Final report for GNE19-204
Project Information
Soil microarthropods, a group of fauna dominated by mites and collembolans, mediate multiple microbial-driven soil processes. The effects that soil microarthropods have on crop production are typically explored with a focus on one microbial driven process, such as nutrient cycling or plant pathogen disease incidence, though their behaviors influence these processes simultaneously. The primary objective of this research is to understand how the effects of microarthropods on these interconnected microbial processes impact crop production.
In 2019 and 2020 these relationships were investigated in both soybeans and dry beans under three different cover cropping treatments [1) no cover crop with tillage (control treatment), 2) cereal rye with tillage, and 3) rolled cereal rye with no tillage]. Within this experiment we measured microarthropod communities, multiple microbial metrics (biomass, five enzymes, community DNA analysis), and soil characteristics that impact biological processes (soil carbon and nitrogen, aggregate stability, soil protein). We also measured select plant pathogen metrics for white mold (Sclerotinia sclerotiorum) and root rot [Fusarium spp.], along with crop production metrics (cereal rye biomass, weed suppression, and crop performance).
Multi-model piecewise SEM was used to determine microarthropod effects on bean production and to investigate the role of nitrogen cycling, pathogen dynamics, and weed communities in the crop production outcomes. Our models focused on bean weight, as this is one of the most important metrics to farmers. Soil nutrient status was one of the most important determinants of total bean weight. Microbial biomass nitrogen was an important soil metric, consistently having a negative effect on total bean weight. This effect of the microbial biomass nitrogen could indicate a high relative importance of plant-microbe competition in crop production outcomes.
The two pathogens of interest in these crops, white mold and root rot, were not primary drivers of the crop production outcomes. White mold was not significant in any of the models, and root rot severity only appeared sporadically in the models with mixed effects. Aside from the volunteer rye, weed competition did not play a major role in the models. In the rolled cereal rye plots, volunteer rye was a major problem because of competition. However, it had a positive effect in other treatments, possibly by providing an additional food source for the soil food web that was complementary to the beans.
Collembola, Mesostigmata mites, and Prostigmata mites were the most important microarthropods in the models. The microarthropods had more positive effects when there were more food resources available, as in the rolled cereal rye treatment. Microarthropods had the greatest abundances in the rolled cereal rye treatment, which would result in increased feeding on both soil microbes and the decomposing cereal rye roots. Ultimately this would likely have caused an increase in nutrient cycling and plant available nutrients. We demonstrated that crop management decisions impact if microarthropods have a positive or negative influence on bean production.
Overall, our research indicates that the management of soil biological processes is a central determinant of crop production outcomes. Soil microarthropods specifically showed up as some of the most important drivers of these outcomes. The findings from this research can help inform sustainable crop management systems that rely on soil processes to enhance crop production.
The long-term goal of my work is to improve fertility management on organic and sustainable farms by providing farmers with information and recommendations to enable them to better utilize soil biological processes. To achieve this goal, for this project I focused on the following objectives:
Objective 1. Quantify the effects of the cover crop and tillage practices on soil microarthropod communities, soil microbial activity, soil and plant tissue nitrogen and carbon pools, and crop yield.
Objective 2. Quantify the impact of soil microarthropods on microbial nutrient cycling and crop productivity under different organic crop production practices.
The purpose of my research is to elucidate the functional roles of soil microarthropods in agroecosystems, specifically regarding nutrient cycling and plant pathogen suppression and transmission. This research project was designed to expand our understanding of how soil microarthropods impact nutrient cycling, and how specific crop management practices can indirect impact nutrient cycling through direct impacts on the soil microarthropod community. These results are not only be applicable to organic farmers, but will also be beneficial to any farmer that is seeking to making their cropping systems more environmentally sustainable and resource efficient.
An increased understanding of the important functional roles microarthropods have in agroecosystems will enable farmers to harness these ecosystem services and make informed crop management decisions that will lead to more sustainable agroecosystems that use fertilizers more efficiently. This project increased our understanding of how to manage soil biotic properties, specifically regarding the soil microarthropod communities that mediate multiple soil processes. Increasing the management possibilities surrounding soil microarthropods is a crucial step to allow farmers strengthen the linkage between soil and plant health, a well-known factor in the long-term sustainability of agroecosystems.
