Final report for GS18-190
Project Information
Owing to its warm climate, Florida has a unique, early-season blueberry market valued over $84 million. Spotted wing drosophila (Drosophila suzukii Matsumura; "SWD"), a global, invasive pest of small, soft-skinned fruits, has become a significant pest of Florida's blueberries over the last decade by rendering ripe fruit unmarketable. Currently, heavy pesticide use is the only feasible and effective control for this pest. The high cost of pesticides and the development of resistance threaten to collapse Florida's niche blueberry market, and excessive pesticide use endangers farm workers and non-target organisms like pollinators, beneficial insects, fish and wildlife.
This project's aim was two-fold: to thoroughly map seasonal timing and patterns of SWD migration from woodlands into blueberry fields; and to evaluate the efficacy of HOOK SWD, a new attract-and-kill product containing an SWD-specific fruit volatile-based attractant and a low-risk, naturally derived insecticide to control SWD populations. Understanding spatio-temporal patterns of SWD migration and utilization of attract-and-kill technology can facilitate more targeted insecticide applications, reducing the amount of insecticide used, reducing costs for farmers, limiting insecticide exposure, and improving environmental quality.
Objective 1: Monitor season-long adult SWD population density and map seasonal patterns of movement along a woodland-to-cropland gradient on two Florida blueberry farms (one conventional, one certified organic).
Objective 2: Compare adult SWD mortality and larval infestation using choice and no-choice cage experiments between HOOK-treated and untreated blueberry plants.
Objective 3: Compare adult and larval SWD population density in HOOK-treated fields to untreated fields on two Florida blueberry farms (one conventional, one certified organic).
Research
Objective 1 (seasonal monitoring)
Materials and Methods
Three Scentry traps containing SWD lures (Scentry Biologicals, Inc. Billings, Montana) were placed along each of three 25 m transects beginning at the woods edge and extending into blueberry fields at commercial blueberry farms. The distances of the traps from the woods represented our treatments: 0m (woods edge), 20m (field edge), and 40m (mid-field). This plot design was replicated four times in individual fields at a conventionally managed site and replicated twice at an organically managed site in 2018 and 2019. Trap catch samples were collected and drowning solution replaced weekly. Lures were replaced monthly. Samples were transported to the University of Florida Small Fruit and Vegetable IPM (UF SFVIPM) Laboratory, immediately refrigerated, and processed during the week of collection. The number of male and female SWD, other drosophilids, other common blueberry pests, and natural enemies were counted in each sample. Collection began in early February and continued until mid-May in 2018 and 2019.
In 2019, 10 ripe blueberries were collected from bushes around each 20 m and 40 m trap at the conventional and organic sites on weeks 8 (26 March), 12 (23 April), and 15 (15 May) of the monitoring period. Blueberry samples were incubated for three weeks, and any emerged adults were counted and identified to sex.
Statistical Analysis
To facilitate interpretation, weeks were combined into ranges before analysis. The effects of treatment, week, and the treatment-by-week interaction on adult trap catch and adult emergence were analyzed using a multivariate analysis of variance (ANOVA) with repeated measures. Due to low variability within 2019 organic emergence data, the random effect (repeated measures analysis) was removed from the model before ANOVA analysis. If significance was observed (α = 0.05), means were separated using Tukey’s Honest Significant Difference (HSD) test. Separate analyses were performed for conventional and organic sites. All trap catch and emergence data were square-root transformed to achieve normality. All analyses were performed using R (statistical program).
Misidentification of male SWD captured in trap samples occurred in weeks 1-6 in 2019. Only female trap catch was analyzed for these weeks. Analysis of total adult trap catch and male trap catch data from both sites were performed for weeks 7-13.
Objective 2 (HOOK evaluation cage study)
Materials and Methods
No-choice experiments. 50 SWD (1:1 ratio of F:M; 2-8 days old) were released in 4 x 2 x ft. mesh cages. Each cage contained two two-year-old blueberry plants with one plant at each longitudinal end of the cage. Five mesh satchels, each containing five store-bought, organic blueberries were randomly hung from each plant. Each blueberry plant contained either one HOOK-treated plastic hangtag per plant (HOOK treatment) or no hangtag (control). Two grams of HOOK were applied to each hangtag. Each cage contained one 500 mL plastic Tupperware container of water with cotton wicks as a water source. A white sheet covered each cage base and white paper discs covered the soil surface of each plant to facilitate observation of dead flies. Every 12 hours after the initial introduction of flies, dead flies were removed by hand and counted. After 72 hours, berry satchels were removed from plants, and berries were incubated for three weeks to compare adult emergence between treatment and control cages. HOOK treatment and control plots were each replicated five times.
