Final report for GNE22-298
Project Information
Increasing functional diversity is essential to enhance ecosystem services and reduce agriculture’s environmental footprint while simultaneously meeting a growing demand for food. One way to achieve this is through the integration of cover crop mixtures into farming rotations. Cover crops provide a multitude of ecosystem services to cropping systems, including nitrogen retention and fixation, weed suppression, reduction in pest pressure, and increased profitability of cash crops.
Here, we evaluated how different cover crop treatments grown in mixtures and monocultures impacted three ecosystem services: weed seed mortality in the soil, nitrogen fixation and uptake between species, and soil labile carbon for the following cash crop. These services are microbially mediated and may be enhanced by increasing species' functional diversity. In 2023, we found that cover crop treatment had a significant effect on giant foxtail (Setaria faberi) weed seed viability, with higher seed viability in canola monocultures than fallow (Spring 2023) or clover (Fall 2023). However, cover crop treatment had no effect on S. faberi viability (Spring 2024) or on Powell amaranth (Amaranthus powellii) seed viability (2023 and Spring 2024). We found no difference in percent of N derived from fixation in either crimson clover or pea when grown in monoculture or mixture. However, the clover and pea monocultures had greater N derived from fixation compared to the three and four species mixtures. Finally, we found no effect of cover crop treatment on labile carbon in either 2023 or 2024. Preliminary results from this study were communicated to the grower community and agricultural professionals through research field tours at the Cover Crop Cocktails field site, a PSU Extension Webinar, and a multi-day presentation at PSU's 2024 Ag Progress Days.
Objective 1: Evaluate how weed seed mortality differs among cover crop mixtures and monocultures.
Hypothesis 1: We expect that weed seed mortality will be greater in cover crop mixtures compared to monocultures. Presumably, the greater functional diversity and abundance of roots in mixtures will increase weed seed mortality by increasing microbial diversity and activity. Additionally, we expect that both legumes and brassicas will increase weed seed mortality relative to grasses; legumes increase N, and in turn microbial activity, and brassicas associate with saprophytic fungi which are primarily responsible for breaking down recalcitrate organic matter (such as weed seeds) in the soil.
Objective 2: Evaluate how biological nitrogen fixation (BNF) of legumes in mixtures compares to legume monocultures and determine how much N from fixation is shared between species within mixtures.
Hypothesis 2: Relative to cover crop monocultures, legume species will have a greater percent of N derived from BNF when mixed with grass and/or brassica due to the drawdown in soil available N. Other species planted with legumes will also have higher N than those planted in monoculture or with other non-legumes due to acquisitioning BNF–derived N exuded from legume nodules.
Objective 3: Evaluate how labile carbon differs among cover crop mixtures and monocultures.
Hypothesis 3: Relative to cover crop monocultures, mixtures will promote higher labile carbon pools due to the greater diversity of cover crop residue inputs (varying in chemical composition) incorporated into the soil.
Objective 4: Evaluate whether cover crop mixtures can increase the diversity and breadth of the ecosystem services they provide (i.e. can mixtures increase multifunctionality).
Hypothesis 4: Relative to cover crop monocultures, cover crop mixtures will increase weed seed mortality, labile carbon, and biological nitrogen fixation ecosystem services. We predict that the provisioning of any one service will be lower in mixtures compared to the best performing monoculture. However, when considering all of our measured services mixtures will exhibit greater performance.
The purpose of this project was to examine whether various cover crop monocultures or mixtures promoted three microbially-mediated ecosystem services: weed seed mortality, biological nitrogen fixation (BNF), and soil labile carbon (C). This research contributed to agricultural sustainability by evaluating whether the use of diverse cover crop species in crop rotations would achieve a suite of ecosystem services to growers, which would be essential to meet a growing food demand while reducing the environmental footprint of agricultural systems (Finney et al. 2017; Finney & Kaye 2017; Davis et al. 2012).
