Progress report for GNE22-296
Project Information
Microbial carbon use efficiency (CUE) is a critical property of the soil ecosystem that affects both carbon(C) storage and nitrogen (N) mineralization. Microbial CUE is the proportion of C in decomposing organic material that remains assimilated in microbial biomass rather than being respired as carbon dioxide. When microbial CUE is high, plant residue inputs are more effectively stabilized into soil organic matter. But increasing CUE reduces N-mineralization because microbial retention of C requires retention of N. This research will evaluate how soil texture, cover crop residue type, and N-fertilizer additions affect CUE in agricultural soils. Experiments will be conducted on two farmer fields and a research station in Pennsylvania for two years. Microbial CUE will be measured through oxygen isotope labeling, enzyme assays, and cover crop decomposition in litterbags. Microbial CUE will be related to soil properties, microbial community structure, and farm management factors to identify the relevant controlling factors. Finally, measured, and predicted values of CUE will be used to improve a new prototype decision support tool that predicts N-mineralization based on microbial processes and adjusts N-fertilizer recommendations accordingly. Results from the project will be disseminated through a co-learning workshop, a fact-sheet, farmer conferences, and an academic meeting. The improved understanding of CUE gained from this research will help farmers increase soil carbon storage as well as more precisely adjust N-fertilizer inputs based on predictions of N-mineralization from soil organic matter and crop residues, leading to greater farm profitability and fewer N losses to the environment from N over-fertilization.
- Evaluate and understand the effect of cover crop residues and the quantity and type of supplemental N fertilizer inputs on microbial carbon use efficiency.
- Assess whether microbial communities (e.g., fungal: bacteria ratios, ammonia-oxidizing bacteria) respond to different availability of carbon and nitrogen resources and determine if this influences carbon use efficiency and enzyme activity
- Determine if factors that control microbial CUE could be used to improve a nitrogen fertilizer recommendation tool that relies on an estimate of CUE to calculate N mineralization of cover crop residues and soil organic matter.
- Compare corn yield response to supplemental N fertilizer additions to the recommended N application rate from the decision support tool and evaluate how adjusting CUE in the decision support tool with field measured CUE improves the tool’s prediction
Microbial CUE is the proportion of decomposed C used by microorganisms (as a food source) to increase their microbial biomass rather than being lost through respiration1,2. Higher values of microbial CUE allow plant residue inputs to be more effectively stabilized into the microbial biomass and, ultimately, soil organic matter. But the efficient utilization of C to grow microbial biomass demands greater microbial N assimilation because microbial biomass is generally made up of one part of N for every ten parts of C. Hence, a higher CUE means less N from decomposing residues will mineralize into ammonium and be available for plants to use3. This makes microbial CUE a fundamental factor regulating N-mineralization rates. Substantial literature shows that CUE varies according to the type of decomposing substrate (e.g., cover crop C: N ratio), environmental factors, soil inorganic N availability, and microbial community composition. However, most of this knowledge comes from studies in forests or laboratories, leaving a knowledge gap in how microbial CUE behaves in agricultural soils4-7. The latter drives the necessity to evaluate, test, and validate the current understanding of CUE and N-mineralization relationships on farms to support N management decisions.
This project aims to create a robust framework gathering the relationships between microbial CUE, N-supply, and the microbial community as affected by soil properties and agricultural management. This framework will be key to improving the N-mineralization model used in a new N decision-support tool that corn growers can use to obtain recommendations for nitrogen applications based on their cover cropping practices, soil texture, and soil organic matter. N decision-support tool for this tool are currently based on soil texture as suggested by corn yield responses to soil organic matter content and cover crop biomass N and C: N ratio that were observed across a wide variety of sites8. Importantly, microbial CUE was never directly measured at these sites, and there are ranges of soil texture where the tool currently predicts a negative CUE, which is not biologically possible. Furthermore, other factors that are known to affect microbial CUE, such as the C: N ratio of decomposing plant residues, microbial community composition (e.g., bacterial: fungal ratio), and level of external N inputs, are currently not considered by the N decision support tool. A more robust understanding of model microbial CUE in agricultural systems will improve the existing decision support tool and better allow farmers to implement sustainable practices, calculating the contribution of nitrogen from cover crops and soil organic matter. Thus, having the capability to accurately predict the correct amount of supplemental nitrogen needed for corn will reduce under- and over-fertilization, thus reducing economic losses and N leaching while maintaining high crop yields. Likewise, this is not only key to helping farmers make ongoing decisions for current years; inserting this framework into the N decision-support tool will enable farmers to evaluate different scenarios according to their site-specific conditions to integrate cover crops and build up in the long-term soil organic matter.