Choice experiments. All aspects of experimental design and protocol were the same as described for no-choice experiments, but each cage contained one HOOK-treated plant on one longitudinal end of the cage and one control plant on the other end. Choice treatment cages were replicated five times.
Statistical Analysis
The effect of cage treatment (no choice with HOOK hangtags, no choice with no HOOK (control), and choice with one HOOK hangtag on one plant and no HOOK hangtag (control) on the other plant) on adult survival was analyzed using a Cox Proportional-Hazards Regression model. The effects of cage treatment and plant treatment (HOOK, no HOOK (control)) on adult emergence were analyzed using a two-way ANOVA. If significance was observed (α = 0.05), means were separated using Tukey’s HSD test.
Objective 3 (HOOK evaluation field studies)
Materials and Methods
During the 2018 field season, the field study took place at a conventionally managed commercial blueberry farm in Waldo, FL. During the 2019 season, the study was repeated at the same conventional farm and a USDA Certified Organic commercial blueberry farm in Hawthorne, FL. HOOK was applied at a rate of 1.5 L/acre in two of the three treatments at each site. Treatments included: a) HOOK applied once every 7 days in addition to the grower’s standard (suite of insecticides and cultural practices) (HOOK-7d), b) HOOK applied once every 14 days in addition to the grower’s standard (HOOK-14d), and c) no HOOK applied (grower’s standard only; control). Plots were approximately 2.7 hectares (6.5-6.68 ac.) with three replicates per treatment in 2018 and four replicates per treatment in 2019. HOOK was applied to the bases or lower canopies of bushes using hand-pump backpack sprayers. In 2018, the first application at the conventional site was made on 13 April, and the final application of all treatments was made on 11 May. In 2019, the first application at the conventional site was made on 12 April, and the final application was made on 10 May. In 2019, the first application at the organic site was made on 11 April, and the final application was made on 19 May.
Adult SWD density was evaluated using Scentry traps and lures (Scentry Biologicals, Inc., Billings, MT). Lures were replaced on the third week of each trial. Tap water with several drops of unscented dish soap was used as a drowning solution in each trap. One trap was deployed randomly in each treatment replicate. The contents from each trap were emptied into a collection jar, labeled, and stored in a cooler each week before transporting to the UF SFVIPM Laboratory for processing. The drowning solution was replaced weekly, and SWD per trap sample were counted by sex under a dissecting microscope.
Blueberries (1.8 L) were harvested on weeks three and six of the studies at the conventional site (27 April and 18 May 2018; 29 April 2019) from bushes around each trap to measure adult emergence. Very few berries were present in the field at the conventional site on week 6 in 2019 (15 May 2019), so only 100 berries were collected around each trap. At the organic site, 400 ripe blueberries were randomly collected weekly from each plot. All blueberry samples were stored in plastic containers and incubated in an environmental chamber for three weeks at 24°C, 64% RH, 16:8 L:D cycle. The number of SWD adults that emerged from each sample were counted by sex under a dissecting microscope.
Statistical Analysis
Data were separated by year, and the effects of treatment (HOOK-7d, HOOK-14d, and the grower’s standard (control)), week, and the treatment-by-week interaction on adult trap catch and adult emergence were analyzed using a multivariate ANOVA with repeated measures. Square root transformations were applied to normalize adult trap catch and emergence data. If significance was observed (α = 0.05), means were separated using Tukey’s HSD test. All analyses were performed using R. No emergence was observed from blueberries collected on week 3 (25 Apr 2019) at the organic site due to a growth chamber malfunction. These data were removed from the dataset before analysis.
