Final report for GNE19-199
Project Information
Bacterial diseases of tomato, such as bacterial speck (Pseudomonas syringae pv. tomato), are becoming more prominent in the Northeast USA due to shorter crop rotations and changes in weather events that are more favorable for disease development. Proactive management against bacterial diseases is required to reduce long-term effects on tomato growth and yield in conventional and organic production. A microbial community-based approach could improve plant productivity and is supported by both theoretical and empirical research. The inspiration for a microbial community-based approach comes from natural disease suppressive soils. Such soils have been observed to suppress the severity of soil-borne pathogens over multiple years of monocrop cultivation and are linked to the development of a microbial community that reduces disease. We hypothesized that the passaging of microbial communities (like continual crop production) in the presence of a bacterial pathogen would have a similar disease progression to suppressive soils.
The experimental approach in this research used a microbial passaging method where tomato plants are applied with a phyllosphere community, challenged with Pseudomonas syringae pv. tomato, assess for disease severity, and then plants are selected for a low disease phenotype. This passaging was completed nine times with four biological replicates. In the current study, the experimental approach of passaging a phyllosphere microbial community to select for a low disease phenotype (indicative of pathogen inhibition) suggests that passaging was successful and resulted in a significant reduction in bacterial speck disease severity over time can be achieved. These results are similar to what is known regarding certain suppressive soils.
The impact of this research is yet to be seen, but a small reduction in disease incidence and severity based on a microbial community-based approach that is amplified to a field-level could provide great benefit against foliar diseases.
In this project, I proposed to develop tomato foliar microbial communities effective at suppressing bacterial spot in tomato. I hypothesized that a community of microorganisms would provide increased plant health by preventing disease development, and result in continual disease suppression.
The specific objectives of this proposal was to:
- Develop a method for transferring communities during germination in the greenhouse. Expected outcome: This objective would identify the efficacy of foliar microbial community transfer using tomato leaflets as starting material.
- Determine if passaging of phyllosphere microbial communities could mimic the observed disease outcomes similar to disease suppressive soils. Expected outcome: This objective would establish the development of disease suppression using an experimental method.
- Assess the efficacy of plant material stored over an extended period of time for disease reduction. Expected outcome: This objective would indicate whether plant material can be stored long-term prior to application and still retain effectiveness.
Overall, this project provided a proof-of-concept for designing a more sustainable method for protecting crops from diseases in the short-term, and potentially long-term in the field. This research could provide critical data that will aid in selecting methods that could be incorporated into current growing practices in an economically feasible manner.
Tomato is an economically important horticultural crop in the Northeast (MD, PA, NJ, NY), particularly for small-scale growers, with approximately 8000 acres harvested in 2017 worth $59 million (USDA-AMS 2017). Yet, bacterial diseases of tomato, such as bacterial speck (Pseudomonas syringae pv. tomato) and spot (Xanthomonas spp.), are becoming more prominent in the Northeast region, and in particular Pennsylvania, due to shorter crop rotations and increased rainfall (Bogash, 2016). Given the nature of bacterial plant diseases and the economic necessity of growing highly susceptible cultivars, there are significant reliance on chemical (e.g. fixed copper) and antibiotic (e.g. streptomycin) control measures (Vidaver, 2002). However, such control management strategies have become less effective as resistance has emerged and spread through pathogen populations (Obradovic, Jones, Momol, Balogh, & Olson, 2004; Stall, Loschke, & Jones, 1986; Stall & Thayer, 1962). Hence, alternative approaches, such as utilizing biological control agents (BCAs), have the potential to substitute or combine with currently employed strategies to provide more robust disease control in both conventional and organic tomato production.
References
Bogash, S. (2016). Farming like you expect bacterial diseases. Retrieved from https://extension.psu.edu/farming-like-you-expect-bacterial-diseases
Obradovic, A., Jones, J. B., Momol, M. T., Balogh, B., & Olson, S. M. (2004). Management of Tomato Bacterial Spot in the Field by Foliar Applications of Bacteriophages and SAR Inducers. Plant Disease, 88(7), 736–740. https://doi.org/10.1094/PDIS.2004.88.7.736
Stall, R. E., Loschke, D. C., & Jones, J. B. (1986). Linkage of Copper Resistance and Avirulence Loci on a Self-Transmissible Plasmid in Xanthomonas campestris pv. vesicatoria. Molecular Plant Pathology, 76(2), 240–243. https://doi.org/10.1094/Phyto-76-240
Stall, R. E., & Thayer, P. L. (1962). Streptomycin resistance of the bacterial spot pathogen and control with streptomycin. Plant Disease, 46, 389–392.