Cooperators
Research
Study site
The study was conducted in three different locations in Pennsylvania, (1) Turbotville/Muncy in Northumberland County, (2) New Holland in Lancaster County, and (3) Pennsylvania Furnace in Centre County. The focal crop for this study is corn because it has a high demand for nitrogen (N) inputs, and it is the most widely planted grain in the Northeastern U.S.. Results from this study will support a larger research project where a corn N decision-support tool is being refined6. Three experimental fields will be established: two of them on-farm sites (Carl Schmidt's farm in Turbotville/Muncy and Wade Esbenshade’s farm in New Holland), and the third site used different cover crop plots embedded within a long-term experiment at Penn State’s Rock Springs Research Farm in Pennsylvania Furnace.
Field experiments took place for two years. An initial year was already completed in 2021 at Muncy, New Holland, and Rock Springs, where soil samples to be used in this study were collected and preserved, and initial laboratory extractions of soils were completed, resulting in frozen extracts that remain to be analyzed. The field experiments were repeated in 2022 with on-farm sites rotating to different fields. The variation across experimental sites will allow me to assess CUE and N-mineralization relationships in different soil textures, soil organic matter levels, and cover cropping practices. The soil in the experimental field located on Schmidt’s farm (41°05' N 76°45' W) and Esbenshade’s soil (40°05′ N, 76°04′ W) are classified as Hagerstown soil series (Fine, mixed, semiactive, mesic Typic Hapludalfs), and Rock Spring’s research field (40°43′ N, 77°55′ W) contains Murrill and Buchanan soils (Fine-loamy, mixed, semiactive, mesic Typic Hapludults, and Fine-loamy, mixed, semiactive, mesic Aquic Fragiudults respectively). Each experimental field had a different cover crop management: a grass and legume mixture at Schmidt’s farm, a mix of 5 species (radish, triticale, oat, crimson clover, and peas) at Esbenshade’s farm, and for the experimental field at Rock Spring’s farm, three cover crop treatments are included: triticale monoculture, crimson clover monoculture, and fallow.
Experimental design
The experimental design was a Randomized Complete Block Design with four replicate blocks. Each block was divided into six experimental plots (15 m x 5 m each with 2.5-m buffer zones between adjacent plots) and assigned one of the six levels of N fertilization treatments. The six N-levels were established as 0, 30, 60, 120, and 240 lb N/ac. Although experimental fields were divided into six N-levels for the N decision-support tool assessment, to complete the objectives of this proposal, were considered only 0, 120, and 240 lb N/ac treatments. For yield response analysis, the variety among N additions between sites was evaluated through a regression model. The fertilization levels will be comparable to previous N addition experiments used in the development of the decision support tool. Because the on-farm trials are under organic production, N additions were performed using Chilean Nitrate fertilizer, a naturally-occurring mineral N source allowed in organic production. Because Chilean nitrate is a mineral source of nitrogen (sodium nitrate), results from the study can also be extrapolated to conventional farmers that use mineral fertilizers such as urea and urea-ammonium-nitrate solution. Response to nutrient management with fertilizer additions will be compared to on-farm nutrient management practices (with four external experimental plots at each side of the experimental fields), according to the manure applications performed by farmers.