Objective 1 (seasonal monitoring)
Results
Conventional. Total SWD adult trap catch (males and females, combined) was different among treatments in 2018 (F4,95 = 15.00; P < .0001; Table 1). The greatest captures were observed in the 0 m treatment (traps deployed at woods edge), which were greater than captures at the 20 m treatment (traps deployed on the field edge; P = 0.02). SWD captures in traps placed at 40 m (within-field) were not different than traps placed at 0 m and 20 m. Trap catch was greater in weeks 13-14 than in all other weeks except weeks 1-3 (F2,35 = 3.81; P = 0.03). A treatment-by-week interaction was observed (F8,95 = 2.66; P = 0.01). Female trap catch was the same among treatments (F2,33 = 3.36; P = 0.05) but was different among weeks. The greatest number of females were captured in weeks 1-3 compared with all other weeks (F4,95 = 19.79; P < .0001). A significant treatment-by-week interaction was observed (F8,95 = 2.78; P = 0.008). Male trap catch was greater at the woods edge (0 m) than in traps placed at 20 and 40 m within fields (F2,35 = 4.54; P = 0.02). The greatest number of male SWD were trapped in weeks 13-15 (F4,95 = 13.61; P < .0001). The treatment-by-week interaction was not significant.
Total SWD trap catch in 2019 was greater at 0 m traps than in 20 m and 40 m traps (F2,33 = 21.31; P < .0001) and was greatest in weeks 11-13 (F2,66 = 13.61; P < .0001; Table 2). A treatment-by-week interaction was observed (F4,66= 6.04; P < .0001). Female trap catch was not significantly different among treatments across the entirety of the study but was greater in weeks 11-13 than in all other weeks (F5,165 = 79.16; P < .0001; Figure 1). A treatment-by-week interaction was observed (F10,165 = 4.63; P < .0001). Male captures were significantly greater in 0 m traps than in 20 m and 40 m traps (F2,33 = 39.90; P < .0001) and were greatest in weeks 11-13 (F2,66 = 295.85; P < .0001). The treatment-by-week interaction was significant (F4,66 = 8.49; P < .0001).
Total SWD adult emergence from blueberries collected in 2019 was not different among treatments but was significantly greater in the final collection date (15 May) compared with the two earlier collection dates (26 March and 23 April; F2,18 = 28.20; P < .0001; Figure 2). A significant treatment-by-week interaction was observed, with greater emergence from blueberries collected at 40 m than 20 m on the last collection date (F2,18 = 4.66; P = 0.02). Female emergence was similar to total emergence when analyzed separately (week: F2,17.99 = 35.98; P < .0001; treatment*week: F2,17.99 = 8.19; P = 0.003). Consistent with total and female emergence, male emergence was greatest on the final collection date (F2,18 = 17.84; P < .0001), but no treatment-by-week interaction was observed.
Organic. Total SWD adult trap catch was significantly greater in 20 m traps than in 0 m traps (F2,15 = 4.20; P = 0.04; Table 3) in 2018. Neither treatment was different than the 40 m treatment. Captures in weeks 12-13 and 14-15 were greater than those in weeks 8-9 (F3,45 =15.67; P < .0001). The treatment-by-week interaction was not significant. Similar differences among treatments were observed when female trap catch was analyzed individually (F2,15= 4.17; P = 0.04). Captures in week 12-13 were greater than those in all other weeks except 10-11 (F2,45 = 13.68; P < .0001). No treatment-by-week interaction was observed. Trends in male trap catch were similar to those observed for total trap catch (treatment: F2,15 = 4.51; P = 0.03; week: F3,45 = 15.02; P < .0001).
In 2019, total SWD trap catch was not significantly different among treatments but was greatest in weeks 12-13 (F3,45 = 83.89; P < .0001; Table 4). A significant treatment-by-week interaction was observed (F2,15 = 9.51; P = 0.002). Similar trends existed when female (week: F4,60 = 54.59; P < .0001; treatment*week: F8,60 = 6.23; P < .0001) and male (week:F3,45 = 54.95; P < .0001; treatment*week: F6,45 = 3.33; P = 0.009) trap catch were analyzed individually. More males than females were captured across all weeks (t = 3.11; df = 71; P = 0.003; Figure 3).
Unlike adult emergence data from the conventional site, the greatest total emergence from blueberries collected at the organic site in 2019 occurred on the second collection date (F2,6 = 14.95; P = 0.005; Figure 4). Emergence was not different among treatments, and no treatment-by-week interaction was observed. Similar trends were observed when female (week: F2,6 = 8.27; P = 0.02) and male (F = 5.02e31; df = 2; P < .0001) emergence were analyzed independently.