Research
The Integrated Cover Cropping Experiment (ICCE) was designed to test the effect of rolled cereal rye on white mold and weed suppression. The soybeans or dry beans are the main plot treatments, and the sub plot treatments are: 1) no cover crop (control treatment), 2) cereal rye with tillage, and 3) cereal rye mulch. The no cover crop treatment did not have cereal rye planted in the fall and used shallow tillage to control weeds as needed. The cereal rye with tillage and cereal rye mulch treatments had cereal rye planted in the fall at a rate of 200 kg seed ha-1. The cereal rye with tillage treatment and the no cover crop treatment were both plowed prior to stem elongation in the cereal rye in the spring. The cereal rye mulch treatment was instead mechanically terminated and flattened using a roller-crimper (no soil tillage). Prior to planting the soybeans and dry beans in the spring, poultry manure and potassium sulfate were applied based on needs as determined by soil testing. The measurements within this experiment included cereal rye biomass, soybean and dry bean establishment, carpogenic germination of Sclerotinia sclerotiorum, Sclerotinia sclerotiorum ascorporic inoculum density, microclimate conditions, white mold disease incidence, weed suppression, and cash crop performance.
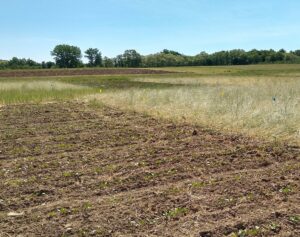
For this research project, I built upon this established field experiment to address my objectives. I completed soil sampling events in June 2019 (pre-SARE grant) and October 2019 to measure soil biological metrics (microarthropod community abundance, microbial biomass, microbial enzymes), physical metrics (soil aggregate stability), and chemical metrics (carbon, nitrogen, soil protein). In 2020 I sampled soil for all of these metrics again in June, July, and September.
To measure the microarthropod community we collected three composite soil samples in each plot, each composed of three soil cores collected from randomized locations in the plots. Each composite soil sample was placed on a Berlese funnel and the microarthropods were extracted into 70% ethanol over a 3‐day period. After extraction, the microarthropods were identified and quantified under a dissecting microscope.
We collected additional soil to send to the Cornell Soil Health Lab for analysis of the soil protein and soil aggregate stability. A hand trowel was used to collect three composite samples in each plot, each composed of a trowel full of soil collected from 3 randomized locations in the plots. Soil was sent to the lab for analysis.
To measure the other soil metrics (microbial biomass, microbial enzymes, soil carbon and nitrogen) one composite soil sample composed of 10 soil cores was collected in each plot. Cores were taken with a soil fertility probe (1.75 cm diameter) to a depth of 15 cm. Microbial biomass was measured using a chloroform (CHCl3) fumigation incubation and extraction. The samples were processed through the total organic carbon/nitrogen analyzer. Microbial enzyme activity was analyzed using the appropriate microplate assays for each of the following enzymes: B-1, 4-N-acetyl-glucosaminidase, Leucine amino peptidase, Phosphatase, and Phenol oxidase.
Rolled cereal rye tissue was collected in June 2019 and again in October 2019 when the beans were harvested. Bean plant tissue was also collected in October 2019 at time of harvest. The plant tissue was dried in oven to remove moisture so the tissue can be ground up and analyzed from carbon and nitrogen content using a combustion elemental analyzer. Yield data was collected for the bean crops at time of harvest. This process was completed again during the 2020 field season.
To tackle the second objective of this project, during the 2020 fields season a stable nitrogen isotope tracer (15N) was added to microplots in the experiment which allows us to track nitrogen as it cycles through the system. Tracking the 15N isotope allowed us to quantify the amount of nitrogen partitioned into the different nitrogen pools measured (bulk soil in microplots, soil microarthropods, microbial biomass, cereal rye tissue, and bean plant tissue). Shortly after the beans were planted in the field, two microplots were set up in each plot. Within each plot, one microplot was covered with cereal rye tissue that was labelled with additional 15N, and the other microplot was covered with non-labeled cereal rye. Over the growing season the cereal rye was decomposed by the soil biota and presumably the nitrogen that was released moved through the soil food web into the bean crop. Near the time of the bean harvest, soil was collected for microarthropod extraction, microbial biomass, and bulk soil nitrogen pools. Any remaining cereal rye tissue in the microplots was collected, and two bean plants from each microplot. The samples collected from the microplots were processed and sent to the Cornell Stable Isotope Lab which has the equipment necessary to determine the 15N content of the samples submitted.
