Final report for GNE22-304
Project Information
Higher frequency of temperature extremes under climate change poses great challenges to grape growing in Northeastern U.S. Among these temperature extremes, late spring frost directly threatens the survival of fragile but most fruitful primary buds, leading to significant crop losses when damage occurs. However, current management methods are either not applicable or not economically friendly to the growers with established smaller vineyards, compromising their use in Northeastern U.S. Some budbreak delaying products were recently evaluated for their potentials to mitigate spring frost damage in grapevine. Among these products, abscisic acid (ABA) analogs exhibit both ease of application and efficacy of budbreak delay in preliminary testing; two characteristics favored by growers. In this project, we examined the efficacy of a synthetic abscisic acid (ABA) analog, tetralone-ABA, in improving grapevine dormant season resilience in two economically important cultivars for the Finger Lakes wine region, ‘Riesling’ and ‘Cayuga White’ and their offspring, ‘Aravelle’, a newly released grapevine cultivar from Cornell. In 2022 and 2023, tetralone-ABA was applied at 0.5 g/vine after harvest. Within weeks after treatment application, chlorophyll content decreased significantly faster in tetralone-ABA treated grapevines, which resulted in earlier defoliation. The whole winter monitoring of bud cold hardiness indicates that tetralone-ABA promoted cold acclimation and slowed deacclimation, but the effects were different in different cultivars. Transcriptomic characterization of buds during deacclimation in controlled environment suggests that tetralone-ABA intensively impacted the pathways involved in growth restoration. Spring phenology records show that tetralone-ABA delayed budbreak for 7 to 11 days in different cultivars, and the delaying effect faded during the growing season and did not impact growing season physiology or yield. Our research highlights the potential of tetralone-ABA as a valuable tool in viticulture, offering a novel approach to enhancing the resilience of grapevines against climatic challenges.
The overall goal of the proposed project is to determine whether an ABA analog, tetralone-ABA, can be used as a budbreak delaying product in vineyards to effectively manage late spring frost without negative impacts in the dormant and growing seasons, and the underlying mechanism of its delaying effect through a systematic approach by long-term monitoring of grapevine physiology and RNA-seq. The specific objectives are:
- Monitor the impact of tetralone-ABA on dormant season physiology, including cold hardiness dynamics and the transition between endodormancy and ecodormancy.
- Identify the underlying mechanism of tetralone-ABA’s delaying effect on budbreak using comparative transcriptomics
- Monitor the impact of tetralone-ABA on grapevine phenology and physiology during the growing season
- Evaluate the impact of tetralone-ABA on yield component
The purpose of this project is to evaluate the feasibility of using an abscisic acid analog, tetralone ABA, as a budbreak delaying product in vineyards to mitigate spring frost damage. Cold-related damage is estimated to result in 5%-15% of crop loss worldwide, and this number is likely to increase due to more weather extremes induced by climate change. Apart from the direct impact of extreme cold on the survival of grapevine in winter, late spring frosts directly threaten the growth and productivity of grapevines, especially the fragile and most fruitful primary buds. For example, the 2007 Easter freeze led to 50-100% crop loss in vineyards in the Eastern and Midwestern U.S. when an unusually warm and early spring in March was followed by devastating freezing events in April. Climate change predictions suggest that while winter temperatures will become less severe on average, there is potential for increased frequency of temperature extremes and damaging spring frosts due to earlier budbreak. Major crop losses caused by spring frost have been reported with higher frequency in major grape and wine regions in the U.S. and the world. For the Northeastern U.S., the occurrence of spring frost damage may increase in severity due in part to a high adoption rate of hybrid varieties with Vitis riparia background in this region. These cultivars were selected for their supreme cold hardiness in midwinter; however, they also tend to initiate budbreak earlier in the season, increasing the risk of spring frost damage. With these factors combined, methods for the management of spring frost are urgently needed for the sustainability of the grape and wine industry in Northeastern U.S.
Research
Plant materials and treatment application
The experiment was conducted in 2022-2023 dormant season on Vitis vinifera ‘Riesling’, Vitis hybrid ‘Cayuga White’ and their offspring, ‘Aravelle’ at Finger Lakes Teaching and Demonstration Vineyard at Anthony Road Wine Co. at Geneva, NY, US (42.71° N, -76.97 ° W). ‘Cayuga White’, with Vitis labrusca and Vitis rupestris genetic background, sustains sufficient cold hardiness to survive in the winters in the cool climate viticultural regions in North America. This cultivar tends to deacclimate slower and intiate budbreak later as compared to other hybrid cultivars. ‘Riesling’ is a moderate cold hardiness cultivar of great economic importance for cold climate grape and wine industries such as Finger Lake regions. 'Riesling' exhibits mid-seaon budbreak as compared to other Vitis vinifera cultivars. Limited information is available for the overwintering behavior of ‘Aravelle’ as this cultivar was recently released.
The experimental vines were subjected to standard vineyards management practices in 2022 growing season. The treatments were applied as a foliar spray to the canopy a week after the harvest of 'Riesling' on 10/5/2022. The two treatments are tetralone-ABA treatment and control treatment. Tetralone-ABA ((±)-tetralone ABA, purity > 95%, ABAzyne Biosciences Inc., Saskatoon, Canada) was obtained as fine powder and was kept under dry, cool and shaded condition to maintain the chemical activity. Upon the use for field experimentation, stock solutions were prepared by dissolving every 6 g of tetralone-ABA in 1 L of distilled water with 120.0 mL of DMSO (Fisher Scientific, NH, US) and 3840.0 µL of 5.00 M NaOH solution (Sigma-Aldrich, MO, US). The stock solutions were transported to the experimental site and further diluted to the final concentration of 0.5 g·L-1 using distilled water. During treatment application, 1 L of 0.5 g·L-1 tetralone-ABA solution was applied on the foliar on each experimental vine, which corresponds to 0.5 g tetralone-ABA per vine. Control treatment was distilled water mixed with same concentrations of DMSO and NaOH. Silwet L-77 (Helena Agri-Enterprises, TN, US) at a rate of 0.05 % (v/v) was added as a surfactant to both treatments. For each cultivar, each treatment was applied on 12 vines.