Discussion
Trap catch and emergence data provide evidence that SWD migrate into blueberry fields from surrounding areas during the growing season. As the 2018 and 2019 blueberry seasons progressed at the conventional site, captures of SWD increased in within-field traps (20 m and 40 m) relative to traps placed at the woods edge (0 m) (Figures 5 and 6). This movement into cultivated fields is illustrated by treatment-by-week interactions. Female trap catch was highest in 0 m traps early in both seasons but became more evenly distributed in later weeks, suggesting movement of females away from the woods and into blueberry fields. Overall, females were trapped equally among treatments at the conventional site in 2018 and 2019, while male trap catch was highest in 0 m traps in both years. The tendency of males to remain in woodlands during berry growing seasons is likely due to differential resource requirements and resource-seeking behavior. Females are more likely to disperse within crop fields in search of suitable oviposition sites, while males may stay in woodlands where food is more abundant (Swoboda-Bhattarai 2017).
Adult emergence in 2019 supports the results observed from the trap catch data. A treatment-by-week interaction shows increased emergence from blueberries collected 40 m from the woods relative to other treatments in later weeks. This is the result of SWD migration into croplands and increased oviposition therein.
No patterns of seasonal migration were detected in 2018 at the organic site. It’s possible that minimal migration did occur in 2018, but measurement at a finer scale may have been required to tease out patterns of movement (Pelton et al. 2016; Figure 7). At the organic site, a treatment-by-week interaction showing seasonal migration into croplands was observed in 2019 (Figure 8). Similar to trends observed at the conventional site, total captures increased in 40 m traps relative to other treatments as the season progressed at the organic site, reflecting movement into fields and population growth.
Despite clear patterns of migration, trap catch data also provides evidence that adult SWD aggregate at woods edges (0m) throughout the blueberry growing season. Total SWD was only greater at 20 m traps at the organic site in 2018. Swoboda-Bhattarai (2017) and Evans et al. (2017) both observed aggregation of SWD along the edges of woods adjacent to cultivated berry crops, likely because food resources are more abundant and temperature and humidity are more favorable than among crops. The adjacent wooded area where 0 m traps were placed contains several known non-crop hosts of SWD, including wild blackberry (Rubus spp.), black elderberry (Sambucus nigra), wild grape (Vitis spp.), and pokeweed (Phytolacca americana) (Elsensohn & Loeb 2018; Lee et al. 2015).
Several factors may have restricted SWD to the wooded area despite the presence of ripe blueberries in cultivated fields. Higher ambient temperatures, more direct sunlight, and lower humidity in the open, mowed areas separating the cultivated fields from adjacent wooded areas may have discouraged some SWD from migrating into crops (Evans et al. 2017). Also, non-crop hosts in the surrounding woods may have set fruit during the blueberry growing season, deterring movement into crops (Pelton et al. 2016). While movement into fields did occur in our studies, significant population growth likely also occurred in the surrounding woods. Lower within-field trap catch may also have been the result of frequent insecticide treatments within cultivated blueberries in all studies.
There was no difference in trap catch or emergence among treatments at the organic site in 2019, and aggregation at the woods edge was not observed. Although weed coverage was not tested in these studies, weeds were much more abundant among cultivated blueberries at the organic site than at the conventional site. Greater weed abundance and increased structural complexity within the habitat may have generated more favorable microclimates, facilitating SWD movement into the blueberry field (Diepenbrock & Burrack 2017).
In all studies, total SWD trap catch was highest in the later weeks of the season, reflecting seasonal population growth at both sites. Emergence was also greatest on the last collection date (week 15) at the conventional site in 2019. Increasing SWD populations throughout berry growing seasons have been documented in multiple studies (Swoboda-Bhattarai 2017; Klick et al. 2016; Pelton et al. 2016).
Our findings complement other studies of seasonal SWD migration and small-scale population dynamics. Our studies are the first to demonstrate seasonal migration into cultivated fields within Florida commercial blueberry systems. These data illustrate when SWD populations are highest and when migration is likely to occur under normal growing conditions in north Florida. This information can be used to recommend optimal timing of SWD control activities to Florida blueberry growers, resulting in more efficient resource use and reduced effects to non-target organisms. Our results also confirm aggregation of SWD populations along wooded and field edges during the blueberry growing season. As illustrated in Iglesias and Liburd (2017), these findings support the use of border insecticide sprays and other targeted applications.