Soil sampling
To accomplish the soil analysis related to the objectives of this study, soil samples were collected on three sampling dates per year: baseline (before cover crop termination), establishment (while corn is in the early growth stages, ~V6 stage), and late (near corn harvest). Taking into consideration the microbial characterization, soil sampling was conducted following an aseptic protocol, rinsing tools with isopropyl alcohol (70%) and letting them dry before making another sampling. From each plot, 15 soil sub-samples (cores of 0-20 cm depth) were collected from random locations and combined into a single composited soil sample. Soil samples were placed in plastic bags and stored in a compartment with ice packs to transport to Soil Fertility Laboratory at Penn State. Each composite sample was sieved through a 2-mm diameter mesh sieve to remove coarse material and roots and divided into three parts: (1) the sub-sample (air dried) used for a standard soil fertility test, texture, SOM, total C and total N, (2) a sub-sample that was storage at –20 °C until Next Generation Sequencing, extractable inorganic N and enzyme analysis, and (3) a subsample stored at 4 °C until microbial carbon use efficiency analysis trough 18O-water tracing and cumulative respiration.
Before (less than one week ahead of) cover crop termination in spring, cover crop biomass and its N concentration (Objective 1) were measured by species in 3 small quadrants (0.25 m2) per plot. For each sampling date, soil extractable inorganic N (NH4+—N and NO3—N) was measured with 2M KCl solution. The microbial biomass carbon quantification will be measured using the chloroform fumigation extraction method with 0.5 M potassium sulfate11. Briefly, fumigation was performed for a period of 48 hours in vacuum desiccators with ethanol-free chloroform. Extractions were stored for further analysis. Afterward, an estimation of total organic carbon (TOC) will be conducted to calculate the differences between fumigated and non-fumigated soil samples. The fumigation provides a means to calculate the microbial biomass because the carbon in microbial biomass is released by the lysis of the microbial cells exposed to chloroform. A trial for a new approach to measuring TOC through an elemental analysis was conducted with good performance. All soil samples for microbial carbon use efficiency measurements (Objective 1) will be incubated for 5 days at 15 °C before analysis. The 18O-water tracing method uses 18O-labeled water into microbial DNA to calculate total growth and then microbial CUE12,13. Briefly, 3 g of soil will be labeled with 18O-water to reach 20.0 at% of 18O in the final soil water content (field capacity). After every five samples, an empty vial will be sealed as a control sample of lab air. Samples will be incubated at 15 °C for up to 48 h. After the incubation, a 1 mL gas sample was used to determine CO2 concentration with a LICOR-7100 infrared gas analyzer. Soils were frozen at –20 °C until DNA extraction. DNA will be extracted with a kit according to the manufacturer’s protocol and the extract (n=278) will be dried13 and sent to the Cornell University Stable Isotope Laboratory for δ18O quantification. Cumulative respiration described above will be used for the calculation of CUE with 18O-water according to Spohn et al. (2016b)14.
Update 2024: DNA extraction and DNA yield for the 18O water tracing method in the first year were conducted. The DOC trial was carried out in a more extended study to determine if the Unicube method could be used to access the values needed for microbial biomass carbon.
Next steps: Samples will be sent to Cornell lab in late March 2024 for isotope analysis.
The stoichiometric modeling of CUE uses indicator exoenzymes (Objective 1 and 2) to evaluate resource uptake and allocation related to C and N resources available and microbial community growth requirements. The indicator enzymes for this analysis are β-1,4-glucosidase (BG), leucine aminopeptidase (LAP), and β-1,4-N-acetylglucosaminidase (NAG). Substrate uptake is related to the activity and concentration of enzymes. BG enzymes mediate the acquisition of C and have been used as a soil quality indicator because they are cosmopolitan and widely distributed in ecosystems. The enzymes LAP and NAG mediate N acquisition and are indicators of the shift to microbial N-immobilization. The indicator enzyme activity will be measured by the method described by Tabatabai (1994)15 and modifications of Sinsabaugh et al., (1991, 2008)16,17. Three different substrate analogs for each enzyme will be used: p-nitrophenyl-β-D-glucoside for BG, L-leucine-AMC for LAP, and 4-methylumbelliferone for NAG. Measurements of the enzyme concentration will be through colorimetry (BG) and fluorescence emission (LAP and NAG). For this analysis, microbial biomass C by chloroform fumigation and extraction, as described above, will be used as well. The CUE calculations will be fitted to a Michaelis-Menten model, as Geyer et al. (2019)18 describe.