Cover crop adoption is highest in the northeast, partly driven by incentive programs and regulations governing nutrient management in the Chesapeake Bay watershed (Hamilton et al. 2017; Myers et al. 2019). While soil health and weed management are the primary motivators that growers cite for using cover crops (CTIC et al. 2020), cover crops serve a multitude of additional related functions such as diversifying cropping rotations, retaining nitrogen (N), adding N through fixation, reducing pest pressure, and increasing profitability of cash crops (Finney et al. 2017; Finney & Kaye 2017). However, cover crop species vary in their provisioning of ecosystem services. For example, grasses are particularly effective at scavenging inorganic nitrogen and suppressing weeds, while legumes provide N via biological N fixation. Combining diverse cover crop species enables a wider array of services to be achieved, otherwise referred to as ‘multifunctionality’ (Finney & Kaye 2017). Despite this, to date, there is conflicting evidence whether cover crop mixtures provide increased multifunctionality when compared to their cover crop monoculture counterparts (Blesh et al. 2019; Finney et al. 2017; Florence & McGuire 2020).
Despite the role that cover crops play in suppressing growing weeds and breaking up weed life cycles, thus far, research is limited on how varying cover crop species affect rates of weed seed mortality in the soil (Mohler et al. 2018; Sias et al. 2021). The seed and seedling stage are the most vulnerable stages in weed life cycles (Gallandt 2006; Davis et al. 2008; Sarabi 2019); therefore, targeting weed seeds in the soil seedbank can result in substantial declines in weed population densities. It is well established that the plant community has strong effects on the soil microbial community, and because weed seed mortality is a microbially mediated process, it is therefore possible that varying cover crop species may differ in their effects on weed seed mortality rates in the soil either through effects on soil microbes or via effects on soil abiotic factors (inorganic N and moisture) that may influence seed mortality.
Nitrogen is the most limiting nutrient to plants in agroecosystems, and legumes play an important role as a source of N through fixation. In organic systems, legumes can be one of the primary sources of N inputs, while in conventional systems including legumes in crop rotations can offset fertilizer inputs. Previous work shows that intercropping legumes with grasses can facilitate sharing of fixed N between species and increase rates of BNF (Blesh et al. 2019). While numerous studies have examined N fixation rates in grass and legume bicultures, thus far, research is limited on how more complex mixtures influence N fixation.
Finally, crop productivity and sustainability are tightly linked to soil organic matter (Culman et al. 2013), and labile C is an important predictor of soil health. Additionally, labile C is an ideal component of the soil organic matter pool to measure because it is a proxy for labile N and is more likely to exhibit quantifiable changes following management on a short time scale than renewable stable nutrient pools (Culman et al. 2013; Wander & Drinkwater 2000).
In this research, we focused on three essential, but relatively less studied ecosystem services: weed seed mortality, BNF, and labile C. We selected these three services because they have the potential to greatly increase crop productivity and profitability. Additionally, the three ecosystem services we evaluated within this study are all microbially mediated, and there is an abundance of work showing that plant diversity increases microbial diversity and functioning (Cantarel et al. 2015; Bargaz et al. 2017; Blesh et al. 2019; Freschet et al. 2021). Therefore, we hypothesized that more diverse cover crop mixtures would enhance the functioning of these ecosystem services.
Cooperators
- (Educator and Researcher)
Research
Site characterization and experimental design: All research objectives were performed in the existing organic long-term cover crop cocktails experiment (CCC) located at the Russell E. Larson Agricultural Research Center at Rock Springs, PA. The existing CCC experimental design is comprised of a three-year corn, soybean, winter wheat rotation (Murrell et al. 2017). The experiment was established in 2011 at Penn State University to determine whether diverse cover crop mixtures, as opposed to single-species cover cropping, can enhance ecosystem functions in a corn-soybean-wheat cash crop rotation that produces organic feed. The selected cover crop mixtures target nutrient supply, nutrient retention, weed suppression, and management ease to investigate whether diverse mixtures provide these functions better than cover crops in monoculture. Experiments in CCC have measured total N, C:N in cover crop aboveground biomass, soil weed suppression, and cash crop production and profitability. We added the services of BNF, and labile C to the service measurement suite.
We used a subset of eight existing treatments that only contained cover crops which over-wintered or tolerated mild winters (in the case of Austrian winter pea). In addition to overwintering, these four species were selected based on their functional diversity (Amsili & Kaye 2020). Cover crop treatments included three mixtures and four monocultures (listed below). Each treatment was replicated across four blocks and organized in a random split plot design.
