlighted the complexity and challenges of identifying crops capable of growing on salt-intruded farm fields as every crop responds differently at different life stages. Additionally, understanding the germination success is critical in developing farm management strategies in coastal agricultural regions.
Specifically, we founds no significant difference in percent germination between the standard and the alternative crop species under osmotic or salt stress in controlled environmental conditions. Under field conditions, germination success was two times higher in chloride-excluding soybean than sorghum. The in-field germination patterns of sorghum and chloride-excluding soybean were explained by increasing salt stress and osmotic stress, respectively.
The environmental variability of coastal environments and the interaction of hydrology and climate with SWI make it complex to understand how tolerance to osmotic and ionic components of salt stress will translate into field germination success. There is still work to do to understand how crops will respond to both salt stress and fluctuating wet-dry regimes. However, the fact that sorghum and chloride-excluding soybean show sensitivity to different stressors associated with SWI demonstrates that different crop species will have different susceptibilities that will translate into performance success. Furthermore, the performance of quinoa in the laboratory, and its high tolerance to both osmotic and salt stresses, suggests that it is worthwhile to initiate field trials with this species to investigate its viability in the region. Our study focused only on germination and not crop yield. More research is needed to identify how salt tolerance at each life history stage translates into yield effects of SWI. The field experiment described herein will run for 3+ years to have a full rotation of the alternative crops and multiple insertion points for all crops in order to disentangle the relationship between species performance, inter-annual climate variability, and SWI impacts on yield. In field experiments, we are also exploring the possibility of halophytic and perennial species, Spartina patens and others, to be grown on salt-intruded farm fields as a means of ameliorating salt damage, providing a transitional forage or biomass crop, and promoting coastal wetland restoration.