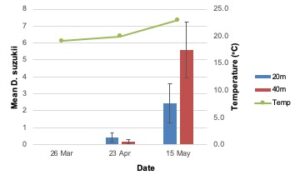



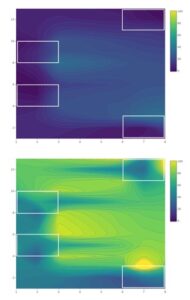
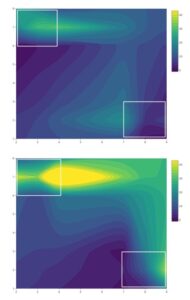
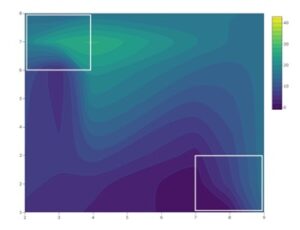
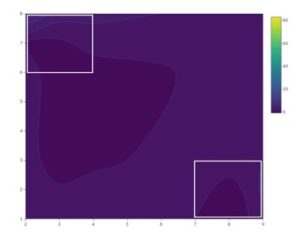
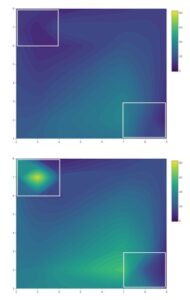
Objective 2 (HOOK evaluation cage study)
Results
Adult survival. Total SWD survivorship (males and females, combined) over the duration of the 72-hour study was not significantly different among cage treatments (χ2 = 0.76; P = 0.68; Table 5). Similarly, survivorship of males (χ2 = 0.34; P = 0.84) and females (χ2 = 0.45; P = 0.80) were not significantly different among treatments when analyzed individually. In all treatments, the largest proportion of SWD died within the first 12 hours of the study. Total mortality never exceeded 28% over the duration of the study.
Adult emergence. The number of adult SWD to emerge from tested blueberries was not significantly different among cage treatments (F2,15.74 = 1.76; P = 0.21; Figure 9) nor plant treatments (F = 0.161,14; P = 0.69; Figure 10). Similarly, no difference was observed when males (cage: F2,15.55 = 1.75; P = 0.21; plant: F1,14 = 0.00; P = 0.97) and females (cage: F2,15.94 = 1.56; P = 0.24; plant: F1,14 = 0.40; P = 0.54) were analyzed individually.
Discussion
Due to miscommunication between the UF SFVIPM Laboratory and ISCA Technologies, attractant-only HOOK (without toxicant) was used in the cage study without our knowledge. The absence of spinosad explains why survival and emergence were not different between choice and no-choice plots in the cage experiment. Even when plant treatment (HOOK or control) was analyzed separately from the cage treatment in which it was nested, emergence was not significantly different. Repeating this study, using HOOK with and without toxicant, is required to confirm these results. Low overall mortality is easily explained by the absence of toxicant in the HOOK product. We can assume any mortality observed was due to environmental factors within the experimental cages.


Objective 3 (HOOK evaluation field study)
Results
2018 (Conventional)
Adult trap catch. The number of adult SWD trapped was not significantly different among treatments (F = 1.85; df =2 ; P = 0.16) but was significantly different among weeks (F = 30.94; df = 4; P ≤ .0001), with the greatest trap catch observed on week 4 (11 May; Table 6). The treatment-by-week interaction was not significant. Similar trends were observed when the number of trapped females (treatment: F = 2.32; df = 2; P = 0.11; week: F = 13.5; df = 4; P = < .0001; treatment*week: F = 0.84; df = 8; P = 0.57) and males (treatment: F = 1.00; df = 2; P = 0.37; week: F = 38.43; df = 4; P = < .0001; treatment*week: F = 0.96; df = 8; P = 0.47) were analyzed individually.
Adult emergence. Overall, the number of adult SWD to emerge from collected blueberries was significantly different among treatments (F = 4.67; df = 2; P = 0.03) and weeks (F = 13.5; df = 1; P = 0.0032; Figure 11). No difference was observed among treatments in the first collection week (week 3), but emergence was significantly reduced in blueberries collected from treated plots on the second collection week. A significant treatment-by-week interaction was observed (F = 6.65; df = 2; P = 0.01).