The quantification of the cover crop’s litter decomposition and N-mineralization (Objective 1 and 3) within the nitrogen fertilizer rate treatments across sites was conducted with the buried litterbag technique (10 cm depth). For this evaluation, the litter of cover crops was collected from field sites before termination, air-dried, and stored. The C and N concentrations of cover crop litter from the first year were measured with a dry combustion elemental analyzer. Each experimental plot received a set of litterbags containing three standard cover crop litters with different C: N ratios (~10:1; 20:1 and 40:1). For each litter type, five grams of litter were transferred into 1 mm mesh litterbags, and the bags were buried in plots at the time of N fertilizer addition (4 weeks after corn planting). Five litterbags of each C: N level were placed in each plot to allow for periodic removal over the course of the growing season. Each set of litterbags was placed in the three replicated plots of three levels of N addition (0, 120, and 240 lb N/ac). Carbon and nitrogen release curves from the litterbags over time were used to calculate CUE based on the equation described by Manzoni et al., (2008)19.
Update 2024: Litter bags from the second year were weighed, grounded, and analyzed through the Unicube. The statistical analysis for decomposition curves is being developed.
Next steps: The analysis and comparison of two years will be finalized by April 2024.
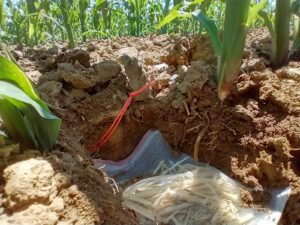
The Next Generation Sequencing analysis (Objective 2) evaluated the soil microbial community's size, composition, and structure. DNA will be extracted using DNA extraction kits according to the manufacturer’s instructions. Having a specific interest to determine the genetic potential for nitrification, a PCR of variants of the functional gene amoA (found in ammonia-oxidizing bacteria and archaea) was evaluated as described by Monteux et al. (2020)20. The abundance and amplification of the V3 region of the 16S ribosomal RNA to characterize bacterial communities and ITS1 region fungal communities were assessed. Primers, conditions for PCR, clean-up, and preps for sequencing were developed and performed with the guidance of professor Dr. Bell and post-doc Dr. King (see commitment letters) from the Department of Plant Pathology and Environmental Microbiology. The Genomics Core Facility at Penn State conducted amplicon sequencing on the MiSeq-250 platform (Illumina). The bioinformatics pipeline was performed using UCHIME databases. The analysis will be computed using DADA and RStudio. Only data from 2021 was analyzed for the Next Generation Sequencing, samples from 2022 are scheduled to be analyzed in fall 2023. I will compare the sequencing analysis to the microbial characterization by phospholipid fatty acid (PLFA) analysis performed by the N decision-support tool project. As PLFA is less expensive and used by farmers but cannot represent a specific species and their functional properties such as N-oxidizing capacity, comparing both methods could reflect functional groups' similarities and dissimilarities and provide information to develop linkages for future studies.
Update 2024: The samples from the microbial community for 2022 have not yet been analyzed through NGS.
Next steps: I expect to have done this analysis by May 2023.
At harvest season, corn yields (Objective 4) were measured per plot in an area of two rows by 5 m. Plants were hand cut off above brace roots, separating ears from the rest of the biomass. I weighted grain from the corn ears to obtain yield estimations. The hypothesis of the grain yield response to N fertilizer additions is that yield will increase up to the level of N recommended by the current decision support tool and then plateau at higher N rates. Regression analysis was used to determine the N rate at which yield plateaued in the experiment, and that were compared to the N rate recommended by the decision support tool. Furthermore, the decision support tool will be modified to use the CUE measured in the experiments to determine if this improves the accuracy of the decision support tool.
Statistical Analysis
Data sets will be analyzed using RStudio and SAS statistical software. A Generalized Linear Mixed Model will be used to analyze data in a randomized block split-plot in time design with fixed effects of the nutrient management levels, sampling dates, location, year, and their interactions, and a random effect of block and the interaction block x nutrient management level. Separations of least-square means will be conducted using the least significant difference by Fisher (α = 0.05). Other statistical approaches will be conducted, such as regression analysis, unconstrained and canonical ordination analysis to interpret the microbial community characterization and the CUE variation according to soil inherent properties, the response to management practices, and the relationship with yields observed.
The following results and discussion are based on preliminary results.
