Final report for GS20-222
Project Information
Bahiagrass pastures (Paspalum notatum Flugge; BG) dominate Florida grazing land. Grown on over one million hectares, this warm-season perennial grass produces abundant, low-quality forage. Seasonal dormancy and low soil fertility limit BG production and require feed and fertilizer inputs to support forage production and quality. However, high input costs and scrutiny of the carbon footprint associated with fertilizer and feed production and transport highlight the need for sustainable alternatives. Integrating legumes and over-seeding cool-season annual forages into BG pastures supplies N through biological N2-fixation while cool-season annuals extend the grazing season, thus reducing the need for fertilizer N and stored feed. Species diversification of BG pastures over time and space, referred to here as agroecological intensification (AEI), may enhance soil organic matter (SOM) and improve nutrient cycling by changing the quality, quantity and timing of organic inputs. Despite advances in mixed BG-rhizoma peanut (Arachis glabrata Benth; RP) research, the influence of RP proportion and other forage species on below-ground responses linked to SOM formation and nutrient cycling remains understudied. This project aims to evaluate increasingly intensified pasture systems for forage productivity and quality and to understand root-rhizome and microbial outcomes of intensification and pasture composition. Ultimately, it seeks to maximize pasture productivity, promote on-farm resource use efficiency and enhance ecosystem services by supporting SOM and year-round plant growth to reduce external inputs.
- To evaluate the productivity and quality of forage systems along a gradient of AEI. Specifically, we will examine both overall productivity and quality as well as the smoothness of their seasonal distribution (i.e. ability to reduce seasonal over/under-supply), given the large importance of the latter to realizing production benefits in practice.
- To evaluate root and rhizome production along a gradient of AEI, and to link these below-ground responses to SOM formation and soil fertility indicators.
- To explore how variations in legume proportion, soil quality (indexed by baseline SOM) and soil resource supply (including fertility and soil moisture) relate to variations in the realized production and ecological benefits of AEI.
Research
Experimental design: Forty-eight 1.4-m x 1.4-m experimental plots were established at the Beef Research Unit in Gainesville, Florida in an existing system of experimental plots of 'Pensacola' BG-dominated and BG-RP pasture. Bahiagrass-RP contributes to plant diversity within a given pasture by growing two warm-season forages concurrently. The 'Ecorturf' RP variety was selected for its high nutritive value, grazing tolerance and persistence. Over-seeded cool-season annual forage treatments add a temporal diversity dimension by growing multiple species at different times. Cool-season species were selected based on previous research and life cycle: rapid germination of rye and oat provide early cool-season growth, followed by annual ryegrass peaking in January, and late emergence of red clover (Fonatelli & Sollenberger, 2000). Per common practice, we will supply cool-season annual forages with 18 kg/ha of N fertilizer. To maximize the real-world relevance of our results, and to isolate N-related from non-N-related benefits of AEI, we include both unfertilized and N fertilized bahiagrass (Bahiagrass-N, 2 split doses of 40 kg N/ha) as treatments.
A completely randomized block design with blocks of warm-season perennials overlaid with a cool-season annual forage treatment will consist of:
1) Bahiagrass
- without cool-season grasses
- with over-seeded cool-season grasses + N fertilizer
- with over-seeded cool season grasses + N fertilizer + legume
2) Bahiagrass-N
- without cool-season grasses
- with over-seeded cool-season grasses + N fertilizer
- with over-seeded cool season grasses + N fertilizer + legume
3) Bahiagrass-Rhizoma peanut
- without cool-season grasses
- with over-seeded cool-season grasses + N fertilizer
- with over-seeded cool season grasses + N fertilizer + legume
4) Bahiagrass-N - Rhizoma peanut
- without cool-season grasses
- with over-seeded cool-season grasses + N fertilizer
- with over-seeded cool season grasses + N fertilizer + legume
All plots will be maintained with simulated grazing. Based on previous research, we determined that a 4-wk frequency of defoliation to 5 cm represents a decent compromise to simulate grazing utilization while maintaining adequate persistence and quality in both BG and RP (Stenklyft, 2017; Mislevy et al., 1991).