Relative chlorophyll concentration measurement
After treatment application, relative chlorophyll concentration of the leaves in experimental vines were quantified using MC-100 chlorophyll concentration meter (Apogee Electronics, CA, USA). For each experimental vine, the measurement was conducted three times on three randomly selected leaves in the lower, middle and upper canopy. Relative chlorophyll concentration was expressed as SPAD (Soil Plant Analysis Development), a relative measure of chlorophyll concentration derived from the absorbance of specific wavelengths of light (Richardson et al., 2002; Parry et al., 2014; Yuan et al., 2016). The three readings were averaged as subsamples to represent the relative chlorophyll concentration of the vine. Relative chlorophyll concentration measurement was conducted four times until defoliation at one-day post-treatment (10/6/2022), one-week post-treatment (10/12/2022), two-week post-treatment (10/19/2022) and three-week post-treatment (10/26/2022).
Cold hardiness monitoring in dormant season
After treatment application, the monitoring of grapevine bud cold hardiness was conducted over the entire dormant season to evaluate the treatment effect of dormant season physiology. Grapevine bud cold hardiness was determined bi-weekly from 10/26/2022 to 3/22/2023 using differential thermal analysis based on standardized protocol (Mills et al., 2006; Londo et al., 2023). Briefly, grapevine buds were loaded in sample plates and treated with a decreasing of temperature with 4 °C·h-1 from 0 °C to -50 °C in a programmable freezer. Thermocouples on samples plates detect low temperature exotherms (LTEs), which represent the intracellular ice nucleation of grapevine buds, corresponding to the cold hardiness of the buds (Pierquet & Stushnoff, 1980; Mills et al., 2006). For each cultivar and each treatment, at least 15 buds were used to determine the concurrent cold hardiness.
Deacclimation assays
In addition to bud cold hardiness monitoring, deacclimation rate (the speed of cold hardiness loss that occurs under growth permissive condition) was periodically measured during the dormant season to monitor the progression of dormancy transition in response to different treatments. Deacclimation rate was only determined in ‘Riesling’ due to limited plant material in the other two cultivars. Buds from both cultivars were collected three times from field at 552 (11/7/2022), 1005 (1/2/2023) and 1352 (3/13/2023) chilling units (NC model, https://products.climate.ncsu.edu/ag/chill-models/) to be subjected to deacclimation assay. These three timepoints correspond to endodormancy (unable to deacclimate and budbreak under growth permissive condition), transition from endodormancy to ecodormancy (partially able to deacclimate and budbreak under growth permissive condition) and ecodormancy (fully able to deacclimate and budbreak under growth permissive condition), which represent the major physiological stages in dormant season (North & Kovaleski, 2023). During each deacclimation assay, canes collected from field were chopped into single bud cuttings, and the cuttings were incubated with cutting end dipped in water in growth chamber at 22 °C. At day 0, day 3, day 5, day 7 and day 14 in growth chamber, bud cold hardiness was determined by conducting DTA with at least 15 buds. Deacclimation rate, the coefficient of the linear regression between LTE and days in chamber, was generated during each deacclimation assay for each treatment to determine the ability of deacclimate (Kovaleski et al., 2018).
Phenology recording
Phenology recording was conducted before budbreak to evaluate treatment effect on growth restoration and vegetative growth during growing season. Given one of the major purpose of the study is to assess the impact of tetralone-ABA on the timing of budbreak, we combined two previously established grapevine phenology systems, EL system (Eichhorn & Lorenz, 1977) for the stages after budbreak and Modified Shaulis Field Score (https://lergp.com/modified-shaulis-field-score) for the stage before budbreak to increase the resolution of phenological stages in early growing season. Visual assessment of phenology was conducted on each experimental vine three times every week from 04/19/2023 to 06/26/2023. For each cultivar and each treatment, the date that at least 50% of experimental vines reached budbreak stage was recorded as the date of budbreak (Kovaleski & Londo, 2019; Wang et al., 2020; Wang & Dami, 2020).
Growing season physiology and harvest data
During the growing season, grapevine photosynthesis and respiration was measured to identify if the tetralone-ABA effect growing season physiology. A Ll-600 Porometer/Fluorometer (LI-COR Biosciences, NE, US) was used to quantify the photosynthesis efficiency, expressed as ETR (µmol·m-2·s-1), calculated from operating efficiency of PSII (ΦPSII) and ambient light condition (Qamb), and stomatal conductance, expressed as gsw (mol·m-2·s-1). Harvest data, including yield per vine and cluster per vine, were obtained during the harvest to evaluate tetralone-ABA’s impact on the overall productivity of the treated grapevines.
RNA-seq during deacclimation assays, library preparation and data processing
During each deacclimation assay and in parallel to bud cold hardiness measurement, bud samples were also collected for RNA-seq to investigate if tetralone-ABA induced divergence of transcriptome during deacclimation. RNA-seq was conducted in triplicate with five buds per replicate. Spectrum Plant Total RNA Kit (Sigma Aldrich, St Louis, MO) was used to extract total RNA from ground bud tissues. The Cornell University Institute of Genomic Diversity (Ithaca, NY, USA) provided technical support for library construction using Lexogen QuantSeq 3’mRNA-Seq Prep Kit (Lexogen, Greenland, NH). Sequencing of libraries as conducted at Cornell University Institute of Biotechnology (Ithaca, NY) using NextSeq500 (Illumina, Inc., San Diego, CA, USA) with 95 samples per lane. The read length was 85 bp, and sequencing was replicated three times in each library for technical validity. RNA-seq data was analyzed following previously generated pipeline specifically for grapevine tissues, including the downstream weighed gene co-expression network analysis (WGCNA) and over representation analysis (ORA) (Wang et al., 2022).