2019 (Conventional)
Adult trap catch. Across all weeks, the greatest number of SWD were trapped in HOOK-7d plots (F = 18.15; df = 2 ; P < .0001; Figure 12). Trap catch in HOOK-14 plots was not significantly different than the control. The greatest trap catch across all treatments was observed on week 2 (26 Apr) (F = 14.37; df = 4 ; P < .0001). The treatment-by-week interaction was not significant. Similar trends were observed when the number of trapped females (treatment: F =15.33; df = 2; P < .0001; week: F = 15.09; df = 4; P = < .0001; treatment*week: F = 1.51; df = 8; P = 0.18) and males (treatment: F =11.31; df = 2; P = 0.0001; week: F = 10.97; df = 4; P = < .0001; treatment*week: F = 0.88; df = 8; P = 0.54) were analyzed individually.
Adult emergence. The number of SWD to emerge from collected blueberries was not significantly different among treatments (F = 1.55; df = 2; P = 0.22) or weeks (F = 1.25; df = 1; P = 0.27; Figure 13). No treatment-by-week interaction was observed.
2019 (Organic)
Adult trap catch. The number of adult SWD trapped was not significantly different among treatments (F = 2.07; df = 2 ; P = 0.14) but was significantly different among weeks (F = 46.62; df = 4; P < .0001; Table 7), with the greatest trap catch observed on week 4 (9 May). A significant treatment-by-week interaction was observed (F = 2.66; df = 8; P < 0.02). Trends were similar when the number of trapped females (treatment: F = 0.99; df = 2; P = 0.38; week: F = 31.89; df = 4; P = ≤ .0001; treatment*week: F = 2.29; df = 8; P = 0.04) was analyzed individually. When the number of trapped males was individually analyzed, significance was observed among treatments (F = 3.46; df = 2; P = 0.04) and weeks (F = 59.91; df = 4; P = < .0001). A significant treatment-by-week interaction was observed (F = 2.24; df = 8; P = 0.04).
Adult emergence. The number of SWD to emerge from collected blueberries was not significantly different among treatments (F = 0.08; df = 2; P = 0.92) or weeks (F = 1.15; df = 3; P = 0.35; Figure 14). The treatment-by-week interaction was not significant (F = 0.35; df = 6; P = 0.91).
Discussion
HOOK containing toxicant (spinosad) was used in the 2018 field study (conventional). As in the cage study, attractant-only HOOK (no toxicant) was used in the 2019 field studies (conventional and organic).
In the 2018 field study, adult trap captures from HOOK-treated plots were similar to control plots. SWD individuals are highly mobile and may move within and between large areas daily (Klick et al. 2016). Adult trap catch may have been influenced by immigration to treated fields from surrounding areas (Mensah & Macpherson 2010). Adult emergence was not different between treatments on the first collection date in 2018 but was reduced 15-fold in blueberries collected from HOOK-treated plots on the second collection date, suggesting that the application of HOOK provides additional control when added to the grower’s standard. Emergence from blueberries was not different between HOOK-7d and HOOK-14d plots, indicating that biweekly applications may be as effective as weekly applications. Applications of HOOK at biweekly intervals could have serious economic benefits for the grower, including labor cost savings and more efficient product use. The treatment-by-week interaction was significant, suggesting that HOOK may provide more effective control later in the blueberry season when SWD pressure is greater.
The use of an attractant-only product explains why more SWD were trapped in HOOK-7d plots than in biweekly-treated plots and control plots in the 2019 conventional study. These data provide evidence that the proprietary attractant in HOOK SWD effectively draws SWD to a target area when applied weekly. Ample evidence from both laboratory and field trials illustrates spinosad’s effectiveness for SWD control and its favorability from environmental and export perspectives (Sial et al. 2019; Van Timmeren & Isaacs 2013; Shawer et al. 2018; Cuthbertson et al. 2014; Haviland & Beers 2012). Given spinosad’s status as an industry standard, its inclusion in HOOK would reasonably result in pest mortality. The reasons why emergence in HOOK-treated plots (conventional, 2019) was not greater than in control plots is unclear. However, plot-specific factors, like the absence of oviposition sites (i.e. ripe fruit) or favorable microclimates (i.e. larger, less frequently pruned bushes) may have affected oviposition in treated plots.