Cover crop treatments:
- triticale
- crimson clover
- Austrian winter pea
- canola
- three species mixture (triticale, crimson clover, and Austrian winter pea)
- four species mixture (triticale, crimson clover, Austrian winter pea, and canola)
- fallow control
The experimental design of this project was created through a collaboration between scientists at Penn State and organic growers on the board of this project to ensure that the design yielded meaningful information and addressed grower questions.
Plant tissue sampling and processing (Objectives 2): Within each treatment plot, we established two 1 m2 subplots, one for fall and one for spring sampling. Subplots were hand-weeded once a season (we weeded the 1m2 subplot as well as an additional area of approximately 0.5 m in each direction) to ensure that any differences in ecosystem services were due solely to the cover crops, and not due to variation in the weed community within cover crop stands. Within the 1 m2 subplots, we collected stand counts in fall, and aboveground biomass at both the fall and spring timepoints. Aboveground biomass was sorted by species, dried, and weighed. Soil core samples were taken from all treatments aside from the fallow control. Sampling was conducted over two time points: at fall frost, and before cover crop termination in the spring (Figure 1). At fall and spring sampling, two cores were taken in the planting row and two between planting rows for an accurate root composition estimate (Amsili & Kaye 2020). We divided cores to three depths (0-5, 5-20, 20-40 cm) to understand how roots were dispersed vertically. Collected soil core samples were washed, dried, and weighed.
Emma Rice at Cover Crop Field Site
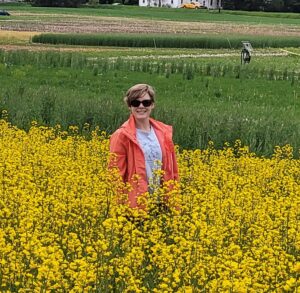
Weed seed mortality (Objective 1): We selected Amaranthus powellii (broadleaf) and Setaria faberi (grass) as target weed species because they are summer annual weeds which are abundant during growth of summer annual cash crops and are challenging to control organically. Using the weed seed burial bag method (Davis et al. 2005), we installed mesh bags in each plot (2 bags/species/plot; 128 total) containing 200 seeds combined with 10 g soil. Soil was collected in the fall directly from each plot where the bag was later installed to ensure the soil in the weed seed burial bags reflected the soil chemical and microbial composition of the soil within each cover crop treatment. Bags were buried at depth soon after fall planting and strategically located where all species in the mixture were present. The burial depth was selected so that seeds were in an area of high microbial activity. To minimize the possibility of disturbance from field equipment, bags were placed close to the planting row. All bag locations were marked with flags (Figure 2).
We evaluated weed seed mortality at two timepoints: 1) prior to cover crop termination (May), to evaluate the effect that living cover crops have on weed mortality; and 2) at corn harvest (October), to evaluate the effect that cover crops have on weed seed mortality after incorporation into the soil. All seed bags were retrieved at spring cover crop termination, but only half of the bags were processed at that time (Timepoint 1 above). In Year 1 (2023), the second half was kept in dark, cool conditions (black bags in a larger refrigerator) mimicking conditions in the soil before being redeployed into the same plot following corn planting (Figure 1). In year 2 (2024), instead of storing the second half of seed bags, we made new seed bags (with dry seed and soil) to deploy after the corn was planted to try to minimize temperature shock and light signals at burial that may have signaled increased germination in 2023. The remaining seed bags were retrieved just prior to corn harvest (Timepoint 2). Using sieves (0.5 - 1mm) and hand picking, we washed away soil from the seeds and conducted recovery counts. We then performed tetrazolium (TZ) viability testing as per AOSA handbook guidelines (Miller 2010), with a slight modification for A. powellii (pierce with needle instead of bisect seed). Total viability was calculated as % recovery x % TZ viable. Data was analyzed via generalized linear mixed effects models (with treatment as a fixed effect and block as a random effect) with a beta distribution, which is recommended for analysis of proportional data.
N fixation (Objective 2): We estimated the proportion of N derived from N fixation within legume plants and their neighbors using the 15N natural abundance approach, which is commonly used to estimate N fixation of legumes grown in both monocultures and mixtures.