Cultivar-specific earlier defoliation, enhanced of cold acclimation and slower deacclimation
The impact of tetralone-ABA treatment on the relative chlorophyll concentration (SPAD) of three cultivars is shown in Figure 1. For ‘Riesling’, tetralone-ABA treated vines started showing significantly lower SPAD on one-day post-treatment (10/6/2022) (Figure 1). The treatment effect was also observed in the following three measurements on one-week post-treatment (10/12/2022), two-week post-treatment (10/19/2022) and three-week post-treatment (10/26/2022). Visually, tetralone-ABA treated ‘Riesling’ vines showed yellower pattern on one-week and two-week post-treatment and reached 100% defoliation on three-week post-treatment when control vines just started defoliation (Figure 1). In contrast, in ‘Cayuga White’, tetralone-ABA treatment effect was limited to a marginally lower SPAD at only one-week post-treatment (Figure 1). Defoliation occurred simultaneously in tetralone-ABA treated vines and control ‘Cayuga White’ vines. In ‘Aravelle’, the tetralone-ABA treatment effect was intermediate to its parents: significant reductions in SPAD were noted at one-week and two-week post-treatment, yet defoliation timings were consistent in tetralone-ABA treated and control vines (Figure 1).
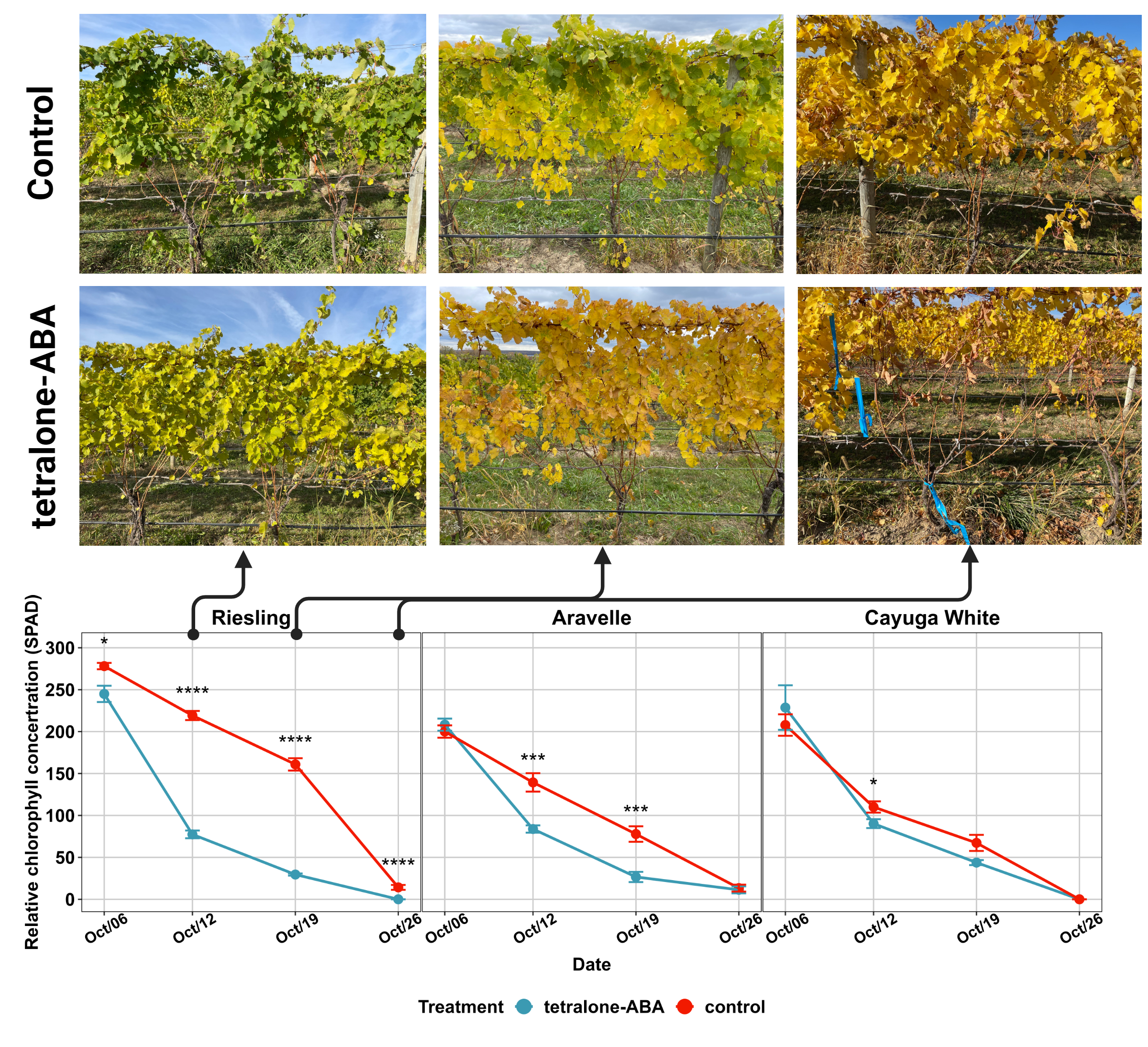
The impact of tetralone-ABA treatment on the dormant season bud cold hardiness of three cultivars is shown in Figure 2. In ‘Riesling’, following treatment application, significant enhancements in bud cold hardiness were consistently noted during the cold acclimation following decreasing minimum temperature from mid Oct. to mid Nov. (Figure 2). A sudden deacclimation was observed in control vines following an unusual warming event in early Jan., and tetralone-ABA treated vines exhibited superior bud cold hardiness in this period (Figure 2). Significant enhancements of bud cold hardiness were observed in tetralone-ABA treated vines after mid Feb., indicating tetralone-ABA’s delaying effect on deacclimation with increasing temperature approaching the end of dormant season (Figure 2). To compare, in ‘Cayuga White’, no treatment effect was identified during cold acclimation, but significant enhancements of bud cold hardiness were observed throughout the deacclimation process from until the end of data collection (Figure 2). Again, the tetralone-ABA treatment effect on ‘Aravelle’ was intermediate to its parents: enhancement of bud cold hardiness was observed in cold acclimation and deacclimation, but the degree of enhancement was comparatively modest (Figure 2).