Soil Properties
The different content of soil organic matter and soil texture types across the on-farms and experiments allow us to measure microbial carbon use efficiency (CUE) through a range of soil textures. Tables 1 to 4 resumes different soil parameters to describe each site used for this study.
Table 1. Soil chemical analysis for the five locations used during the first year of study.
Year 1 (2021) | |||||
On-farm 1 | On-farm 2 | Rock Spring - Exp A | Rock Spring - Exp B | Rock Spring - Exp C | |
OM (%) | 3.28 | 1.77 | 2.25 | 2.20 | 2.34 |
pH | 6.74 | 6.88 | 6.48 | 6.69 | 6.45 |
P (ppm) | 82 | 95 | 36 | 37 | 42 |
K (ppm) | 123 | 141 | 150 | 130 | 155 |
S (ppm) | 13 | 9 | 8 | 8 | 9 |
Mg (ppm) | 130 | 77 | 111 | 153 | 106 |
Ca (ppm) | 1759 | 1162 | 1100 | 1258 | 1075 |
CEC (meq/100g) | 11.25 | 7.3 | 9.1 | 9.5 | 9.0 |
On-farm 1 refers to the farm at New Holland, on-farm 2 refers to the farm at Muncy, while Experiment A is the plots under triticale monoculture, Experiment B is the plots under crimson clover monoculture, and Experiment C is the plots in fallow from the Cover Crop Cocktail Experiment in Rock Spring.
Table 2 - Soil particle size analysis for the five locations for the first year of study.
Year 1 (2021) |
|||||
On-farm 1 | On-farm 2 | Rock Spring - Exp A | Rock Spring - Exp B | Rock Spring - Exp C | |
Sand (%) | 17 | 67.25 | 37 | 35.2 | 30.6 |
Silt (%) | 53.4 | 21.25 | 38.3 | 41.2 | 43.1 |
Clay (%) | 29.6 | 11.5 | 24.7 | 23.6 | 26.3 |
On-farm 1 refers to the farm at New Holland, on-farm 2 refers to the farm at Muncy, while Experiment A is the plots under triticale monoculture, Experiment B is the plots under crimson clover monoculture, and Experiment C is the plots in fallow from the Cover Crop Cocktail Experiment in Rock Spring.
Table 3. Soil chemical analysis for the five locations used during the first year of study.
Year 2 (2022) | |||||
On-farm 1 | On-farm 2 | Rock Spring - Exp A | Rock Spring - Exp B | Rock Spring - Exp C | |
OM (%) | 2.97 | 2.61 | - | - | - |
pH | 6.86 | 7.15 | - | - | - |
P (ppm) | 75 | 64 | - | - | - |
K (ppm) | 96 | 99 | - | - | - |
S (ppm) | 17 | 9 | - | - | - |
Mg (ppm) | 123 | 153 | - | - | - |
Ca (ppm) | 1543 | 1503 | - | - | - |
CEC (meq/100g) | 9.5 | 9 | - | - | - |
On-farm 1 refers to the farm at New Holland, on-farm 2 refers to the farm at Turbotville, while Experiment A is the plots under triticale monoculture, Experiment B is the plots under crimson clover monoculture, and Experiment C is the plots in fallow from the Cover Crop Cocktail Experiment in Rock Spring.
Table 4 - Soil particle size analysis for the five locations for the first year of study.
Year 2 | |||||
On-farm 1 | On-farm 2 | Rock Spring - Exp A | Rock Spring - Exp B | Rock Spring - Exp C | |
Sand (%) | 28 | 20 | - | - | - |
Silt (%) | 46 | 50 | - | - | - |
Clay (%) | 26 | 30 | - | - | - |
On-farm 1 refers to the farm at New Holland, on-farm 2 refers to the farm at Turbotville, while Experiment A is the plots under triticale monoculture, Experiment B is the plots under crimson clover monoculture, and Experiment C is the plots in fallow from the Cover Crop Cocktail Experiment in Rock Spring.