For all mixtures containing legumes, dried shoot tissue was ground separately by species (52 samples/year) and sent for analysis at the Cornell University Stable Isotope Laboratory. We then used the equation below to calculate the percent of nitrogen derived from the atmosphere (%Ndfa) and estimated the total N contribution by BNF (Pinto et al. 2021):
%Ndfa = 100[(δ15Nref - δ15Nleg) / (δ15Nref - B)]
In equation one, δ15Nref is the 15N relative natural abundance of the reference plant (triticale grown in monoculture and averaged from the four blocks, %Ndfa=0) to estimate standard background δ15N (Blesh et al. 2019; Peoples et al. 1995). δ15Nleg is the legume’s 15N relative natural abundance, and B is the δ15N relative natural abundance of the legume when it is grown in a N-free medium (%Ndfa=100). The B was calculated previously for both crimson clover (Blesh et al. 2019; Pinto et al. 2021; Huss-Danell 2007) and Austrian winter pea (Blesh et al. 2019). After we calculated the relative natural abundance (%Ndfa), we quantified BNF using the following equation:
BNF (kg ha-1) = biomass (kg ha-1) • plant N concentration (%)/100 • %Ndfa/100
In summary, we calculated the biological nitrogen fixation (BNF) in kilograms per hectare by multiplying the plant biomass with the proportion plant N concentration and multiplying that with the previously calculated proportion atmospheric derived N. This final output of BNF was compared by species between treatments to see if certain mixtures promote higher BNF rates.
Labile Carbon (Objective 3): To measure labile C, soil samples were taken from 0-15 cm depth using a 2.5 cm diameter soil probe in each cover crop treatment two weeks after cover crops were incorporated into the topsoil (Figure 1). Twelve soil cores were randomly collected from each cover crop plot, combined, and homogenized. For each plot, we mixed 20 ml of potassium permanganate (KMnO4) with 2.5 g of soil, agitated for 2 minutes, and allowed all solid material to settle on the bottom of the tube (Culman et al. 2012; Weil et al. 2003). The liquid directly above the solid material was collected and sample absorbance was read with a SpectraMax M5 spectrophotometer at 550 nm (Culman et al. 2013). Labile soil organic matter plays a key role in crop nutrient acquisition and is more likely to show changes following management adjustments on a shorter time scale compared to renewable stable nutrient pools.
Data Analysis:
For each ecosystem service measurement, we performed an analysis of variance (ANOVA) to compare the cover crop treatments to the response variable (weed seed mortality, BNF, labile C absorbance measurement) with treatment as a fixed effect and block as random effect. Additionally, we visualized the response for each treatment and performed pairwise comparisons to evaluate treatment differences.
Weed Seed Mortality:
We expected that weed seed mortality would be greater in cover crop mixtures compared to monocultures. However, we did not detect any consistent increase or decrease in cover crop mixtures compared to the cover crop monocultures. Instead, we found greater S. faberi seed viability when seeds were buried in the canola monoculture in both extraction timepoints in 2023. After 6 months of burial (May 2023), Setaria faberi seed viability was significantly lower in the fallow treatment compared to the canola treatment (p=0.015), but we detected no other differences among cover crop treatments (Figure 3). After 1 year of burial (Fall 2023), S. faberi seed viability was significantly lower in the clover treatment compared to the canola treatment (Figure 4, p=0.028), but we detected no other differences among cover crop treatments. In Spring 2024, cover crop treatment had no effect on S. faberi seed viability (Figure 5, p= 0.239). Additionally. cover crop treatment had no effect on Amaranthus powellii seed viability in Spring 2023 (Figure 6, p=0.199), Fall 2023 (Figure 6, p= 0.294), or Spring 2024 (Figure 8, p= 0.356).
It's possible that we only saw an effect of canola in 2023, but not in 2024, because canola stands were lower in the second year due to poor emergence. More research is needed to determine whether high canola density and biomass can negatively impact weed seed mortality. Glucosinolates are antimicrobial secondary metabolites often found in high abundance in brassicas, which can impact the soil microbial community (Poveda et al. 2020). These compounds have been shown to be beneficial by reducing pathogens that might negatively affect the subsequent cash crop. However, it’s possible they may also suppress seed microbial pathogens that contribute to weed seed bank control. Future research should determine whether high canola density and biomass can impact weed seed mortality, and whether this effect is consistent across brassica species and varieties.
Figure 3. S. faberi seed viability from Spring 2023 (0.5 years burial). Treatment had a significant effect on weed seed viability. Seed viability in the canola treatment was significantly higher than seed viability in the fallow treatment (ANOVA, df =6, p = 0.015).
