Final report for GNE20-228
Project Information
The use of pesticides has unintended effects on pollinators due to pesticides drifting to non-target areas. This drift creates contaminated areas and resources that are used by bees and other pollinators. Wildflowers growing near agricultural areas can be exposed to pesticide drift and become conduits of pesticides if they absorb pesticides and express them in their floral rewards or if the pesticides drift onto blooming flowers. To assess if particular wildflower species serve as conduits of pesticide exposure, I combined field observations with a pesticide expression study and used these data to build a risk model for foraging bees. I assessed floral availability and bee visitation to wildflowers growing in unmown areas adjacent to agricultural fields at the Horticultural Research and Education Center (Hort Farm) in South Burlington, Vermont. For two of the species that were most commonly visited by bees, red clover (Trifolium pratense) and English plantain (Plantago lanceolata), I quantified the extent to which they expressed soil-derived pesticides by growing the species from seed in a greenhouse and exposing the flowers to 0.193 mg of Imidacloprid and/or Difenoconazole per 200 ml of water each week. At the conclusion of the experiment, pesticide concentrations in the anthers were quantified for each floral species.
Results from the field observations of floral resources in non-agricultural areas showed that bees used the unmown areas throughout the farm and had overlapping, but not identical, preferences. Wild bees, all bees except honeybees, preferred wildflowers such as common yellow wood sorrel (Oxalis stricta) and red clover (Trifolium pratense), while honeybees preferred English plantain (Plantago lanceolata) and great mullein (Verbascum thapsus). When red clover and English plantain were exposed to Imidacloprid, 0.46 and 0.66 of the pesticide concentration from the soil was expressed in the anthers, respectively. When red clover and English plantain were exposed to Difenoconazole, expression rates were 0.10 and 0.64, respectively. Pesticide expression rates were used in creating a predictive model that estimated habitat risk. The model predicted that bees could be exposed to a maximum percentage of 15% of their lethal dose in a single day when foraging on English plantain or 11% if bees foraged on red clover that expressed Imidacloprid. These results assumed that bees were exclusively foraging in the contaminated landscapes. However, bees foraging in contaminated areas for shorter lengths of time can still be exposed to concerning levels of pesticide. The model showed that bees that foraged in the contaminated landscape for just 30 percent of the time, or in areas with as little as 25 parts per billion of one pesticide, were exposed to at least 3% of their lethal dose in one day. These risk percentages were only relevant for Imidacloprid only. Because Difenoconazole has a large LD50, the risk posed by this pesticide was miniscule. Our study shows wildflowers in agriculture can act as conduits of pesticide and expose bees to pesticides through their floral rewards. The impact this could have on agriculture is dependent on the practices of the farm. Farms promoting wildflower proliferation for bees need to be aware of the additional risk these wildflowers could pose if actively utilizing pesticides.
The main goal of my research was to investigate the role pollinator friendly flowers play in exposing pollinators to pesticides. Using greenhouse experiments and observational field surveys, this research addresses the following objectives:
Objective 1. Determine if flowers vary in their expression of pesticides in floral resources.
Objective 2. Identify the risk flowers grown in contaminated soil pose to pollinators.
This work benefits management practices of pollinator conservation (farmers, academics, citizens, etc.) by providing information on the role wildflower play in exposing pollinators to pesticides.
Bees pollinate 80-90% of flowering plants, including 75% of agricultural crops, and contribute 235 - 577 billion dollars to the economy each year 10,111 There are an estimated 25,000 bee species worldwide, with many of the species in decline 12. Out of concern for our food supply and ecosystem health, efforts have increased to aid pollinators. Despite the increasing research in this area, nothing has been definitively identified as the cause of the decline bee populations. However the increasing use of pesticides has been strongly implicated as the cause of pollinator declines6.
Pesticides have been implicated in numerous studies as harmful to pollinators4,5,16–19. They are acutely toxic to pollinators, reduce brood survival and foraging success and lead to Colony Collapse disorder 4,5. Although most pesticide applications occur in agricultural habitats, non-agricultural areas and flowers are increasingly recognized as a significant additional source of exposure19–21. As little as 1-10 % of aerosol pesticides, and 1.6-20 % of systemic pesticides, reach target plants directly, with the rest ending up in the soil, water and air 5,22. These pesticides can remain in soils for years after the initial application9,19,23–25, where they can be absorbed by non-target plants and expressed in plant tissue, nectar and pollen3,26–28.