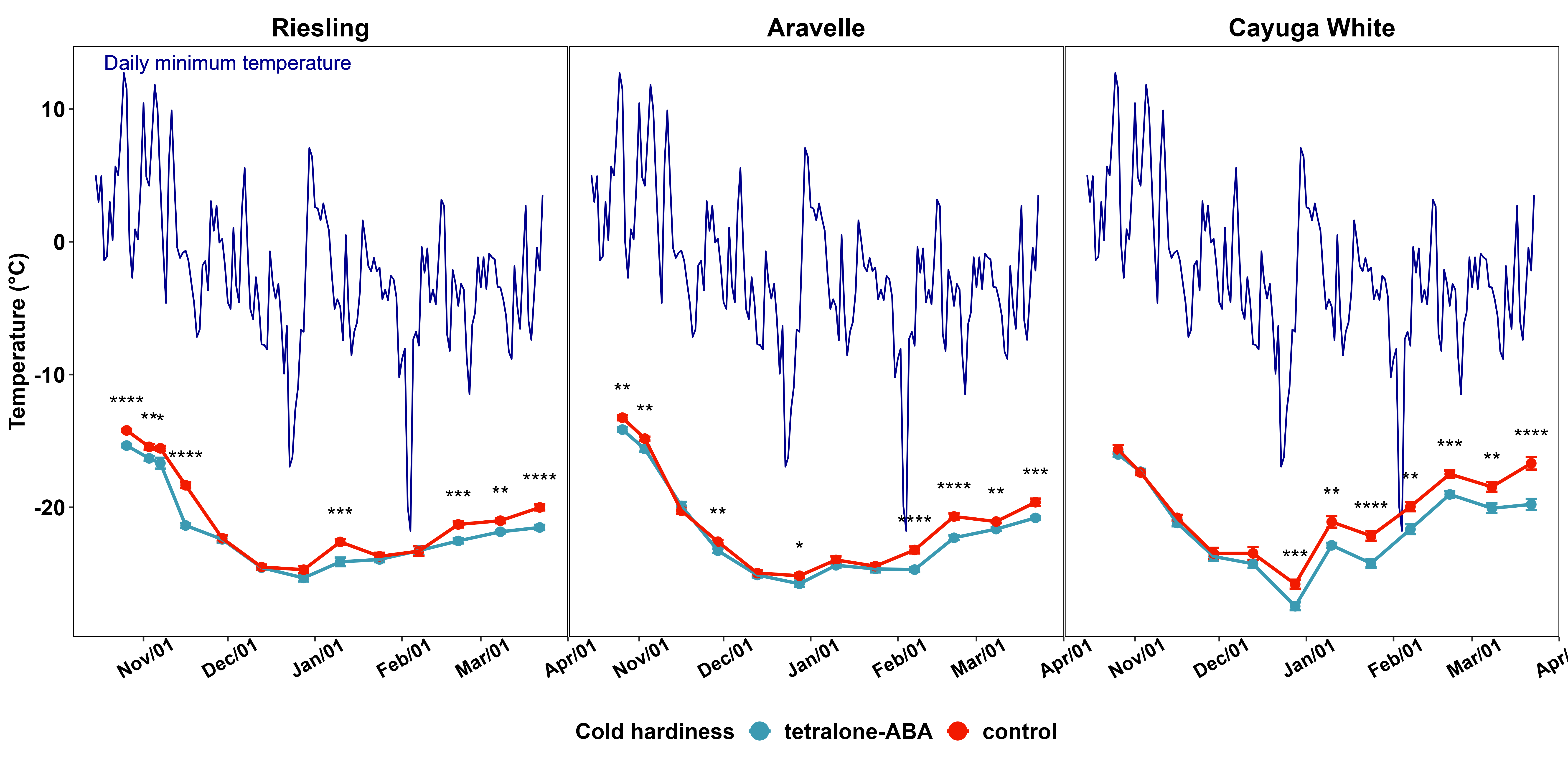
In the three deacclimation assays conducted in growth chamber using the ‘Riesling’ buds collected from field with 552 (11/7/2022), 1005 (1/2/2023) and 1352 (3/13/2023) chilling units, tetralone-ABA showed different effects on bud deacclimation with increasing field chilling (Figure 3A). In the first deacclimation assay, tetralone-ABA enhanced bud cold hardiness on day 0 and day 5 but did not alter the deacclimation rate (Figure 3A). In the second deacclimation assay, tetralone-ABA enhanced bud cold hardiness on day 7 and day 14 and decreased the deacclimation rate by 24.7% compared to the control (Figure 3A). In the third deacclimation assay, tetralone-ABA enhanced bud cold hardiness on day 0, day 3, day 5 and day 7 (Figure 3A). The measurement of bud cold hardiness on day 14 was excluded from the deacclimation rate calculation due to its proximity to budbreak, a period when bud cold hardiness typically plateaus. Compared to the control, tetralone-ABA treatment led to a 22.3% decrease in the deacclimation rate calculated from the bud LTE on day 0, day 3, day 5 and day 7 (Figure 3A).