Figure 4. S. faberi seed viability from Fall 2023 (after 1 year burial). Treatment had a significant effect on weed seed viability. Seed viability in the canola treatment was significantly higher than in the clover treatment (ANOVA df=6, p=0.028).
Figure 5. S. faberi seed viability from Spring 2024 (0.5 year burial). Treatment had no effect on weed seed viability (ANOVA df=6, p=0.239).
Figure 6. A. powellii seed viability from Spring 2023 (0.5 years burial). Treatment had no effect on weed seed viability (ANOVA, df = 6, p=0.199).
Figure 7. A. powellii seed viability from Fall 2023 (after 1 year burial). Treatment did not have a significant effect on weed seed viability (ANOVA, df = 6, p=0.294).
Figure 8. A. powellii seed viability from Spring 2024 (0.5 years burial). Treatment had no effect on weed seed viability (ANOVA, df = 6, p=0.356).
Biological Nitrogen Fixation:
We expected that legumes in mixtures with a competitive grass and/or brassica species to fix more nitrogen because available soil nitrogen would be reduced by the other non-N fixing species in the mixture. However, contrary to our hypothesis and in both Fall 2022 and Spring 2023 (year 1), we found no difference in percent N fixation of crimson clover (p=0.144) or winter pea (p=0.289) when in monoculture or mixture (Figures 9 and 10). When looking at total N derived from fixation on a per area basis (kg N ha-1), in the Fall there was a significantly greater fixed nitrogen in the clover monoculture treatment than clover in the three (p=0.095) and four (p=0.011) species mixtures (Figure 11). A difference in total BNF was also seen in the Fall between the pea monoculture and mixture treatments (both mixtures p<0.001), with the monoculture treatment containing more fixed nitrogen per unit area (Figure 12). This difference was driven by the larger biomass of crimson clover and winter pea in the monoculture treatments compared to their biomass when in mixtures. In the Spring, there were no significant differences in N fixation because much of the clover in monoculture winter-killed. Almost all of the pea winter-killed, therefore N fixation from pea in Spring was minimal. We did not find higher atmospheric nitrogen (%Ndfa) in non-legume species when grown in mixtures with legume species compared to when grown in monoculture treatments (data not shown). BNF analysis is ongoing for year 2 (Fall 2023 – Spring 2024).
Figure 9. Percent nitrogen fixation in crimson clover (cc) from the fall 2022 to spring 2023 across monoculture and mixture treatments. Treatment did not have a significant effect on percent fixation (p=0.144).
Figure 10. Percent nitrogen fixation in Austrian winter pea (WP) in fall 2022 across monoculture and mixture treatments. Treatment did not have a significant effect on percent fixation (p=0.289).
Figure 11. Nitrogen from fixation (kg N ha-1) in crimson clover (CC) from the fall 2022 to spring 2023 across monoculture and mixture treatments. Treatment had a significant effect on aboveground N fixation (p=0.005). In fall, nitrogen fixation in the monoculture treatment was significantly higher than clover fixation in both the three species (p=0.095) and four species mixtures (p=0.011).
Figure 12. Nitrogen from fixation (kg N ha-1) in Austrian winter pea (WP) in fall 2022 across monoculture and mixture treatments. Treatment had a significant effect on aboveground nitrogen fixation (p<0.001). In fall, nitrogen fixation in the monoculture treatment was significantly higher than winter pea fixation in both the three species (p<0.001) and four species mixtures (p<0.001).
Labile Carbon Analysis:
We expected that cover crop mixtures would increase mixtures labile carbon pools due to the greater diversity of cover crop residue inputs (varying in chemical composition) incorporated into the soil. However, we found no effect of cover crop treatment on soil labile carbon in either year 1 (late spring 2023) or year 2 (late spring 2024) (p=0.853; Figure 13). While POXC measurements suggests that the cover crop treatments do not vary in labile carbon, previous research suggests that the different cover crop functional groups may vary in their effects on soil particulate organic matter (Zhang et al. 2022), with different cover crop species contributing to different pools of particulate organic matter. Perhaps we would have detected differences between cover crop treatments if we had continued sampling throughout the corn growing season, as varying cover crop tissues further decomposed, or if cover crop treatments were in place more frequently than once every three years.