These non-target plants can act as a potential route of exposure of pollinators to pesticides 4,19,30. Despite this knowledge, a common conservation tactic for pollinators is restoring and conserving land and creating pollinator gardens to provide more floral resources to bees1,32. This habitat restoration strategy has received less scientific attention despite the increasing number of guides for pollinator friendly plantings 33. In order to maximize the conservation potential of planting pollinator friendly plants, the beneficial or detrimental parameters of these tactics must be identified 33. Identifying which flowers represent the best choice to aid pollinators in a contaminated landscape is necessary because they will minimize further exposure to pesticides. The risk that non-agricultural soil contamination poses to bees depends critically on the extent to which pesticides are expressed by plants in pollen and nectar, yet this is poorly understood outside of agricultural crops 7,25. We know the lethal and sub-lethal effects that pesticides have on pollinators5. However, there is a gap of knowledge regarding the environmental fate of pesticides and the extent to which flowers pose as risks to pollinators4,8,19,24,34.
The purpose my PhD work this project here was to examine the role of flowering plants in non-agricultural areas as potential routes of exposure of pollinators to pesticides and quantify the variation among species in absorption of pesticides from soil and expression of pesticides in nectar and pollen.
Research
Objective 1: Determine if flowers vary in their expression of pesticides in floral resources.
Question 1 (Q1): Do different species of flowering plants express different levels of pesticide?
Study System: The floral species used in this experiment were: red Clover (Trifolium pretense) and English plantain (Plantago lanceolata). These species were commonly observed throughout the summer of 2019 and were preferentially visited by pollinators during an observational floral preference study. These species provided floral resources to a variety of pollinators throughout the summer and fall season, including honeybees and a variety of wild bee species.
To answer Q1: Greenhouse experiments with Trifolium pratense and Plantago lanceolata began in September 2021 to determine expression rates of pesticides and to determine if pesticide type affects expression rates. To determine the rate at which preferred wildflowers express pesticides from soil contamination, I conducted a 2 x 4 factorial design greenhouse experiment and quantified pesticide expression in Plantago lanceolata (English plantain) and Trifolium pratense (red clover). Each treatment consisted of forty replicate plants for Trifolium pratense and thirty-four or thirty-five replicate plants for Plantago lanceolata. Imidacloprid, a systemic neonicotinoid insecticide, was used in the experiment along with Difenoconazole, a systemic fungicide. The experiment consisted of one control group and three treatment groups that were exposed to either Imidacloprid, Difenoconazole, or Imidacloprid and Difenoconazole.
Plant species and culture. Seeds of red clover and English plantain were started in four-inch pots on September 7th, 2021, and September 12th, 2021, respectively in the main campus greenhouse of the University of Vermont. Pots were filled with Pro-mix potting mix comprised of Canadian sphagnum peat moss, perlite, vermiculite, dolomitic and calcitic limestone and mycorrhizae. Plants were exposed to 16 hours of daylight and 8 hours of darkness and were maintained at 24℃ during the day and 20℃ at night. Plastic barriers were placed between the plantain treatments and between plantain and red clover to eliminate cross contamination of pollen. To initiate germination, the pots were misted with water immediately after being sown and watered every other day using a bottom-up method. The process occurred by filling plant trays with water for approximately 30 minutes. This time was to allow water to be drawn up directly to the roots of the plants. After 30 minutes, the trays were emptied. Pots were provided liquid fertilizer (15-16-17) at 200 ppm nitrogen on a bi-weekly basis via bottom-up watering as well. Plants began blooming on October 29, 2021, and the experiment was terminated before all plants stopped blooming on January 9th, 2022.
Plant treatment protocol – soil drench. The treatment protocol began October 13th, 2021 and ceased January 4th, 2022. The treatment does was approximately 0.000965 mg/ml of each the appropriate pesticide. Each pot received ~200 ml of the pesticide solution poured directly onto the soil of each plant. This dose was a calculated average of field rates from the literature and scaled down to the size of the potted plants (Robin and Stork 2003, Dively and Kamel 2012, Krischik 2015, Cowles and Eitzer 2017). After 24 hours, any solution that was not absorbed by the plants was collected as waste.