Results from the 2021 and 2022 field experiment to address Objective 1
The different cover crop practices across the two on-farm locations for the two years of study (Table 5) show the wide range of cover crop N content from the biomass of the species selected. The carbon and nitrogen release curves (Figure 4) conducted per site, according to the cover crop type (range of initial C: N ratio) and the supplemental N addition, showed the amount of N supplied by the cover crops litter to the soil ecosystem. This method allowed us to estimate the microbial CUE (Figures 5 and 6), a key parameter to adjust the nitrogen fertilizer recommendation tool. Within the results from the litterbag study, the key findings were that higher values of microbial CUE cause N tie-up, while lower values of CUE promote N supply. The threshold CUE, which causes N tie-up, will vary from soil to soil. Cover crops with lower C: N values (16) had a stable CUE as fertilizer additions increased (Figure 3), while cover crops with higher C: N values (42) had an increase of CUE when adding more N fertilizer. When cover crops' C: N ratio is high, microbes may need more N fertilizer to efficiently utilize the C in the residues. Litter from cover crops grown on each farm had a significantly higher CUE. This could be because that microbial community is adapted to consume that cover crop type.
Table 5 - Summary of the cover crop type used for the different on-farm locations and the contribution of nitrogen to the system.
Year 1 |
Year 2 |
|||
On-farm 1 | On-farm 2 | On-farm 1 | On-farm 2 | |
Cover crop type | a mix of crimson clover and annual ryegrass | oats | mix five species (legumes, grasses, and brassica) | mix five species (legumes and grasses) |
Cover crop N content (lbs N/ac) |
90 | 76 | 56 | 193 |
Cover crop C: N ratio | 15:1 | 17:1 | 14:1 | 14:1 |
Cover crop termination | April 7th | May 13th | April 9-11th | May 20* |
Results from the 2021 and 2022 field experiment to address Objective 4
The unfertilized yield for on-farm 1 was consistent for both years, resulting in 220 bu/ac for the first year and 217 bu/ac for the second year. The unfertilized yield at the on-farm 2 was 174 bu/ac for the first year and 130 bu/ac for the second year. Table 6 summarizes the experimental yield with unfertilized plots, the corn yield goal where the response curve reaches the plateau, and the N supplemental addition required to achieve the corn yield goal (Nx). The response curve was developed for each on-farm site per year; Figure 7 shows an example. A scatter plot comparing the unfertilized corn yield observed to the predicted unfertilized corn yield from the decision tool (Figure 8) resulted in an R2 = .47 (p-value=0.003), with a linear equation of y=0.68x+73.72. The estimated values from the decision tool are derived from estimated CUE values that predict cover crops and soil organic matter credits. To evaluate how adjusting CUE in the decision support tool with field-measured CUE from the litterbag study could improve the tool's prediction, two different regression analyses were compared: 1. using measured litterbag CUE to predict both cover crops and soil organic matter credits, and 2. using litterbag CUE only to estimate cover crop credits, while using tool's biogeochemical equations to estimate soil organic matter credits. The first analysis showed an R2=0.19 (p-value=0.08) and the linear equation y=1.16x - 41.82, indicating a poor fit using litterbag CUE to estimate cover crops and soil organic matter credits. However, the latter analysis showed an R2=0.36 (p-value=0.01) with the linear equation y=0.81x+44.23 (Figure 9), indicating a better fit even than when the tool's equation estimated for both types of N credits (cover crops and soil organic matter). The better fit could be due to litterbag CUE values coming directly from cover crops litter, while estimated CUE from biogeochemical equations in the tool use soil texture. Soil texture impact directly the decomposition of soil organic matter pools.
Year 1 | Year 2 | |||
On-farm 1 | On-farm 2 | On-farm 1 | On-farm 2 | |
Experimental Y0 (bu/ac) | 220 | 174 | 217 | 130 |
Corn yield goal (plateau) (bu/ac) | 240 | 200 | 230 | 158 |
Experimental Nx (lbs N/ac) | 110 | 25 | 0 | 4 |
Results from the 2021 field experiment to address Objective 2
The Next Generation Sequencing analysis was used to evaluate the soil microbial community. Preliminary results (Figure 10) showed slight changes in the relative abundance of the bacterial community (family level) across the different N additions (p-value > 0.05) but a significant change between on-farms (p-value = 0.001) and lapse of time from cover crop termination to the corn harvest (p-value=0.001). Interestingly, the bacterial community presented an increase in the relative abundance from base soil samples obtained a couple of weeks before cover crops were terminated and before corn was planted to soil samples obtained when corn was growing. Living vegetation such as corn and cover crops provides highly labile C sources through root exudates; however, comparing the root system from both types of crops, corn could provide more inputs of labile C sources that provide an energy source to microorganisms. Another aspect to consider in this preliminary analysis is the behavior of bacteria from the family Nitrosomonadacea. The Nitrosomonadacea family group is responsible for transforming ammonia to nitrate. The group showed a decrease in relative abundance while increasing the supplemental N addition; this could be related to the nitrate added in fertilizer. Further analysis should be conducted to assess whether microbial communities (including fungi) respond to different availability of carbon and nitrogen resources and determine how it could influence microbial carbon use efficiency.