Objective 1:
To quantify pasture productivity, quality and their seasonal distribution at each level of AEI, aboveground biomass samples will be collected monthly from each plot using a 0.0625 m2 quadrat. Measures of monthly fresh and dry biomass weights summed together amounts to the total forage production of each plot. Forage tissue samples analyzed for crude protein and in vitro digestible organic matter will measure forage nutritive value. Total production and quality as a function of position on the AEI gradient will be analyzed with mixed-effects linear regression, while seasonal distribution will be quantified using measures of dispersion and Fourier series analysis. Taken together, this information will illustrate the productive potential of AEI pastures compared with conventional BG pastures with and without N fertilizer and combined with our forage quality measures will inform on the potential to improve animal production and profitability.
Objective 2:
To quantify below-ground root and rhizome production and link them to changes in SOM and microbial biomass at each level of AEI, we will combine sampling of soil cores, with root in-growth core methods.
Changes in SOM and soil microbial biomass will be measured by collecting soil cores prior to the initiation of treatment implementation and at the close of the experiment. Since bulk SOM often takes many years to change appreciably in response to management interventions, size-density fraction will be used to separate pools of organic matter that turn over on different timeframes. In particular, we will use the fast-turnover light coarse fraction as a leading indicator for changes in SOM. Soil analysis using an elemental analyzer will quantify total soil C and N content, while a modified slurry chloroform fumigation extraction (Fierer et al., 2003) will quantify microbial biomass, which responds very quickly to management.
While soil cores give useful snapshots of the below-ground ecosystem, on their own they cannot be used to measure below-ground production since turnover is not accounted for. For this purpose, we will collect three in-growth cores per plot per year corresponding to early warm-season (May), late warm-season (September), and peak cool-season (January). In each extracted core, we will separately weigh roots and rhizomes and analyze for total C and N content and δ13C using elemental analysis. The isotopic δ13C from cores in mixed plots will enable partitioning of the observed biomass into a C4 BG component and a C3 RP component.
Soil and microbial C and N will be measured from root in-growth cores using the above methods. We will then analyze the relationship between below-ground production and changes in our leading indicators of microbial and soil light fraction C and N using linear regression while accounting for treatment and block.
Objective 3:
Separate from our analyses of the impacts of AEI treatment, we will examine how pre-existing, underlying differences in plot characteristics (e.g. SOM, fertility, average soil moisture, proportion of RP) impact variations in the realized production and below-ground benefits of our treatments. This will provide useful and novel insight not only to extend the general framework of AEI, but also will be directly actionable information for farmers and ranchers.
We will measure proportion of legume in the above-ground biomass samples described in Objective 1 by hand separation. Meanwhile, root-rhizome δ13C isotope results described in Objective 2 will be used to inform on below-ground proportions from C3/C4 species. Linking information about the ratio of RP to BG to the information obtained in Objectives 1 and 2 can guide management by targeting specific RP proportions based on expected productive and ecological outcomes.
Plot level SOM and fertility will also be collected as described in Objective 2. Moreover, because our experimental paddocks are blocked along a gradient of soil moisture, weekly soil moisture measurements will be taken using a Hydrosense sensor to 11-cm depth. These characteristics will be used to explain variations in both total forage production, quality and their seasonality using linear regression techniques. The insight gained here will assist scientists and farmers in predicting the benefits from AEI strategies, and guide management in how to optimize them.
The aim of this study was to evaluate the performance of warm-season perennial pastures in terms of forage production and quality along an AEI gradient of increasingly intensified management as well as the impact of AEI on soil organic matter.
Objective 1: Forage productivity and quality
The first objective of this study was to examine the quantity, evenness, and quality of forage production across an AEI gradient. Annual forage accumulation varied by year, with less forage accumulated in 2022 compared with 2021 (Figure 1; Table 1). In year 1 of the study, a trend towards increasing forage accumulation with increasing management intensification was observed. Inclusion of the warm-season legume rhizoma peanut (RP) had a greater impact on increasing total annual forage than did applying warm-season N fertilization alone and in combination with overseeding cool-season grasses + legumes (Table 1). Rhizoma peanut enhanced the rate of warm-season forage regrowth between defoliation events in May-October in 2021 and from June-September in 2022 (Figure 2). Warm-season N fertilization and over-seeding cool-season forages improved the evenness of forage accumulation in BG during the second year of the study (Figure 3). Over-seeding cool-season grasses + legumes and applying 40 kg N ha-1 plus overseeding cool-season grasses had similar effects on promoting evenness compared with unfertilized BG. In 2022, all treatments with BGRP displayed greater evenness of forage production than unfertilized BG.