Anther collection. To determine the rate of pesticide expression in floral rewards, I collected pollen and anthers from each species over the course of the blooming season. To maximize the amount of sample material, while minimizing the inclusion of tissue not relevant to bee exposure, the anthers were included with the pollen samples. Red clover inflorescences were temporarily stored in a refrigerator at ~ 5℃ until the anthers could be removed. To collect the anthers for red clover, individual florets of a red clover inflorescence were removed and placed on weigh paper. Using sterile forceps, each floret was gently opened, and the anther tissue was removed. All anthers from a single inflorescence were collected into a 1ml cryo tube, labeled with the unique ID of the inflorescence, and stored at -20℃. To collect the anthers for English plantain, which is a wind pollinated species, care was taken to ensure that the floral head did not shed its pollen outside of the collection tube. To ensure as much pollen as possible was collected, the inflorescence was slowly inserted two-thirds of the way into a labeled 50 ml falcon tube before the stalk was cut, quickly sealed and stored in a -10℃ chest freezer until the flowers could be processed and the inflorescence head was discarded. Neither species produced a sufficient amount of anther tissue and pollen on a single collection date for chemical analyses. Therefore, multiple samples from each species, and respective treatment groups, were taken from various time periods and pooled to create 100 mg samples for analysis. On average, 12 samples of red clover were needed to create 1 pooled sample with a minimum of 100 mg. For English plantain, an average of 15 samples were needed to create 1 pooled sample.
Soil collection. To measure the level of pesticide contamination pesticide in the soil relative to the concentration of pesticides in the floral reward samples, three replicate soil samples for each species and treatment were collected on December 2nd, December 30th, and January 13th for pesticide analysis. Core samples from multiple pots in a treatment were collected and homogenized into one sample before being stored in 50ml tubes. After sampling, the tubes were labeled and store in a chest freezer at -10℃.
Extraction of pesticide residues. Pesticide extraction for all soil and floral reward samples occurred at the Cornell Chemical Ecology facility. The Quick, Easy, Cheap, Effective, Rugged and Safe (QuEChERS) pesticide extraction protocol was used to clean and purify samples before quantifying pesticide residues using liquid chromatography and mass spectrometry (Won et al. 2010). Liquid chromatography uses pressure to separate the compounds in the sample by identity which can be determined from the weight. Mass spectrometry was used to identify the detected compounds, based on pesticide specific presets, and give the concentration, in parts per billion (ppb), of pesticide in the sample.
Data analysis: pesticide residues. All analyses were performed in R (version 4.0.2) using built in anova, linear regression and Kruskal-Wallis functions. Pesticide expression data were log transformed to fit a more normal distribution. To confirm an ANOVA was appropriate, Kruskal-Wallis tests were also conducted. The Kruskal-Wallis tests returned equivalent results as the ANOVA; thus, ANOVA analyses were kept as the main analysis method. To determine if the applied pesticide persisted in the soil, I used an ANOVA with species, treatment, and time as predictor variables and pesticide concentration as a response variable. When significant effects were found, I used Tukey HSD tests to test pairwise differences between treatment groups and/or species. To quantify the relationship between pesticide residues in the soil and pesticide residues in pollen/anther samples, I conducted a regression analysis of pesticide concentrations in the pollen/anthers on pesticide concentrations in the soil for Imidacloprid and Difenoconazole.
Note: This experimental design was used to show differences in species expression and differences in expression of different pesticide types. However, one of the original objectives (objective 2), to test for differences in expression based on pesticide dosage was unable to be tested in this experiment.
Objective 2: Identify the risk flowers grown in contaminated soil pose to pollinators.
To evaluate the risk that red clover and English plantain in unmown areas pose to pollinators, I constructed a model to predict % of LD50 bees would be exposed to, given a range of soil contamination levels and foraging activities in these areas. The input parameters were based on values from the literature and previous experimental data. The parameters used in the model included soil contamination range for non-target areas, pesticide expression in floral species, and percentage of foraging time a bee would spend in the habitat. The mathematical equation used in the model was based on the pollen hazard quotient equation originally described by Stoner et al., (2019) and Stoner and Eitzer (2013):
Risk = pesticide residue [ppb]/LD50 [ug]
The final equation, quantified for an individual bee, was used in the model:
Est.daily LD50 = ((pesticide rate x pesticide residue[ng/g] ) x (avg. consumption of pollen/day [g] * foraging time)) / ((oral LD50 (ng)) ) * 100
This model multiplies the soil pesticide rate by the expression rate to determine the amount of pesticide from the soil that are translocated into the pollen of wildflowers growing in the habitat. Lethal dose values for each respective pesticide were obtained from the literature. The LD50 for Imidacloprid was 0.0039 ug and the LD50 for Difenoconazole was 177 ug (Stoner and Eitzer 2013). Pesticide rate and foraging time were represented by proportions. Original units for pesticide residues were in ng/g. The units for lethal dose were ug and the units for pollen consumption were in mg. All units for each variable were converted to ng to calculate the amount of pesticides. Pollen consumption, proportion of foraging time, pesticide rate of expression and pesticide residue are multiplied in the numerator to determine amount of pesticides in pollen. The lethal dose is divided into the numerator to determine the percentage of the lethal dose the bee would consume. This modification of the equation allows for the risk of wildflowers to be factored into the overall risk of the habitat. Soil contamination levels varied from 0 to 100 ppb, similar to levels seen in other non-target areas of agriculture (Greatti et al. 2006), while foraging time varied from 0 to 1. These variables were varied to determine their effects on risk. LD50 and average milligrams of pollen per day were set variables that did not change. The value used for daily consumption of pollen was set at 9.5 mg, based on the average amount of pollen a nurse honeybee consumes in a day (Crailsham et al. 1992, Rortais et al. 2005).