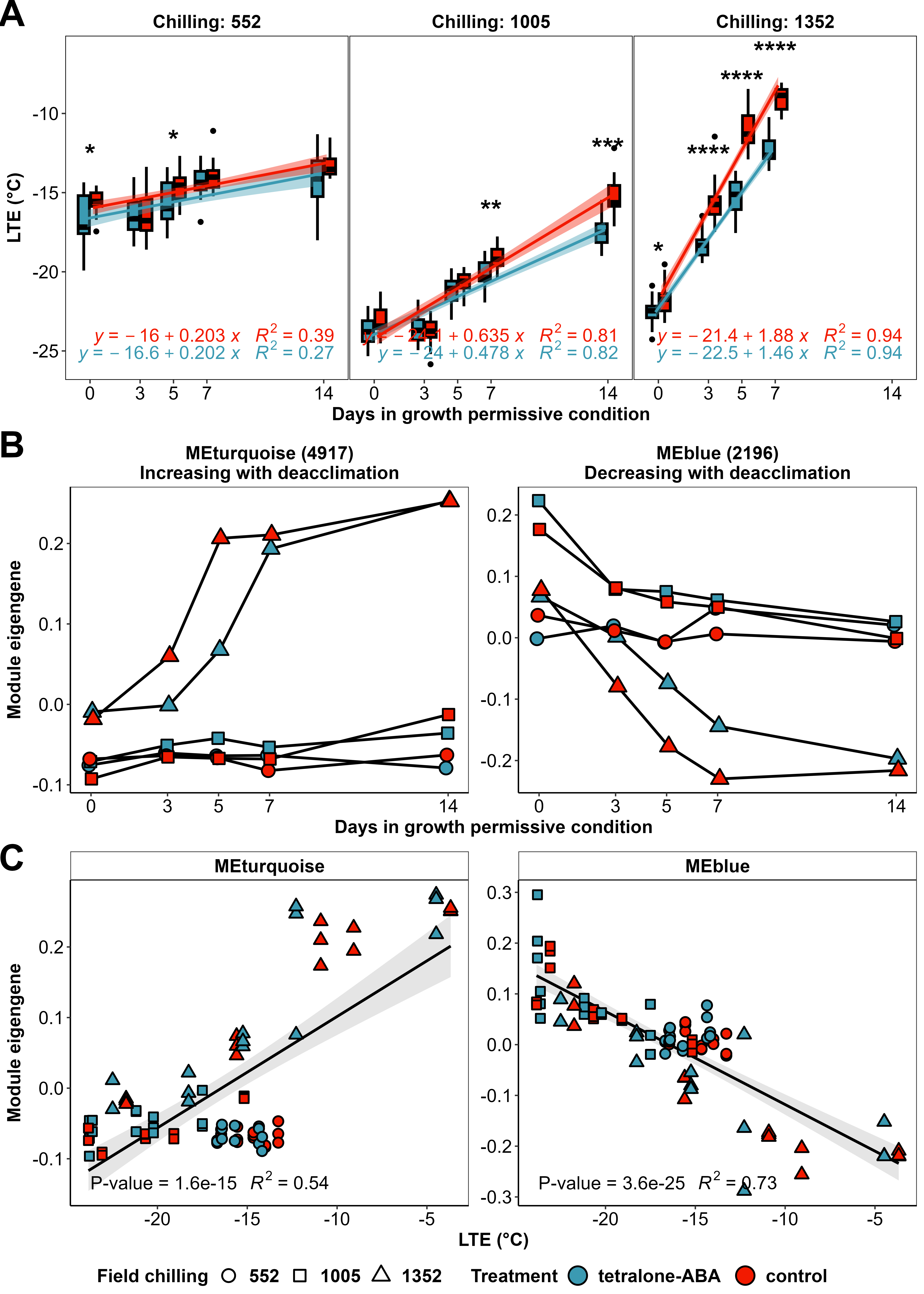
Transcriptomic analysis reveals two major gene clusters in response to deacclimation and tetralone-ABA treatment
In parallel to the bud cold hardiness data collection during each deacclimation assay, the buds were also examined for their transcriptomic response to tetralone-ABA during deacclimation. A total of 90 libraries were sequenced in three deacclimation assays. Each library successfully met the FastQC per base quality standards, demonstrating high-quality raw reads. The average length of these raw reads was 85 bp. After trimming to eliminate adaptor barcodes, the average read length was reduced to 65 bp. On average, each library contained 4.6 million reads, with a unique mapping rate averaging 78.9%. We applied Principal Component Analysis (PCA) and Uniform Manifold Approximation and Projection (UMAP) for the overall transcriptome characterization of the library, and six libraries were identified as outliers and were removed for any downstream analysis. After filtering out low count genes (the genes with averagely less than five transcripts per library), the final gene count matrix for differential expression analysis was composed of 84 libraries and 15,002 genes. PCA and UMAP of the final gene expression matrix demonstrate notable clustering of the libraries by field chilling unit.
As the next step, we implemented WGCNA on DESeq2-normalized gene expression matrix and identified 16 gene co-expression modules, excluding module 'grey' which contains the genes with a random signature with respect to treatments (noisy genes). The number of genes in each module ranged from 57 in module ‘lightcyan’ to 4917 in module ‘turquoise’. The module eigengenes (MEs), which represent the first principal component (PC1) of all genes in a module. Notably, the MEs of the two largest modules, ‘turquoise’ and ‘blue’ displayed divergent behavior with deacclimation: ME ‘turquoise’ generally increases when bud LTE increases, and ME ‘blue’ generally decreases when bud LTE decreases (Figure 3B). Further analysis revealed that ME ‘turquoise’ was positively correlated with bud LTE and ME ‘blue’ was negatively correlated with bud LTE (Figure 3C). More importantly, MEs ‘turquoise’ and ‘blue’ also shed light on the effects of tetralone-ABA treatment: significant differences in ME values were observed between tetralone-ABA treatment and control, especially on day 3, day 5 and day 7 in the third deacclimation assay when the buds were rapidly deacclimating. These results suggest the presence of two gene clusters whose expression is closely linked to deacclimation and is influenced by tetralone-ABA treatment.
Identification of the genes that differentially expressed in deacclimation and in response to tetralone-ABA treatment
Consequently, we applied a differential expression analysis with DESeq2 using bud cold hardiness as the only factor in the gene expression model. As bud cold hardiness aligns with deacclimation and reveals a tetralone-ABA effect (Figure 3A), the genes whose expression is highly correlated with bud cold hardiness are most likely the genes that respond to deacclimation and tetralone-ABA. By employing a padj (False Discovery Rate) threshold ≤ 1e-15, we identified 1314 highly correlated with deacclimation. Among these genes, 908 genes showed increasing expression in deacclimation, and 406 gene showed decreasing expression. ORA of the three lists of genes (all genes, increase in deacclimation and decrease in deacclimation) indicates that 22 pathways in VitisNet were significantly enriched (Figure 4A). In the ‘increase in deacclimation’ gene list, enriched pathways include several pathways in genetic information processing networks, cellular processes, transport and metabolism (Figure 4A). These pathways, such as the ribosome pathway that includes genes encoding for ribosomal proteins and the photosynthesis pathway that includes the genes encoding for photosystem I and II, are the fundamental pathways for plant growth.