Vidaver, A. K. (2002). Uses of Antimicrobials in Plant Agriculture. Clinical Infectious Diseases, 34(s3), S107–S110. https://doi.org/10.1086/340247
Research
Field microbial collections: Asymptomatic tomato leaves were collected from Russell E. Larson Agricultural Research Center at Rock Springs, Pennsylvania in late August 2020. Three leaves from 5 individual plants (1 young, 1 mid, 1 old) were selected across the tomato growing field. All plant material was stored at 4˚C for 5 days.
Greenhouse tomato growth: Mountain Fresh Plus (Johnny’s Selected Seeds, ME) tomato seeds were surface disinfested (1min in 70% EtOH; 20min in a 0.1% Tween, 5.25% bleach solution); rinse 3x with autoclaved ultrapure water (MilliporeSigma, MA)), and sown in 2” square 50 pod flats pots with non-sterile Sunshine Mix 4 soil in a greenhouse (Pennsylvania State University). Seedlings were transplanted to 6” pots 12 days after sowing. Plants were watered at soil level daily.
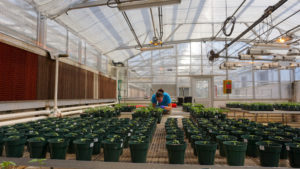
Leaf community inoculum: At least 25 leaflets from the collected material were placed in a 50 mL conical and completely submerged with 10 mM MgCl2, sonicated in an ultrasonic bath (Branson Ultrasonics Corporation, CT) for 15 minutes, and vortexed for 20 seconds. Leaflets were removed and placed in another conical, submerged with 10 mM MgCl2, sonicated in an ultrasonic bath for 15 minutes, and vortexed for 20 seconds. All leaflets were discarded. The remaining supernatant in both conicals was centrifuged for 2 minutes at 7197 x g to pellet cells. The supernatant was extracted leaving 1 mL and up to 25 mL was placed in a new separate 50 mL conical. The pelleted cells were vortexed and combined into a single conical (total of ~2 mL). Finally, the 50 mL of supernatant was added to the cells and then a misting spray bottle was filled to a 1:2 ratio with 10 mM MgCl2 (cells:MgCl2) with 0.001% Silwet L-77.
Pathogen inoculum: Pseudomonas syringae pv. tomato DC3000 (Pto) was grown in liquid King’s B (KB) media at 28˚C for 12 hours. The cells were then centrifuged at 7197 x g, washed with 3 mL of 10 mM MgCl2, and resuspended in 1 mL of 10 mM MgCl2. Bacterial suspensions were standardized to OD 600 nm at 0.3 (108 CFU) in 300 mL of 10 mM MgCl2. Cells were added to misting spray bottle with 0.001% Silwet L-77.
Passage set up: Leaf community inoculum was sprayed onto 1.5-week-old tomato plants grown in the greenhouse. Two days after community transfer, plants were challenged with Pto pathogen. After 5 days, 3 replicate plants showing the lowest disease severity from disease assessment were collected as the source microbial community for the next passage (Figure 2). Each passage is 8 days long and a total of 9 passages were completed.
A single passage consisted of 36 plants split into 4 treatments with 9 replicate plants. Treatments included leaf community and Pto, leaf community only, Pto only, and buffer only (Figure 3). Three plants of each treatment were randomly placed across 3 blocks on a single greenhouse bench. At the end of each passage, all treatments were processed individually and transferred to the next passage.
Leaf community transfer: At 1.5 weeks old, plants were misted with either leaf community inoculum or 10 mM MgCl2 buffer (Figure 2) on the abaxial and adaxial sides of the leaves until run-off at dusk. All plants were immediately placed randomly in a high-humidity chamber for 10 hours (90-100% RH). The plants were moved to a greenhouse bench under growth lights (400-watt High-Pressure Sodium Crop lights, P.L. Light, Canada) on an 8/16 light cycle and misted with tap water.
Pathogen inoculation: Three days following leaf community transfer, plants applied with either leaf community or 10 mM MgCl2 were spray inoculated with Pto pathogen on the abaxial and adaxial sides of leaves until run-off at dusk (Figure 2). All plants were placed in the high-humidity chamber for 10 hours, then moved back to the bench and misted with tap water.
Disease assessment: Disease severity was recorded on a quasi-continual scale (0-100%) and disease incidence based on the presence or absence of symptoms for all replicate plants based on visual observations at the end of each passage (Figure 2).