Pesticide Concentrations in Anther/Pollen
Imidacloprid residues detected in the anthers of red clover and English plantain ranged from 19 ppb to 1027 ppb. Pesticide concentration for Imidacloprid varied significantly between treatment groups but not between species (F1,41 = 0.62; p = 0.436; Figure 1). Imidacloprid residues were significantly greater than residues in control and Difenoconazole treatment (Tukey HSD; p < 0.01). Imidacloprid applications for English plantain led to residues 32X times greater than residues in the control and 19X times greater for red clover. When English plantain was treated with both Imidacloprid and Difenoconazole, detected residues were 39X greater than residues from the control group and red clover had residues 28X greater than the control group.
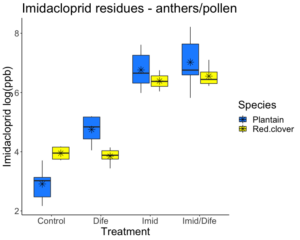
Difenoconazole residues in the anthers of red clover and English plantain ranged from 8 ppb to 6606 ppb. Pesticide concentration for Difenoconazole in the anther samples varied significantly between treatment groups (F3,41 = 8.644; p < 0.01; Figure 2) and species (F1,41 = 68.315; p < 0.01). Plants treated with Difenoconazole had residues 3X greater for red clover, but English plantain did not experience higher residues in this treatment group. Anthers from English plantain treated with both Imidacloprid and Difenoconazole had residues 85X greater than those in the control while anthers from red clover had residues 14X fold greater than plants in the control group.
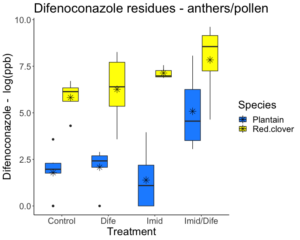
Pesticide expression rate
The relationship between pesticide concentration in the soil and anthers was non-linear for both species (Figure 3). The fitted regression model for Imidacloprid residues in the soils and residues in English plantain anthers was 2.4 + 0.66X and 2.8 + 0.46X for red clover (Figure 3). The overall regression was statistically significant (F1,44 = 178; p < 0.01). Between species, plantain had a higher rate of expression than red clover. The slope of the regression for plantain was 0.66, while the slope for red clover was 0.46. The fitted regression model for Difenoconazole residues in the soils and residues in English plantain anthers was -1.3 + 0.64X and 6 + 0.1X (Figure 4) for red clover. The overall regression was statistically significant (F1,45 = 126.7; p < 0.01). Plantain expression of Difenoconazole occurred at a rate of ~0.64 while expression of residues for red clover occurred at a rate of 0.1.
The results show floral species, that commonly grow in unmown areas of conventional agriculture, readily absorb and express pesticides when growing in soils contaminated with pesticides. When the expression of pesticides from the floral species is considered along with other influencing variables including bee foraging time, daily consumption of pollen and lethality of specific pesticides, then the risky floral species can expose bees to concerning levels of pesticides.