The cover crop treatment had interesting effects on the microarthropod communities. There were more mites in the no cover crop with tillage treatment, primarily driven by Oribatida mite abundances. There were consistently more Collembola in the rolled cereal rye with no tillage treatment compared to the other treatments, and at the end of the growing season there were more Mesostigmata mites which prey on collembolans in this treatment as well. These results were unexpected, as we had anticipated very low abundances in the no cover crop treatment due to there being less organic matter resources available. However, the field this experiment was conducted in had been cover cropped many seasons prior to the experiment set up, therefore the effects of the cover crop in this experiment on the microarthropod communities may have been dampened.
In 2019, soil aggregate stability was affected by an interaction between the cover crop treatment and bean type. Within the dry bean treatments, the no rye-plowed plots had significantly lower aggregate stability that the other cover crop treatments. Within the soybean treatments, the no-till rye plots had significantly higher aggregate stability than the other cover crop treatments. As anticipated, for both the dry beans and soybeans we found that reducing tillage and adding a cover crop increased soil aggregate stability. In 2020, soil aggregate stability was impacted by the cover crop treatment. Both the no rye-plow and rye-no till plots had significantly greater aggregate stability that the no rye-plow plots.
It is interesting is how quickly soil aggregate stability changed in this experiment. Prior to this experiment each of the fields for each growing season had been treated the same for many years, so it is presumed that the soil aggregate stability would have been similar across the entire field. Since the soil aggregate stability changed in the experiment plots significantly in one year in two separate fields, this suggests that even incorporating just one season of reduced tillage and cover cropping into a farmer’s rotation can help to improve soil aggregate stability.
The cover crop treatments did not have much of an effect on microbial biomass, but they did have mixed effects on the production of microbial enzymes. The microbial community produced extracellular enzymes based on the resources in the soil environment, so these results were as expected.
For objective 2, preliminary nitrogen and carbon isotope natural abundance analysis results revealed interesting changes in the nitrogen and carbon dynamics in these systems. The microplot cereal rye N isotopes were affected by the tillage treatment, with the no-rye plowed plots having greater nitrogen isotope concentration than the plowed rye and rye no-till plots. The beans affected the percent of carbon in the cereal rye, with the soybeans having a greater percentage of carbon than the dry beans.
In the microplot soil there were no effects on the percent of nitrogen or carbon, but significant bean and tillage interaction effect on N isotopes, with the dry bean having greater N isotope concentrations than the soybeans in the no rye plow treatments. There was also a significant tillage effect on carbon isotopes, with the rye no-till plots having greater C isotope concentrations than the rye plow plots.
The microarthropods had greater percent nitrogen content in the soybeans than the dry beans, and lower N isotope concentration in the soybeans compared to the dry beans. The tillage treatments also affected the microarthropod N isotope concentrations, with the no rye plowed plots having greater concentrations than the rye plowed plots. The microarthropods had greater percent carbon content in the soybeans than the dry beans, and lower C isotope concentrations in the soybeans compared to the dry beans. The tillage treatments also affected the microarthropod C isotope concentrations, with the rye no-till plots having greater concentrations than the no rye and rye plowed plots.
The bean shoots percent nitrogen content was affected by the bean and tillage treatment interactions, with the soybeans being impacted by the tillage treatment. The no rye plowed plots had greater nitrogen content that the rye plowed and rye no-till plots. The tillage treatment affected the bean shoot N isotope concentrations, with the no rye plowed plots having greater concentrations the rye plowed and rye no-till plots, and the rye plowed plots having greater concentrations than the rye no-till plots. The bean shoot percent carbon concentration was greater in the soybeans compared to the dry beans. There was a treatment interaction effect on the C isotope concentrations, with in the soybean treatment the no rye plowed plots had greater concentrations than the rye plowed plots. These nuanced results illustrate the complex changes in nitrogen and carbon dynamics due to the bean and tillage treatments.