Plant selection and passage transfer: At the end of each passage, all leaflets of 3 tomato plants from treatments with leaf community with the lowest disease severity were collected and stored at 4˚C. Additionally, all leaves of 3 plants from treatments with buffer were randomly selected and collected. Each treatment was processed individually following the protocol outlined for the leaf community inoculum above. The prepared leaf community inoculum for each treatment was applied following the Leaf community transfer protocol from above.
Statistical analysis: Based on the experimental setup where each passaging line was independently passaged and repeatedly measured, the observed disease severity for each passage was treated similarly to that of a time-series experiment. The disease severity at the end of each passage for all treatments was transformed into area under the disease progress curve (AUPDC) values and were analyzed in a mixed model for repeated measures (MMRM) and post-hoc Tukey ANOVA multiple comparisons using JMP® Software (SAS Institute Inc, NC).
1. Develop a method for transferring communities during germination in the greenhouse. This objective would identify the efficacy of microbial community transfer using tomato leaflets as starting material.
We investigated whether it was possible to transfer a field-collected metacommunity (the combined community from multiple plants in a single field) on tomato plants germinated in the greenhouse during August and November 2020. The method we developed to transfer the leaf communities from the field and between passages of the experiment was a spray application (Figure 1). Briefly, leaf material was submerged in buffer and sonicated at a high frequency to disrupt the microbes on the leaf surface and then sprayed on naïve plants (no additional applications) in the greenhouse. At the end of each passage, the resultant plants were selected, and the microbial communities were collected and transferred using the same method.
This method was successful for transferring the leaf microbial community between tomato plants as several passages were completed and resulted in a positive outcome for objective 2. This method proved easy to apply and practical for the number of replicates (144 plants total per passage) under these experimental settings. It was also possible to keep treatments separate with minimal pathogen contamination. However, such a method may not be practical at large scales required for continual tomato seedling production. Confirming what microorganisms were transferred from the field communities and between passages using this spray application method, they will be investigated in another project that aims to sequence the field and passaged communities to gain taxonomic identification.
2. Determine if passaging of phyllosphere microbial communities can mimic the observed disease outcomes similar to disease suppressive soils. This objective would establish the development of disease suppression using an experimental method.
The passaging method of transferring microbial communities using a spray application and selecting plants for low disease resulted in a trend of disease suppression across four biological replicates (107, 108, 109, and 110) during August and November 2020 (Figure 4). This trend is based on the observed progression of disease severity following a similar trend observed in suppressive soils where disease severity increases in a field after the pathogen is present in the soil, a disease outbreak occurs, and then there is a large decline in disease severity and remains low for many seasons/years. Each passage acts as a single season of infection that would occur in the field over multiple years.
Disease severity was recorded on a continuous scale for all treatments within each passage line. Overall, the 'leaf community and Pto' treatment reduced the severity of P. syringae pv. tomato infection when disease severity data were compared with the three treatments across the series of passages in a repeated measure mixed model analysis (F = 30.138; P = 0.0001). However, the 'leaf community and Pto' were not significantly different to 'pathogen only' treatment at each passage in an ANOVA post-hoc Tukey test. For passage line C107, disease severity increased from passage 1 with a peak at passage 4 for both 'leaf community and Pto' and 'pathogen only' treatments (Figure 4A). From passage 4 onwards, disease severity declines by 30-35% by passage 10. Additionally, passages 7 to 10 have an average disease severity lower than the initial disease severity presented at passage 1 for 'leaf community and Pto' and 'pathogen only' treatments. We can observe a peak and decline in disease severity, and the decline is continuous for multiple passages similar to some suppressive soil examples. Treatments that did not introduce pathogen ('leaf community only' and 'buffer only'), remained at 0% disease severity across all passages indicating no contamination of pathogen in the greenhouse.
To determine if a passaging method could develop and enrich a phyllosphere microbial community to suppress bacterial speck disease in tomato, we observed disease intensity over time across all 9 passages. Visual observations included disease severity on a quasi-continual scale (0-100%) and disease incidence (presence or absence of symptoms) for each plant of all treatments. Disease incidence was 100% for all replicate plants of the ‘leaf community with Pto’ and ‘pathogen only’ treatments in each of the four biological replicates. Despite the high incidence levels, disease severity differed over time. There were no visible symptoms for ‘leaf community only’ and ‘buffer only’ treatments at any passage.