Figure 13. Labile carbon (mg/kg) across cover crop treatments. Treatment did not have a significant effect on active carbon (p-value = 0.853).
Data analysis for year two (BNF and Fall 2024 weed seed mortality) is ongoing and will not be discussed herein.
Overall, we found that cover crop mixtures did not increase or decrease the provisioning of three ecosystem services we measured: weed suppression via seed mortality, biological N fixation, and soil labile carbon. Soil labile carbon, measured with POXC showed no differences across any of the cover crop treatments, therefore, our results suggests that this should not be a deciding factor in whether to adopt cover crop mixtures.
No cover crop monoculture or mixture consistently increased weed seed mortality (decreased viability). While we found canola decreased weed seed mortality (increased viability) in one of the two years, this effect was not observed when canola was grown in mixtures, and this effect was inconsistent across weed species and years. This is not surprising given that canola stands were lower in the second year, and cover crops often demonstrate inconsistent effects between years. Canola can provide numerous other ecosystems services when grown in mixtures, such as decreasing N leaching and providing early floral resources to pollinators. Therefore, future research should evaluate whether high densities of canola can influence weed seed mortality, as well as potential tradeoffs with other ecosystem services that can occur when including canola in mixtures.
Individual legume species did not fix more N in mixture compared to when in monocultures. The amount of N introduced into the cropping system via N fixation was largely driven by the quantity of legume biomass, which was lower in our mixtures compared to the legume monocultures in the Spring. Lower seeding rates and potentially increased levels of interspecific competition may have both contributed to lower legume biomass in mixtures. N is one of the major factors limiting yields in organic systems, and legume N fixation is an important and economic source of N. Therefore, future research should examine whether more competitive and winter hardy legumes can increase N derived from fixation when grown in mixtures.
Farmer Impacts:
As farmer interest in cover crop mixtures is growing, our research suggests that cover crop mixtures did not increase nor decrease the performance of the three ecosystem services measured within our study (weed seed mortality, N fixation rates, and soil labile carbon). If farmers prioritize increasing plant available N via N fixation, then they should plant legume monocultures or cover crop mixtures with a high proportion of legumes. While we found canola decreased weed seed mortality (increased viability) in one of the two years, this effect was not observed when canola was grown in mixtures. Therefore, our research suggests any potential negative impacts of canola on weed seed mortality may be alleviated when canola is grown in mixtures. This is further evidence suggesting that the benefits from cover crop mixtures come from ameliorating dis-services rather than increasing individual ecosystem services associated with cover crop monocultures.
Citation List
Amsili, J. P. & Kaye, J. P. Root traits of cover crops and carbon inputs in an organic grain rotation. Renew. Agric. Food Syst. 1–10 (2020) doi:10.1017/S1742170520000216.
Bargaz, A. et al. Species interactions enhance root allocation, microbial diversity and P acquisition in intercropped wheat and soybean under P deficiency. Appl. Soil Ecol. 120, 179–188 (2017).
Blesh, J., VanDusen, B. M. & Brainard, D. C. Managing ecosystem services with cover crop mixtures on organic farms. Agron. J. 111, 826–840 (2019).
Cantarel, A. A. M. et al. Using plant traits to explain plant-microbe relationships involved in nitrogen acquisition. Ecology 96, 788–799 (2015).
CTIC, SARE & ASTA. National cover crop survey annual report 2019-2020. https://www.sare.org/wp-content/uploads/2019-2020-National-Cover-Crop-Survey.pdf (2020).
Culman, S. W., Snapp, S. S., Green, J. M. & Gentry, L. E. Short- and long-term labile soil carbon and nitrogen dynamics reflect management and predict corn agronomic performance. Agron. J. 105, 493–502 (2013).
Culman, S. W. et al. Permanganate Oxidizable Carbon Reflects a Processed Soil Fraction that is Sensitive to Management. Soil Sci. Soc. Am. J. 76, 494–504 (2012).
Davis, A. S. et al. Environmental factors affecting seed persistence of annual weeds across the U.S. corn belt. Weed Sci. 53, 860–868 (2005).
Davis, A. S., Schutte, B. J., Iannuzzi, J. & Renner, K. A. Chemical and physical defense of weed seeds in relation to soil seedbank persistence. Weed Sci. 56, 676–684 (2008).