Total adult trap catch was not significantly different among treatments in the 2019 organic field experiment. Volatiles within the environment may have interfered with attractant reception by SWD, or native attractants in the system may have elicited a stronger attraction response than the HOOK product (Cloonan et al. 2018; El-Sayed et al. 2009). Anecdotally, the organic plots in which this experiment was performed were in closer proximity to unmanaged areas and contained a higher density of understory weeds compared with the 2018 and 2019 conventional sites. These factors may have increased volatile “background noise” during the study and interfered with odorant reception. The conventional site utilized landscape fabric to mulch raised beds, and the organic site did not. It is believed that most SWD larvae pupate in the soil directly beneath crop hosts (Woltz and Lee 2017). The use of landscape fabric may have influenced population dynamics at the conventional site compared to the organic site. Additionally, the quantity of berries collected was greatly reduced in the 2019 field study, effectively reducing the sampling power in these treatments.
Together, data from studies using attractant-only HOOK and HOOK with toxicant suggest that the use of HOOK can increase SWD control in Florida's blueberry production systems. Future research should continue to test the effectiveness of HOOK in commercial blueberries. Studies can focus on optimizing the rate and deployment schedule of attract-and-kill for SWD. Interaction between deployed attractants and alternative attractive volatiles present in the field should be investigated in order to evaluate the effectiveness of synthetic attractants.

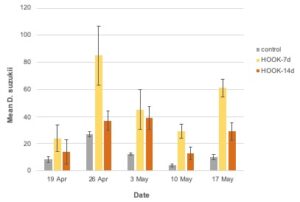
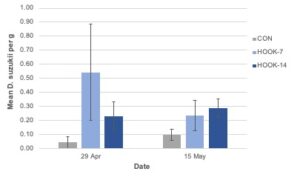
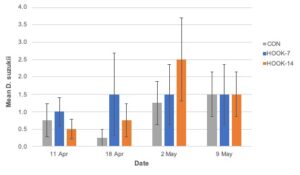
References
Cloonan, K. R., J. Abraham, S. Angeli, Z. Syed, and C. Rodriguez-Saona. 2018. Advances in the chemical ecology of the spotted wing drosophila (Drosophila suzukii) and its applications. J. Chem. Ecol. https://DOI.org/10.1007/s10886-018-1000-y.
Cuthbertson, A.G.S., L. F. Blackburn, and N. Audsley. 2014. Efficacy of commercially available invertebrate predators against Drosophila suzukii. Insects. 5: 952-960.
Diepenbrock, L. M., and H. J. Burrack. 2017. Variation of within-crop microhabitat use by Drosophila suzukii(Diptera: Drosophilidae) in blackberry. J. Appl. Entomol. 141:1-7. DOI: 10.1111/jen.12335.
El-Sayed, A. M., D. M. Suckling, J. A. Byers, E. B. Jang, and C. H. Wearing. 2009. Potential of “lure-and-kill” in long-term pest management and eradication of invasive species. J. Econ. Entomol. 102: 815-835.
Elsensohn, J. E., and G. M. Loeb. 2018. Non-crop host sampling yields insights into small-scale population dynamics of Drosophila suzukii (Matsumura). Insects. 9: 1-11. DOI: 10.3390/insects9010005.
Evans, R. K., M. D. Toews, and A. A. Sial. 2017. Diel periodicity of Drosophila suzukii (Diptera: Drosophilidae) under field conditions. PLoS ONE. DOI: 10.1371/journal.pone.0171718.
Haviland, D. R., and E. H. Beers. 2012. Chemical control programs for Drosophila suzukii that comply with international limitations on pesticide residues for exported sweet cherries. J. Integr. Pest Mngmt. 3. DOI: 10.1603/IPM11034.
Iglesias, L. E., and O. E. Liburd. 2017. The effect of border sprays and between-row soil tillage on Drosophila suzukii in organic blackberry production. J. Appl. Entomol. 141: 19-27.