The average area under the disease progress curve (AUPDC) for the ‘leaf community with Pto’ and ‘pathogen only’ treatments were analyzed across all 9 passages from the observed disease severity percentages. At the end of passage 1, all biological replicates have low AUDPC values. On average, the disease begins to increase gradually until passage 3 (~150-200 AUDPC), and then disease increases to the highest values observed at passage 5 (except for ‘pathogen only’ in 109). Following passage 5, AUDPC values decrease continually to the final passage. Passage 9 disease levels return to levels similar at passage 1. Overall, only biological replicate 109 shows a statistical difference between the two treatments across the entire experiment (F = 2.58; P = 0.0087). However, when examining different time points, the two treatments are only statistically different at passages 3 and 4. This indicated that the passaging method established disease suppression like the observed disease pattern of known disease suppressive soils. Additionally, the introduction of an external field-collected phyllosphere community does not appear to have a statistical significance compared to plants that did not receive, therefore the passaging method effect could be greater than the effect of the starting community.
3. Assess the efficacy of plant material that is stored over an extended period of time for disease reduction. This objective would indicate whether plant material can be stored long-term prior to application and still retain effectiveness.
This objective could not be completed due to disruptions of the COVID-19 pandemic.
The key conclusion for this project is that the disease progression of microbial communities across 9 passages exhibited dynamics like suppressive soils; where disease pressure builds over successive passages to a peak, followed by a sharp decline in disease, and then remains low over several passages. Thus, our passaging of a microbial community demonstrates the development of a disease suppressive phyllosphere community.
The presence of an external field phyllosphere microbial community does suggest a small reduction in disease progression over time compared to the treatment that did not receive a community. Such differences may not be significant in this experiment and could become significant with a larger study. However, small differences could still have an effect on preventing high disease in the field or greenhouse as known chemical synthetic applications can also have an impact with small disease reduction.
Education & Outreach Activities and Participation Summary
Participation Summary:
Oral presentations:
- “The influence of antagonistic and suppressive traits in microbe-microbe interactions” at the Penn State University, Department of Plant Pathology and Environmental Microbiology's Fall 2020 Seminar Series
- “Cats and Mice Among the Leaves: Leveraging Microbial Competition in the Phyllosphere” talk with Dr. Kevin Hockett to the R&D department at Indigo Ag (agricultural technology company) in January 2021
- “Enriching microbial communities to suppress bacterial speck disease in tomato” at the American Phytopathology Society 2021 Plant Health Conference
- “Selecting microbial communities for disease suppression in tomato” at the 2021 American Indian Science and Engineering Society National Conference
- “Artificial selection of the phyllosphere community can suppress plant disease” at the 2021 SACNAS National Diversity in STEM Conference
Poster presentations:
“Enriching microbial communities to suppress bacterial speck disease in tomato” at the Mid-Atlantic Fruit and Vegetable Convention in February 2021
Project Outcomes
This project is just the beginning of investigating suppressive phyllosphere microbial communities and how this can be applied to vegetable crop disease management in the greenhouse and field. The proof-of-concept method used showed that a microbial community can be enriched to suppress disease with or without a field-collected community. There are several steps that will need to be completed to assess the use of such communities such as larger trials, testing in the field, identification of communities, testing against multiple pathogens, application methods and procedures, etc. Researchers and grower input will be required at each step along the way. The desired benefit for growers is a long-term disease management option that requires minimal input to the field and the surrounding environment. More research is needed to assess the economic, environmental, and social benefits for growers.
Overall, this project has allowed a deeper understanding of how passaging can be used experimentally to develop disease suppression in the phyllosphere against a common bacterial pathogen of tomato. Many diseases caused by bacteria do not have an effective application for growers to use in the greenhouse or field to prevent or reduce disease severity. Working in the greenhouse has provided the opportunity to learn hands-on about tomato growth and bacterial disease progression and to envision how our project can enhance tomato production. This project has the potential to develop a management strategy that can support sustainable IPM systems and be applicable in conventional and organic production. This research has aligned with my value of sustainability as an Indigenous scientist and foresee future research following in the lines of discovery around plant diseases and new ethical management practices.
The future of this project is to investigate effective methods for community transfer in greenhouse and field by testing different plant materials (dried, frozen, or slurry) and time of application. Additionally, we will characterize the suppressive phyllosphere communities and identify key functional traits that could be associated with disease suppression using amplicon (bacterial rRNA 16S and fungal ITS1) and shotgun metagenomic sequencing. Assessing the elimination of suppressiveness to show that the disease suppression is due to the presence of the phyllosphere microbial communities will also be investigated.