Davis, A. S., Hill, J. D., Chase, C. A., Johanns, A. M. & Liebman, M. Increasing Cropping System Diversity Balances Productivity, Profitability and Environmental Health. PLoS One 7, 1–8 (2012).
Drost, S.M., Rutgers, M., Wouterse, M., De Boer, W. and Bodelier, P.L. Decomposition of mixtures of cover crop residues increases microbial functional diversity. Geoderma, 361, p.114060 (2020).
Freschet, G. T. et al. Root traits as drivers of plant and ecosystem functioning: current understanding, pitfalls and future research needs. New Phytol. (2021) doi:10.1111/nph.17072.
Florence, A. M. & McGuire, A. M. Do diverse cover crop mixtures perform better than monocultures? A systematic review. Agron. J. 112, 3513–3534 (2020).
Finney, D. M. et al. Ecosystem Services and Disservices Are Bundled in Simple and Diverse Cover Cropping Systems. Agric. Environ. Lett. 2, 170033 (2017).
Finney, D. M. & Kaye, J. P. Functional diversity in cover crop polycultures increases multifunctionality of an agricultural system. J. Appl. Ecol. 54, 509–517 (2017).
Gallandt, E. R. How can we target the weed seedbank? https://doi.org/10.1614/WS-05-063R.1 54, 588–596 (2006).
Hamilton, A. V., Mortensen, D. A. & Allen, M. K. The state of the cover crop nation and how to set realistic future goals for the popular conservation practice. J. Soil Water Conserv. 72, 111A-115A (2017).
Huss-Danell, K., Chaia, E. & Carlsson, G. N2 fixation and nitrogen allocation to above and below ground plant parts in red clover-grasslands. Plant Soil 299, 215–226 (2007).
Miller, A. L. (ed) 2010. AOSA/SCST Tetrazolium Testing Handbook, 2010 edition. Tetrazolium Subcommittee of the Association of Official Seed Analysts and the Society of Commercial Seed Technologists
Mohler, C. L., Taylor, A. G., Ditommaso, A., Hahn, R. R. & Bellinder, R. R. Effects of Incorporated Rye and Hairy Vetch Cover Crop Residue on the Persistence of Weed Seeds in the Soil. Weed Sci. 66, 379–385 (2018).
Murrell, E. G. et al. Achieving diverse cover crop mixtures: Effects of planting date and seeding rate. Agron. J. 109, 259–271 (2017).
Myers, R., Weber, A. & Tellatin, S. Cover crop economics opportunities to improve your bottom line in row crops. https://www.sare.org/wp-content/uploads/Cover-Crop-Economics.pdf (2019).
Peoples, M. B., Herridge, D. F. & Ladha, T. K. Biological nitrogen fixation: An efficient source of nitrogen for sustainable agricultural production? Plant Soil 174, 2–28 (1995).
Pinto, P. et al. Variable root:shoot ratios and plant nitrogen concentrations discourage using just aboveground biomass to select legume service crops. Plant Soil 463, 347–358 (2021).
Poveda, J., Eugui, D. and Velasco, P. Natural control of plant pathogens through glucosinolates: an effective strategy against fungi and oomycetes. Phytochemistry Reviews, 19(4), pp.1045-1059 (2020).
Sarabi, V. Factors that influence the level of weed seed predation: A review. Weed Biol. Manag. 19, 61–74 (2019).
Sias, C., Wolters, B. R., Reiter, M. S. & Flessner, M. L. Cover crops as a weed seed bank management tool: A soil down review. Ital. J. Agron. 16, (2021).
Wander, M. M. & Drinkwater, L. E. Fostering soil stewardship through soil quality assessment. Appl. Soil Ecol. 15, 61–73 (2000).
Weil, R. R., Islam, K. R., Stine, M. A., Gruver, J. B. & Samson-Liebig, S. E. Estimating active carbon for soil quality assessment: A simplified method for laboratory and field use. Am. J. Altern. Agric. 18, 3–17 (2003).
Zhang Z, Kaye JP, Bradley BA, Amsili JP & Suseela V. Cover crop functional types differentially alter the content and composition of soil organic carbon in particulate and mineral‐associated fractions. Global Change Biology 28, 5831-5848 (2022).