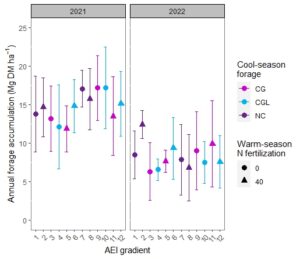
Table 1. Intercept and varying slopes from the model for forage accumulation. Coefficients represent deviations of each fixed effect (AEI gradient) from the baseline bahiagrass (BG) up to inclusion of rhizoma peanut (BGRP), warm-season N fertilization (40 kg N/ha), and cool-season grasses (CG) plus cool-season grasses+legumes (CGL). Negative values represent comparatively lower forage accumulation and positive values comparatively higher forage accumulation.
AEI gradient |
Experimental treatments |
Coef. est. ± SE |
1 |
BG (intercept) |
8.11 ± 0.70 |
2 |
BG40 |
0.39 ± 0.85 |
3 |
BGCG |
-1.60 ± 0.85 |
4 |
BGCGL |
0.96 ± 0.86 |
5 |
BG40CG |
1.79 ± 1.21 |
6 |
BG40CGL |
0.05 ± 1.21 |
7 |
BGRP |
1.14 ± 0.85 |
8 |
BGRP40 |
0.37 ± 1.21 |
9 |
BGRPCG |
1.74 ±1.21 |
10 |
BGRPCGL |
-0.95 ± 1.21 |
11 |
BGRP40CG |
-0.74 ± 1.71 |
12 |
BGRP40CGL |
-0.48 ± 1.71 |
|
Year |
-4.45 ± 0.29 |
Forage nutritive value
We examined the protein content and digestibility of forages to evaluate the effect of different management practices on forage quality. Nitrogen serves as a proxy for crude protein, since it is essential in the amino acids that build proteins. Rhizoma peanut inclusion enhanced the forage N concentration compared with unfertilized bahiagrass, even in the cool-season when RP was not present in the botanical composition due to dormancy (Figure 4). The improved forage quality may be the result of a greater rate of N mineralization in the presence of RP (Kohmann et al., 2019). Nitrogen concentration of cool-season forages ranged from 14.15 g kg-1 for BG40CG (5 on the management intensification gradient) to 19.00 g kg-1 for BGRP40CGL (12 on the management intensification gradient). Warm-season forage N concentrations were between 14.09 g kg-1 for unfertilized BG to 18.61 g kg-1 for BGRPCG (8 on the management intensification gradient). These values are slightly lower than those observed for pure RP stands in the Florida Panhandle (Dubeux et al., 2017), likely due to the inclusion of grasses, which are of lower nutritive value than legumes in general.
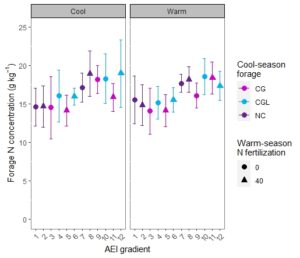
In vitro organic matter digestibility was slightly higher on average for cool-season forages than for warm-season forages (Figure 5). Averaged across treatments, IVOMD was 695.64±84.42 g kg-1 for cool-season forage and 629.69±49.98 g kg-1 for warm-season forage. The slightly higher IVOMD values for cool-season forages are likely due to differences in the leaf tissue anatomy between C3 and C4 plants. Bahiagrass, a warm-season (C4) grass, has more complex carbohydrates in the cell wall than found in broadleaf and cool-season (C3) plants like RP, rye, oat, ryegrass, and clovers (Coleman et al., 2004). Consequently, C4 forage tends to be less digestible than C3 forage. Our range for cool-season forages was slightly lower than the averages observed for rye-oat, rye-oat-ryegrass, rye-oat-crimson clover, rye-oat-ryegrass-crimson clover, rye-oat-red clover, and rye-oat-ryegrass-red clover in 1996 and 1997 (706 and 736 g kg-1, respectively; Fonatelli et al., 2000). The lower IVOMD of cool-season forages that we observed might be explained by less N fertilization applied in our study (40 kg N ha-1) compared with Fonatelli et al. (2000; 120-160 kg N ha-1).