Differences in expression between tetralone-ABA treated and control of the genes within the enriched pathways in ‘increase in deacclimation’ and ‘decrease with deacclimation’ are presented in the heatmaps of Figures 4B and C. For the genes with increasing expression in deacclimation, limited significant expression differences were observed between treatments in the first and second deacclimation assays (Figure 4B). However, in the third deacclimation assay, significantly lower expression was identified in tetralone-ABA treatment on day 3 and day 5 in almost every gene in every enriched pathway (Figure 4B). Similarly, the genes with decreasing expression in deacclimation did not show consistent differential expression in the first and second deacclimation assays but showed consistent higher expression on day 3, day 5 and day 7 in the tetralone-ABA treatment in the third deacclimation assay (Figure 4C).

Delayed budbreak but unchanged phenology after shoot elongating
Phenology recording was initiated at the end of dormant season on 04/19/2023. For ‘Riesling’, the phenological window from ‘first swell of bud (Modified Shaulis Field Score 2)’ until ‘shoot elongating (EL-15)’ was delayed (Figure 5). The dates of budbreak, defined as the date when more than 50% of experimental vines reached the budbreak stage, (Modified Shaulis Field Score 4) in control vines and tetralone-ABA treated vines were 04/24/2023 and 05/05/2023, respectively (Figure 5). For ‘Aravelle’, the phenological window from ‘first swell of bud’ until ‘inflorescence clear (EL-12)’ was delayed (Figure 5). The dates of budbreak in control vines and tetralone-ABA treated vines were 04/24/2023 and 05/03/2023, respectively (Figure 5). For ‘Cayuga White’, the phenological window from ‘full swell of bud (Modified Shaulis Field Score 3)’ until ‘inflorescence clear (EL-12)’ was delayed (Figure 5). The dates of budbreak in control vines and tetralone-ABA treated vines were 05/01/2023 and 05/09/2023, respectively (Figure 5). In summary, tetralone-ABA resulted in a delay of budbreak for ‘Riesling’, ‘Aravelle’ and ‘Cayuga White’ by 11 day, 9 day and 8 day, respectively.
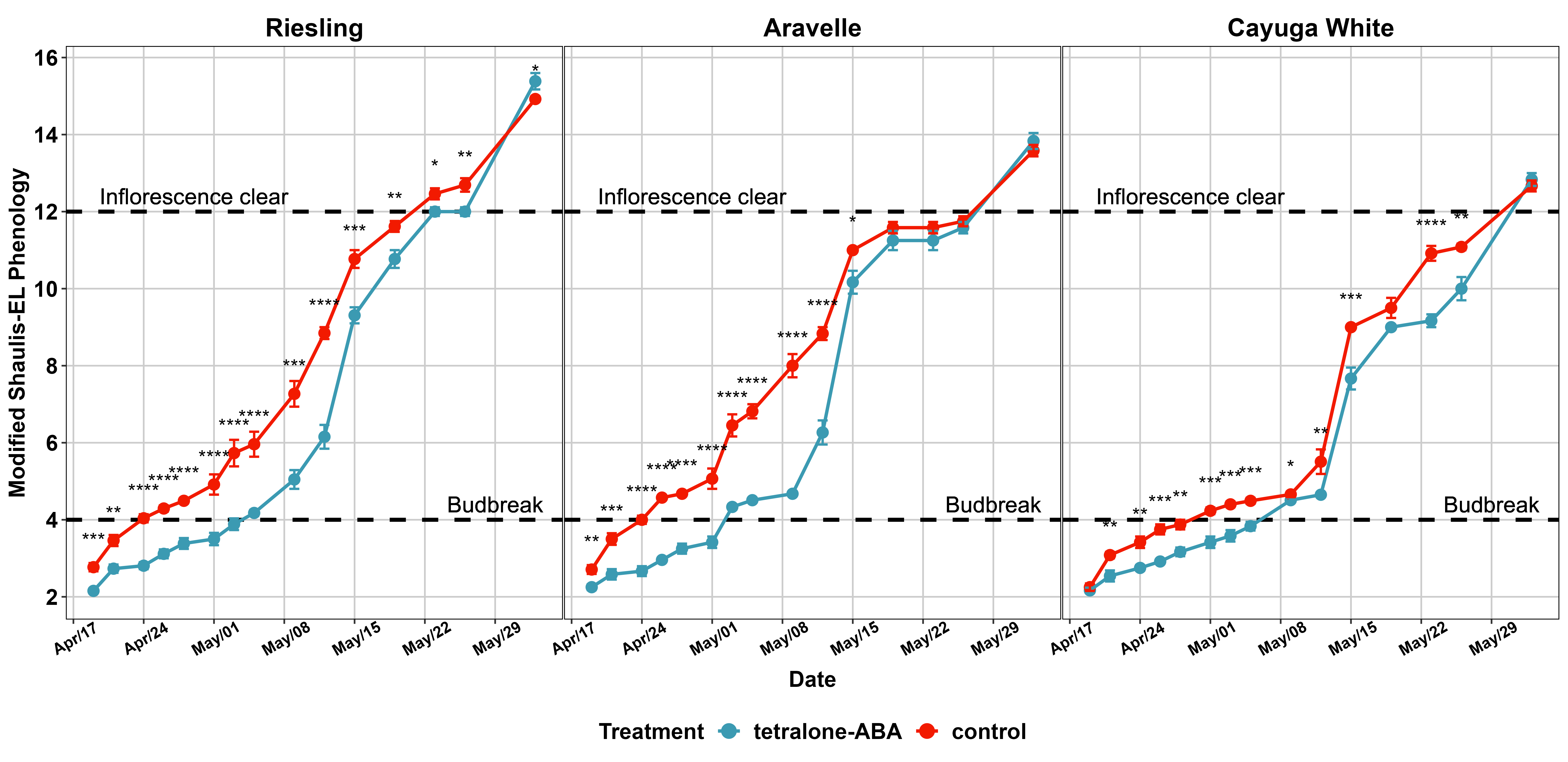
Unchanged growing season physiology and yield
During the growing season, we conducted two measurements of grapevine canopy physiology on 6/28/2023 and 7/11/23 which involves the relative quantification of photosynthesis efficiency and stomatal conductance. In both measurements, the photosynthesis efficiency and stomatal conductance did not show a sidnificant difference between the two treatments for any of the three cultivars (Figure 6A and B).