Education & Outreach Activities and Participation Summary
Participation Summary:
Completed Outreach Efforts
The results of this research were shared by the graduate students Kara Eckert and Emma Rice (original grant recipient) with growers, extension agents, and other researchers in the Northeast through the following avenues:
PSU-Rodale Sustainable Agriculture Tour of Rock Springs – September 2023: Kara Eckert briefly presented the weed seed mortality portion of the project in Spanish during a tour of the cover crop test plots. The event was jointly organized by the Rodale Institute and PSU for visiting Argentinian farmers. No data were analyzed at the time, so a project overview was given, highlighting the potential importance of microbes in weed seed mortality.
Making Cover Crops Pay: Quantifying Cover Crop Ecosystem Services – February 2024: Emma Rice presented project findings during a Penn State Extension Making Cover Crops Pay webinar series on how cover crop ecosystem services are measured, how certain species are best at promoting specific services, and how to maximize service multifunctionality with strategic mixture design.
Penn State's Ag Progress Days – August 2024: Kara Eckert, Emma Rice, and other cover crop scientists presented research findings and guided a discussion each day of Ag Progress Days (3 days). About 30 farmers attended in total and we presented a poster on weed seed mortality and cover crop biomass allocation. We then answered questions and helped guide a discussion on cover crops.
Ag Progress Days 2024 Poster on Cover Crops
- Lancaster Farming Article on Ag Progress Days Presentation – August 16, 2024: A reporter from the Lancaster Farming newspaper attended our Ag Progress Days cover crop outreach event and wrote a summary article about our work. CCC APD Article in Lancaster Farming 2024
- Weed Seed Mortality Fact Sheet for Ag Progress Days – We also created a fact sheet to hand out to the public during the Ag Progress Days presentation which covered the main findings from the weed seed mortality project. CCC APD 2024 Weeds Fact Sheet
PSU-Rodale Sustainable Agriculture Tour of Rock Springs – September 3, 2024: Kara Eckert briefly presented data from the weed seed mortality portion of the project in Spanish during a tour of the cover crop test plots. The event was joint organized by the Rodale Institute and PSU for visiting Argentinian farmers. Printed graphs, example weeds, and sample seed bags were used to further educate attendees.
Future Outreach Efforts
Northeast Weed Science Society - January 2025: Emma Rice’s undergraduate mentee will present a poster on biological nitrogen fixation (year 1) and labile carbon ecosystem service measurements. Attendees will have a strong background in weed management, but varying familiarity with agriculture and ecosystem service research.
PSU Undergraduate Research Poster Exhibition – Spring 2025: Emma Rice's undergraduate mentee will present the biological nitrogen fixation (full dataset) and labile carbon ecosystem service measurements from this project. Attendees may or may not have a foundation in agriculture/cover crops so the mentee will adapt presentation style to facilitate understanding.
Publish in a peer reviewed journals – Once the second year of sampling is finished and data processed, we will publish our results in multiple papers, including a weed seed mortality paper, and a separate paper covering N fixation and labile C. The main audience for these manuscripts will be researchers and extension agents. The hope is that our work will promote future cover crop research investigating the relationship between plant functional diversity and microbially and root mediated ecosystem services.
Project Outcomes
In this study cover crop mixtures did not increase rates of weed seed mortality, the percent of N derived from fixation, or labile carbon. However, overall, they did not decrease these key ecosystem services either. Often mixtures are not the best at any one ecosystem service, but they may be best at minimizing the disservices of monocultures and produce a more middle ground effect that could be more resilient in a changing climate. However, more research is needed to determine whether cover crop mixtures can increase cover crop ecosystem multifunctionality.
During this project, we worked on optimizing TZ seed viability testing. The traditional method of bisecting Amaranthus seeds for staining resulted in too much cut damage of the embryo and the final staining was challenging to interpret. Also, it was too precise a procedure to allow assistance from undergraduate research assistants. We developed a pierce method to minimize disruption of the embryo while also allowing the TZ solution to enter the seed and stain tissue. Seeds were then disturbed after the TZ staining procedure to view the embryo, resulting in clearer staining patterns.
Through this, we learned that weed seedbank dynamics are complex and weed seed viability can vary greatly across fields of the same treatment. Research on weed seedbank control will require a more complex study of other factors influencing mortality, including seed microbes and soil abiotic factors, which we will investigate in NE SARE grant GNE24-308.