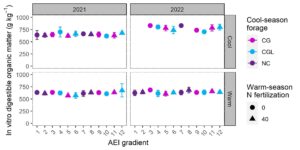
Objective 2: Belowground impacts on soil microbial biomass C and SOM
Our second objective was to quantify below-ground root and rhizome production and link them to changes in microbial biomass and SOM at each level of AEI. Soil microbial biomass C ranged from 0.03-0.06 mg C g soil-1 in the cool season and 0.02-0.05 mg C g soil-1 in the warm season. A decline in soil microbial biomass C was observed as C in roots and rhizomes increased during the cool season but the trend changed directions in the warm season (Figure 6). At lower levels of root and rhizome C, BGRP enhanced soil microbial biomass C. Meanwhile the application of 40 kg N ha-1 in May 2022 enhanced soil microbial biomass relative to 0 kg N ha-1 although the two had similar increasing slopes.
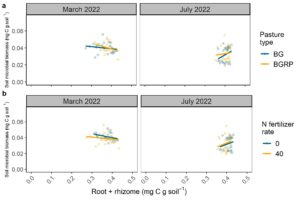
Greater variation was observed in the C and N concentrations in the coarse sand fraction than in the medium to fine soil fraction at the beginning of the experiment but variation in this fraction declined after two years of applying the experimental treatments (Figure 5a and 5b). The δ13C signature was more negative (more C4) in the coarse sand fraction than in the medium to fine fraction, suggesting greater contributions from BG (Figure 5c). However, after two years of applying the experimental treatments, the δ13C signature in the coarse sand fraction slightly declined, suggesting greater contributions of C3 materials (e.g. RP and cool-season forages). The δ15N signature of medium to fine sand was enriched from the beginning to the end of the experiment, suggesting that a low rate of warm-season N fertilization enhanced N in these soils since N isotopic signatures near zero suggest active biological N fixation (Dubeux et al., 2017), which would have contributed more to the δ15N signature in the absence of N fertilization.
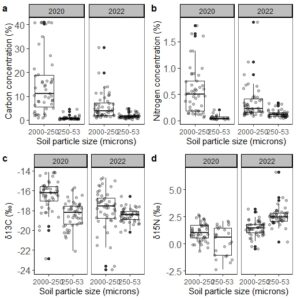
The effect of the experimental treatments on soil fertility was to increase the spread of values observed. Higher pH, Ca, and Mg had a positive impacts on net primary productivity (NPP, Figure 8a, 8d, 8e). The range of P and K expanded by the end of the study (Figure 8b, 8c). Copper was enriched from 0.2-0.6 mg kg-1 in year 1 of the study to 0.4-0.8 mg kg-1 (Figure 8g). The range of manganese and zinc both increased slightly from the start to the end of the project, which corresponding gains in NPP (Figure 8h, 8i).
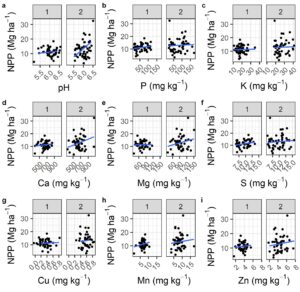
Objective 3: Impacts of the proportion of rhizoma peanut and soil moisture
The proportion of RP increased from 2021 to 2022 (Figure 9). Rhizoma peanut enhanced forage accumulation in 2021 compared with sole bahiagrass. As the proportion of RP increased in 2021, greater forage accumulation was observed with 0 kg N ha-1 than given 40 kg N ha-1. However, greater forage accumulation was observed at all levels of proportion RP for 40 kg N ha-1 in 2022 than for 0 kg ha-1.
Soil volumetric water content was greater on average in 2021 than in 2022 (Figure 11). Cumulative precipitation during the warm season was approximately 220 mm greater in year 1 of the study compared with year 2 (Table 2). The proportion of RP as a component of the forage biomass had a greater range in 2022 (Figure 11). This is likely due to superior performance of RP under limited precipitation than BG (Williams, 1994). Reduced precipitation in June and July 2022, when evaporative pressures are high, may have stunted BG growth that year due to limited soil water availability. Thus, RP constituted a greater proportion of the total forage biomass in the drier of the two years of the study.
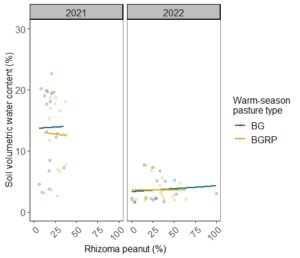
Educational & Outreach Activities
Undergraduate student volunteer Hannah Shareen presented a poster related to her work on this project at the fall 2021 University of Florida Center for Undergraduate Research Center symposium in Gainesville, Florida, USA.