Klick, J. W. Q. Yang, V. M. Walton, D. T. Dalton, J. R. Hagler, A. J. Dreves, J. C. Lee, and D. J. Bruck. 2016. Distribution and activity of Drosophila suzukii in cultivated raspberry and surrounding vegetation. J. Appl. Entomol. 140: 37-46.
Lee, J. C., A. J. Dreves, A. M. Cave, S. Kawai, R. Isaacs, J. C. Miller, S. Van Timmeren, and D. J. Bruck. 2015. Infestation of wild and ornamental noncrop fruits by Drosophila suzukii (Diptera: Drosophilidae). Ann. Entomol. Soc. Am. 108: 117-129.
Mensah, R. K., and I. MacPherson. 2010. Lure-and-kill as reduced-risk strategy for managing Helicoverpaspp. on conventional cotton crops within transgenic cotton fields. J. Biol. Control. 24: 91-103.
Pelton, E., C. Gratton, R. Isaacs, S. Van Timmeren, A. Blanton, and C. Guédot. 2016. Earlier activity of Drosophila suzukii in high woodland landscapes but relative abundance is unaffected. J. Pest Sci. 89: 725-733.
Shawer, R., L. Tonina, P. Tirello, C. Duso, and N. Mori. 2018. Laboratory and field trials to identify effective chemical control strategies for integrated management of Drosophila suzukii in European cherry orchards. Crop. Prot. 103: 73-80.
Sial, A. A., C. R. Roubos, B. K. Gautam, P. D. Fanning, S. V. Timmeren, J. Spies, A. Petran, M. A. Rogers, O. E. Liburd, B. A. Little, S. Curry and R. Isaacs. 2019. Evaluation of organic insecticides for management of spotted wing drosophila (Drosophila suzukii) in berry crops. J. Appl. Entomol. DOI: 10.1111/jen.12629.
Swoboda-Bhattarai, K. A. 2017. Determining factors that affect host use by the invasive vinegar fly Drosophila suzukii (Diptera: Drosophilidae). Ph.D. dissertation, North Carolina State University, Raleigh.
Van Timmeren, S., and R. Isaacs. 2013. Control of spotted wing drosophila, Drosophila suzukii, by specific insecticides and by conventional and organic crop protection programs. Crop Prot. 54: 126-133.
Woltz, J. M., and J. C. Lee. 2017. Pupation behavior and larval and pupal biocontrol of Drosophila suzukii in the field. Biol. Control. 110: 62-69.
Educational & Outreach Activities
Participation Summary:
We provide regular consultation to our participating growers and keep them abreast of current on-farm SWD pest pressure and findings from our research. I presented preliminary HOOK field findings (2018) at the Entomological Society of America Southeastern Branch annual meeting in March 2019 and discussed SWD trapping methods and efficacy at a University of Florida Doctor of Plant Medicine Program event called Natural Area Teaching Lab Trap Day. 2019 HOOK evaluation data will be presented at the Entomological Society of America national meeting in November 2019.
Project Outcomes
Data is inconclusive as to whether ACTTRA provides significantly better control of SWD larvae when added to the grower's standard management program. Because ACTTRA is applied to a localized area, specifically attractive to SWD, and utilizes an OMRI-approved reduced-risk insecticide, we believe it has a lower overall impact on non-target organisms, environmental health, and poses less health risk for farmers and farm employees compared to a traditional foliar spray. We suspect that growers will see higher yields and more stability in Florida's valuable, niche blueberry market. In 2018, we observed that biweekly ACTTRA applications provide the same level of SWD larval control as weekly applications, which can save growers time, money, and labor.
Preliminary data (2018) from the attract-and-kill component of this project suggests that ACTTRA is a tool that my advisor and I can recommend to growers for increased control of spotted wing drosophila (SWD) larvae. However, more field studies on both conventional and organic farms should be performed to confirm these findings. While researching the monitoring component of this project, I learned how to effectively deploy and service traps for SWD, identify and count SWD, and analyze trap data. In investigating the ACTTRA component of this project, I learned the importance of a multi-faceted management program for sustainable blueberry production. I also found that Florida's commercial blueberry farmers are eager to adopt emerging technologies that can reduce their insecticide use.
Options for future study include evaluation of ACTTRA for SWD control alone, in absence of the grower's standard. Because the SARE-funded research is currently underway, the final report will share results from the other objectives of this project.