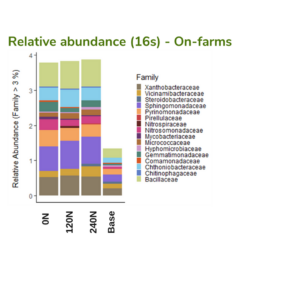
The research has not been completed to provide a conclusion.
Education & Outreach Activities and Participation Summary
Participation Summary:
1. Co-learning workshop – This workshop will be organized to share academic and farmer learning experiences from the results of this project. The audience (potentially 25) for this workshop will be farmers and Extension personnel. Both on-farm collaborators hosting research plots are active members in regional organic farmer learning networks facilitated by Extension Educators that are team members in the USDA-OREI project we are involved in, and we will use these relationships to organize the workshop within the regular programming of these learning networks. We will discuss the dynamics between CUE, N-mineralization, and microbial community composition. Furthermore, the discussion will center on the behavior of these parameters according to cover cropping and nutrient management evaluated in this study. Additionally, participants will use their data or provided data in the decision-support tool to test cover cropping and nitrogen management scenarios for their farms and provide feedback about the results of the tool and how it compares to their experiences with nitrogen management on their farms. The purpose of promoting engagement with the decision-support tool is to determine the best sustainable practices for nutrient management and collect their feedback and needs. The workshop will be evaluated for efficacy in knowledge and engagement.
2. Farmer conference workshop – A conference workshop will be developed that aims to share the knowledge derived from this research and collect feedback from farmers. The conference will discuss the dynamics between CUE, N-mineralization, and microbial community composition as affected by soil properties and agricultural management. The conference workshop will be evaluated for efficacy in knowledge and engagement. Involvement in farmer conferences will be facilitated by Dr. White, who is an Extension Specialist on the Penn State Agronomy Team and organizes numerous farmer conferences each year. For example, in February 2022, I and a post-doctoral researcher collaborating with my work (Dr. Kathleen Arrington), shared preliminary results of my research and demonstrated how these results are used in the new N fertilizer recommendation tool in a session of the “Making Cover Crops Pay” extension webinar series, reaching an audience of approximately 100 farmers and agronomists. The plan is to develop a similar conference workshop, or online webinar, for next year as well.
3. Fact sheet – I will create an online fact sheet intended for farmers and Extension personnel to provide information about the knowledge derived from this study and examples of using the decision-support tool based on different scenarios of on-farm management observed in the research. The different case studies will span different cover cropping practices on different soil textures and provide an overview of the yield results obtained according to N supplemental additions. The information provided in the fact sheet will enhance the use of the decision-support tool and sustainable nutrient management.
**A preliminary fact sheet was created for a farmer's meeting scheduled on December 16th, 2022 but it has to be rescheduled for 2023 due weather conditions.
4. Academic meeting – The scientific results were presented in the SSSA-ASA-CSA meeting in November 2022 to make researchers aware of the relationships between microbial CUE, N mineralization, and the ability of the N decision support tool to use these measurements to recommend N-fertilizer rates.
Project Outcomes
Preliminary results from the litterbag study (2021) improve the calibration of the n-tool. We want to compare the results from the litterbag study during the on-farms for 2022 and the other methods to evaluate microbial carbon use efficiency.
Preliminary results of the litterbag study provide a better understanding of the behavior of microbial carbon use efficiency and how it is related to nitrogen supply.