Participation Summary:
To date, twenty University of Florida undergraduate students and a visiting scholar from Brazil have been involved in carrying out the project activities. Through their participation, they are gaining valuable field and laboratory research experience. One student volunteer obtained a University Scholar Award to collaborate on the soil microbial biomass C and N component of this project and will present a paper and poster later this spring.
Project Outcomes
This project examined the potential for a suite of low-input management practices such as legume inclusion and overseeding cool-season forages to produce forage in similar quantities and of similar quality as intensively managed (e.g. high N fertilization) bahiagrass pastures. Additionally, we aimed to quantify the impact of these low-input practices on soil organic matter. We established an agroecological intensification (AEI) gradient to evaluate how incremental increases in management practices influenced pasture performance. Our results indicate that inclusion of rhizoma peanut had the strongest effect on forage production and soil organic matter, likely due to the longer-term implementation of this treatment compared with warm-season N management of 40 kg N ha-1 yr-1 and overseeding cool-season forages (7 vs. 2 years, respectively). Additional years of implementing experimental treatments may produce results that indicate greater changes over time.
Key lessons learned about sustainable agriculture:
- Warm-season pasture management: Year-to-year variation had more of an impact on pasture outcomes than did the experimental treatments. Legume inclusion can enhance the productivity of bahiagrass pastures. Application of a low N fertilization rate of 40 kg N ha-1 yr-1 to warm-season pastures can have variable results.
- Skills: This project trained graduate and undergraduate students in multiple field and laboratory methods. For example, students executed a soil fractionation and permanganate oxidizable C (POXC) methods to quantify C and N in soils. The latter method was much faster making it a more practical option for broad adoption for quantifying soil organic matter. Monthly harvests were collected and sorted by hand to separate grasses from legumes. Future research should reduce the frequency of sorting biomass to spend more time learning additional methods. Additionally, several undergraduate and graduate students gained valuable experience analyzing data and communicating results in scientific forums. They gained skills using R statistical software and preparing scientific posters, for example.
- Pasture evolution: Warm-season pastures were established in 2015 in plots of sole 'Pensacola' bahiagrass and mixed 'Pensacola' bahiagrass+'Ecoturf' Rhizoma peanut. Seven years after establishment, we observed that 'Ecoturf' rhizoma peanut spread into sole 'Pensacola' bahiagrass plots. This coincides with previous research on rhizoma peanut that reported successful colonization of C4 grass pastures over time (Mullenix et al., 2014). The invasion of BG plots with BGRP may have contributed to limited differences observed along the AEI gradient.
- Cool-season forage establishment. Cool-season forages were seeded within the UF/IFAS recommended date range for the study location and irrigation was applied during the first month after overseeing warm-season pastures. Yet, cool-season forage growth was slow and limited. In year 2 of the study, a promising establishment was set back by wildlife grazing. Other set backs might include competition from the warm-season pasture and inadequate soil moisture, among others.
Year 2:
- A complementary study was established in November 2021 at the UF|IFAS Plant Science Research and Education Unit in Citra, Florida, USA to build off of the observations in year 1 (see first bullet point below). Cool-season grasses and cool-season grasses+legumes were established under three land preparation strategies (tillage, no-tillage, no-tillage+herbicide) and four N fertilizer rates (0N, split 30-50lbsN, split 50-30lbsN, and 80N) treatments. Irrigation was applied to all plots for the first month after planting. Leaf area index (LAI; a measure of light interception) and above-ground biomass was harvested 60, 90, and 120 days after planting. Data from year 1 of this new study is being analyzed.
Year 1:
Several project ideas have emerged as a result of the observations made during the first season of this project. These include:
- Timing and method of cool-season forages in warm-season pasture. Suggested treatments would be to 1) kill warm-season foliage with a contact herbicide prior to overseeding cool-season forages, 2) overseed cool-season forages after the first killing frost burns warm-season foliage. An additional layer could be study irrigated vs. non-irrigated cool-season forages.
- Below-ground activity of warm-season pasture and cool-season forage establishment. Is warm-season root and rhizome activity competing with newly-established cool-season forages? For example, is N immobilization by warm-season pasture limiting cool-season forage acquisition during initial growth stages?