The agroecosystem modeling revealed that soil nutrient status was one of the most important determinants of total bean weight. Microbial biomass nitrogen was an important soil metric, consistently having a negative effect on total bean weight. This effect of the microbial biomass nitrogen could indicate a high relative importance of plant-microbe competition in crop production outcomes.
The two pathogens of interest in these crops, white mold and root rot, were not primary drivers of the crop production outcomes. White mold was not significant in any of the models, and root rot severity only appeared sporadically in the models with mixed effects. Aside from the volunteer rye, weed competition did not play a major role in the models. In the rolled cereal rye plots, volunteer rye was a major problem because of competition. However, it had a positive effect in other treatments, possibly by providing an additional food source for the soil food web that was complementary to the beans.
Collembola, Mesostigmata mites, and Prostigmata mites were the most important microarthropods in the models. The microarthropods had more positive effects when there were more food resources available, as in the rolled cereal rye treatment. Microarthropods had the greatest abundances in the rolled cereal rye treatment, which would result in increased feeding on both soil microbes and the decomposing cereal rye roots. Ultimately this would likely have caused an increase in nutrient cycling and plant available nutrients. We demonstrated that crop management decisions impact if microarthropods have a positive or negative influence on bean production.
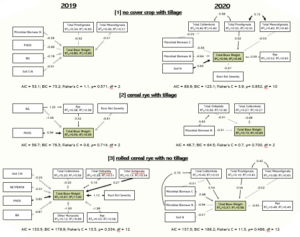
Overall, our research indicates that the management of soil biological processes is a central determinant of crop production outcomes. Farmers should prioritize enhancing the biologically driven nutrient cycling in their fields, as this management focus may have the largest impact on their yields relative to pathogen and weed management.
Soil microarthropods specifically showed up as some of the most important drivers of crop production outcomes. We observed that the microarthropods have more positive effects when there are more food resources available, as in the rolled cereal rye treatment. Microarthropods also had greater abundances in this treatment, which likely resulted in increased feeding on both soil microbes and the decomposing cereal rye cover crop roots that had not been disturbed by tillage. Therefore, leading to increased nutrient cycling and more plant available nutrients.
The findings from this research can help inform sustainable crop management systems that rely on soil processes to enhance crop production. We recommend that farmers prioritize feeding their soil biota and providing hospitable habitats by reducing tillage and increasing organic matter availability through cover cropping.
Education & Outreach Activities and Participation Summary
Participation Summary:
Education and outreach efforts have been facilitated through an Extension and Outreach Activity (EOA) coordinated by Cornell's entomology department. During the fall of 2020 I worked with three farmers in the Finger Lakes region that farm varying cropping systems. Each farmer selected multiple fields of interest, then I collected microarthropod samples from each field which will allow us to compare how different crop management practices influence microarthropod communities. Samples from these farms were processed and the results were incorporated into outreach materials. I distributed individualized reports detailing these results to each farmer who participated with additional insights and recommendations for their farms.
I completed three fact sheets that communicate (1) who mesofauna are and why we care about them, (2) where they are and how we know, and (3) what affects mesofauna and how can they be managed (https://cpb-us-e1.wpmucdn.com/blogs.cornell.edu/dist/9/4123/files/2021/07/Jernigan_EOA-Fact-Sheets_Merged.pdf). I also created a video (in place of on-farm demonstrations due to pandemic) that describes these concepts in more depth (https://www.youtube.com/watch?v=AHVGFDpRXhQ&t=205s).
Project Outcomes
This project has the potential to contribute to future sustainability by informing farmers how to best steward their soil to promote a well-functioning agroecosystem that is less reliant on external inputs. Improved soil functioning has the potential to reduce external inputs that can have environmental consequences (fertilizers, pesticides) and can cause economic strain to farm operations.
During the course of this project I gained many new skills related to the different soil ecology methodologies utilized for this project. These methodologies can help inform the impacts of sustainable agricultural practices. Through conversations with farmers I became more aware of the trade-off that farmers must consider when making sustainable crop management decisions. In the future I hope to conduct research that will help farmers make scientifically-back decisions around these tradeoffs.