The experimental vines under tetralone-ABA treatment and control treatment were harvested on 10/10/2023 for ‘Riesling’ and 9/26/2023 for ‘Aravelle’, and harvest data, including cluster per vine and yield per vine were collected to evaluate the treatment effect. In ‘Riesling’, the cluster per vine ranged from 37 to 74 and did not show significant difference between the two treatments (Figure 6C). The yield per vine ranged from 2.7 kg to 5.3 kg and did not show significant difference between the two treatments (Figure 6D). In ‘Aravelle’, the cluster per vine ranged from 70 to 150 and did not show significant difference between the two treatments (Figure 6C). The yield per vine ranged from 3.6 kg to 12.8 kg and did not show significant difference between the two treatments (Figure 6D).
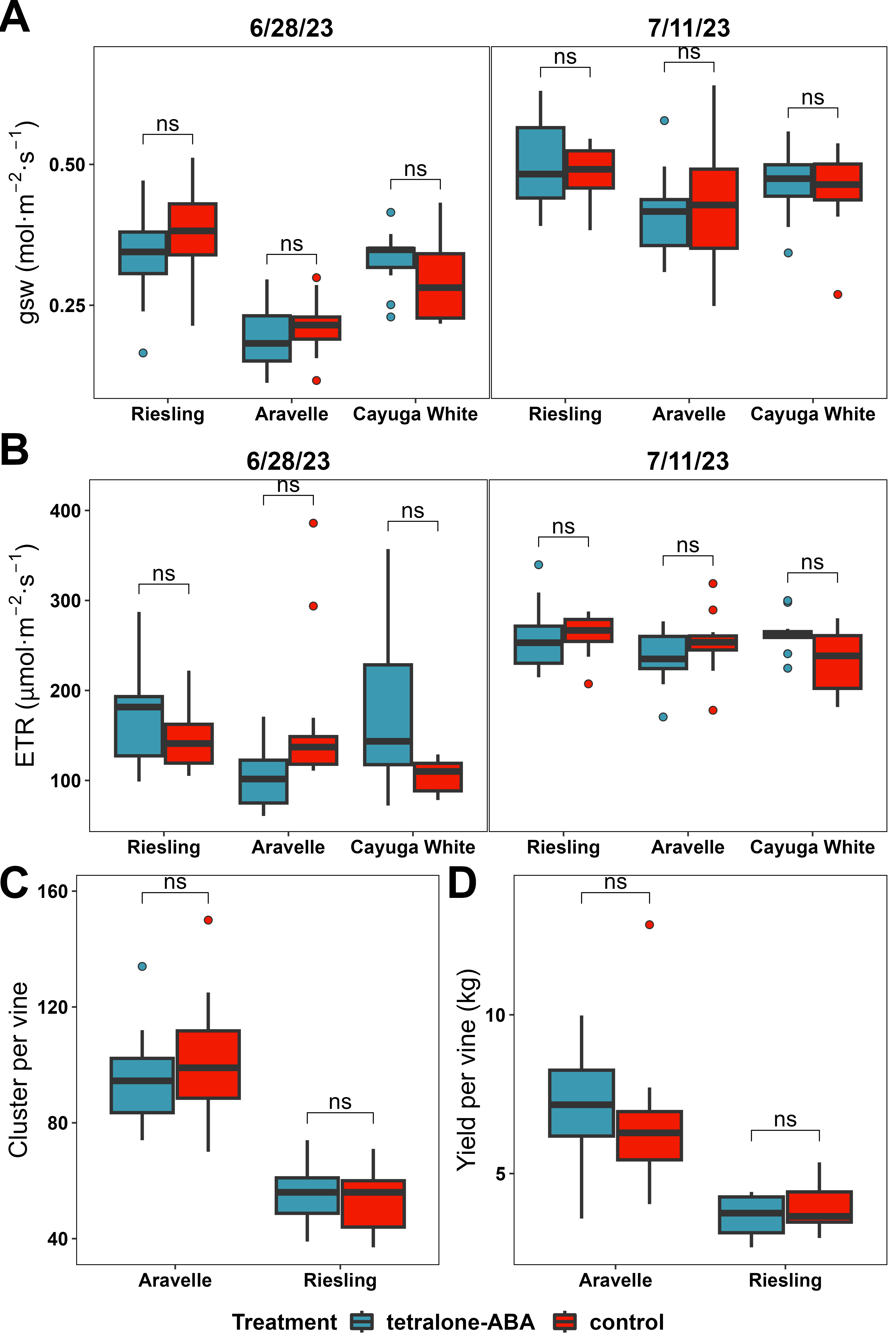
In the whole-year evaluation of the impact of post-harvest foliar application of tetralone-ABA on grapevine, we observed that tetralone-ABA induced:
- Faster leaf chlorophyll degradation
- Earlier defoliation
- Enhanced cold acclimation
- Slower deacclimation
- Delayed budbreak without affecting growing season physiology and yield
In addition, transciptomic analysis of buds during deacclimation indicates that the slower deacclimation in tetralone-ABA treated buds in field and controlled environment conditions observed in this study is likely a consequence of tetralone-ABA’s delaying effect on the upregulation or downregulation of key regulatory and functional pathways during deacclimation. We also observed cultivar-specific response to tetralone-ABA treatment in chlorophyll degradation, defoliation and bud cold hardiness in dormant season. The intermediate response in ‘Aravelle’, compared to its parents ‘Riesling’ and ‘Cayuga White’, might indicate the existence of a genetic variation controlling the sensitivity to tetralone-ABA.