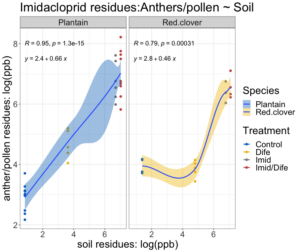
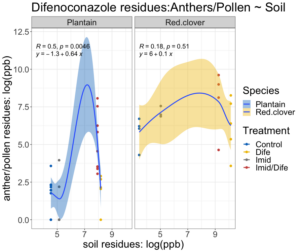
Risk
Results for Objective 2:
Because the rate of pesticide expression differed between species, two models were created to reflect the risk of red clover and English plantain in the contaminated landscapes. Large concentrations of Difenoconazole were expressed in the anthers of both English plantain and red clover, however, due to the higher LD50 of Difenoconazole, the risk posed to bees was not significant and therefore, not visualized. The models for Imidacloprid showed that as residues in the soil increase and/or percent foraging time increase, bees were exposed to a greater percentage of their LD50. English plantain growing in soils that contain pesticide residues ranging from 0 to 100 ppb, can expose bees to up to 15% of the lethal dose for Imidacloprid in one day (Figure 5). Red clover growing in soils that contain pesticide residues ranging from 0 to 100 ppb, can expose bees to up to 11% of the lethal dose for Imidacloprid in one day (Figure 6).
Based on the predictive model, the risk to bees from contaminated floral resources is dependent on the floral species and the type of pesticide. We found that pesticide expression differed between species and between pesticide types. Between species, English plantain served as a better conduit of pesticides than red clover for both pesticide types. When comparing pesticides, Imidacloprid was more readily expressed than Difenoconazole and had greater risk. Thus, indicating that risk posed by the floral species is not straightforward and will depend on context.
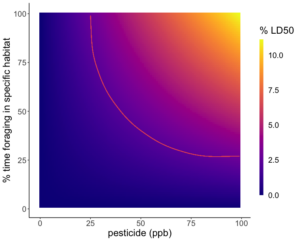
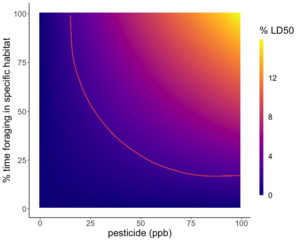
This study has provided evidence that wildflowers growing within agricultural areas, planted or allowed to proliferate naturally, serve as conduits of pesticide exposure to bees and other pollinators. We found that flowers absorbed and expressed residues from the soil and bees foraging on these risky floral species may be exposed to concerning levels of pesticides. This study contributes valuable information regarding the role of wildflower species in exposing bees to pesticides, but also highlights the need for further study. In the future, investigating more floral species is crucial given the varying responses seen within this study. Additionally, investigating other pesticides, and combinations of pesticides, would provide more results on the response of plants to pesticides. We found that expression was different when pesticides were in the presence of another pesticide and knowing if this is a consistent pattern for multiple pesticides would be vital given that multiple pesticide residues are often found in the environment. It is highly likely that bees in conventional agriculture are exposed to multiple routes of pesticide exposure that contribute to greater cumulative exposure over time. Pollinator success is dependent upon identifying these routes of exposure and mitigating their responses to ensure bee safety.
Education & Outreach Activities and Participation Summary
Participation Summary:
Results from this project will be shared with the public and academic sector in various ways. I am creating an information poster describing my results and highlighting the risk of wildflowers when growing in contaminated areas. This leaflet will be distributed in the Xerces Society Newsletter and within the Vermont Beekeepers Association.
Throughout the course of the greenhouse experiment, I worked with various undergraduate students and trained them on experimental methods, plant cultivation, pesticide awareness, safety, and risk. I hosted a Teen Science Cafe through the UVM 4H program. During this time, I gave a 45-minute presentation to students (K - 12) and their families about the potential risk wildflowers may pose to pollinators.
Additionally, results from this study were presented at the International Union for the Study of Social Insects. The manuscript created from this symposium was recently accepted for publication in Insectes Sociaux.
Project Outcomes
The outcomes from this experiment showed:
- Wildflower species can be conduits of pesticide exposure to bees when growing in contaminated landscapes because the floral species absorb and express pesticides.
- Pesticide type affected expression between the wildflower species and the presence of multiple pesticides increased expression.
- Pesticide exposure affected other plant characteristics which can affect the risk to bees.
- Contaminated floral resources represent an additional route of pollinator exposure to pesticides that warrants more investigation.
My view of sustainable agriculture has increased when reviewing the work that others have done through SARE. Sustainable agriculture is more than just one decision but an ongoing process and responsibility. We have a duty to protect and serve our communities and ecosystems to preserve them or the next generation.
My future career path will focus on building the bridge between academia and the public. I envision a career as an outreach/extension coordinator that targets under-represented youth and communities. My goal is to increase STEM awareness in those communities by facilitating science based experiences that the public can attend on a voluntary basis to learn more about specific fields of STEM. I hope to be able to begin some of this work by collaborating with he 4H department at UVM.