Education & Outreach Activities and Participation Summary
Participation Summary:
In spring 2023, we worked together with Cornell viticulture extension specialists through Cornell Recent Advances in Viticulture and Enology (CRAVE) and Business Enology Viticulture NY (B.E.V. NY) to introduce tetralone-ABA as a budbreak delaying practice and translate the result generated from this project to the growers in NY. Hongrui Wang (graduate student PI) and Jason Londo (faculty PI) organized a workshop, ‘Measuring and Managing Cold Hardiness in Grapevines’, in B.E.V. NY 2023 with the help of Hans Walter-Peterson. During the workshop, the preliminary findings of the tetralone-ABA project were presented, and the attendees’ feedback was collected using PollEverywhere.
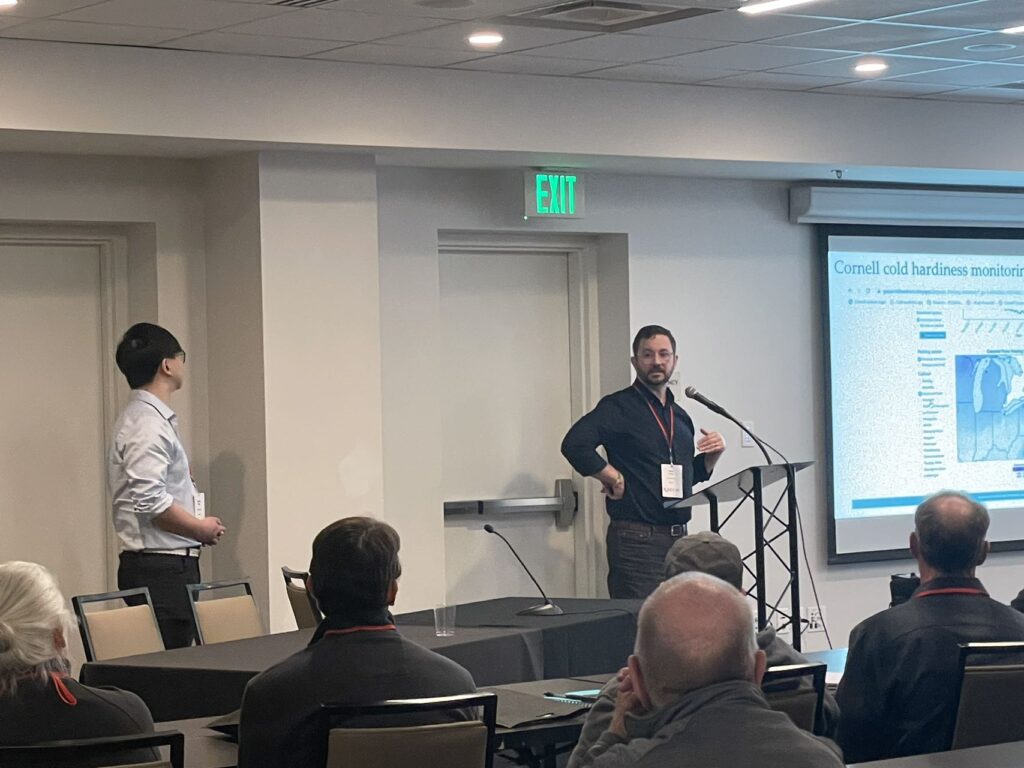
In addition, the preliminary result from the project was presented by Hongrui Wang (graduate student PI) in the Graduate Review Day of Cornell School of Integrative Plant Science Horticulture Section in spring 2024.
Project Outcomes
Our research demonstrates that the application of tetralone-ABA can effectively enhance cold hardiness and delay budbreak in grapevines, thereby offering a viable strategy to mitigate the risks associated with extreme cold events in winter and late spring frosts. This outcome brings considerable economic benefits for farmers, particularly in regions prone to such climatic extremes, by potentially reducing crop losses and enhancing the viability of grapevine cultivation in cold/cool climate viticultural regions in Northeastern U.S. Environmentally, the use of this ABA analog represents a sustainable and less invasive approach compared to traditional frost mitigation techniques, which often involve energy-intensive methods like heaters or wind machines. From a social perspective, the adoption of tetralone-ABA can contribute to the stability and resilience of rural communities dependent on viticulture, which ensures the livelihoods of small-scale farmers and contributes to the economic health of these regions. Additionally, our research into the transcriptomic changes induced by tetralone-ABA provides a foundational understanding that could guide future product development and breeding programs aimed at improving grapevine resilience. The knowledge and methodologies developed through this project promise to have far-reaching impacts, contributing to the overall sustainability of the grape and wine industry.
During this project, significant advancements were made in our understanding of grapevine dormant season physiology, particularly in the context of viticulture under climate change. Both my advisor and I developed a deeper appreciation and skill set for integrating cutting-edge transcriptomic analysis with traditional vineyard management practices. This project shed light on the responses of different grapevine cultivars to the tetralone-ABA treatment, enhancing our understanding of grapevine dormant season physiology, dormancy dynamics, and the underlying molecular mechanisms for grapevine cold hardiness. Our results might partially indicate that tetralone-ABA treatment might be a safer sprayable product that effectively induces enhanced cold hardiness and delays budbreak in grapevines without negatively impacting growing season physiology and yield. I am now more passionate about exploring sustainable, bio-informed strategies to enhance crop resilience against environmental stresses, especially in cold/cool-climate viticulture. The successful integration of molecular biology techniques with field-based research in this project paves the way for innovative approaches in sustainable agriculture, potentially influencing future practices in crop management and breeding for climate resilience.