Final report for LS20-337
Project Information
Managing insects in cucurbit crops is a longstanding challenge due to conflicting needs of intense pest management and strong pollination services. The cucurbit pest complex is notoriously problematic because it includes insects that inflict considerable feeding damage and are also vectors of pathogens that threaten total crop loss. Intense broad-spectrum pesticide use can harm predatory insects and pollinators that are critical for profitable yields. Stronger cultural tools and more selective insecticides are needed to limit pest pressure and conserve ecosystem services by beneficial insects. Colored and reflective mulches are commonly employed to promote plant growth and repel herbivores, but their effects on predator and pollinator attraction are unclear. Likewise, living mulches planted between plastic-mulched rows may buffer the effects of insecticide applications on beneficial insects by providing refuge habitat. However, ecosystem services and potential disservices associated with living mulches in conventionally-managed systems are not well-documented, and for that reason, they are rarely incorporated in conventional vegetable production. To that end, we proposed a research project and a series of experiments manipulating colored mulches, living mulches, and applications of broad spectrum and selective insecticides across three Southeastern states. Our goal is to develop novel cultural tools that better harmonize chemical and biological control, and evaluate their utility in an economic framework that balances risks, costs and benefits in each system. We also demonstrated and deployed these tools by engaging with growers in workshops, field days, and grower conferences across the Southeast.
Objective 1. Determine the effects of colored plastic mulch on the production of cucumber and squash and their pest and beneficial insect communities
Objective 2. Harmonize chemical and biological control in cucurbit systems by integrating plasticulture with living mulches between beds
Objective 3. Assess the impact of augmentative releases of the egg parasitoid Hadronotus pennsylvanicus (formerly Gryon pennsylvanicum) on squash bug populations in squash.
Objective 4. Estimate the economics of different plastic mulch colors, living mulches between beds, and reduced risk pesticides
Cooperators
- - Producer
- - Producer
- - Producer
- - Producer
- - Producer
- - Producer
- - Producer
Research
Objective 1. Effects of colored plastic mulch on cucurbit production and insect communities.
The agronomic and weed suppression benefits of growing vegetables on plastic mulch are well documented (Decoteau et al. 1986, Lament 1993, Greer and Dole 2003, Andino and Motsenbocker 2004, Frank and Liburd 2005, Gibson et al. 2011). Colored mulches, in particular aluminated (silvery) or reflective mulch, have also been shown to suppress a number of insect pest species (Wolfenbarger and Moore. 1968, Cartwright et al. 1990, Henshaw et al. 1991, Caldwell and Clarke. 1999, Greer and Dole 2003, Nyoike and Liburd. 2010). However, their effects on squash bug, Anasa tristis, are not completely known, and this insect pest can be quite damaging to summer squash in the southeastern U.S.
Experimental Design
We conducted field experiments to test the effects of mulch color on A. tristis during the summers of 2019 and 2020 at Kentland and Homefield Farms in Whitethorne, VA. To do so, we constructed four treatments of plastic mulch beds (1.2 x 9.1 m /block) as a Latin square design in early June. Treatments included black, white, and reflective mulch (Berry Hill Irrigation, Buffalo Junction, VA) and bare ground. Zucchini seeds (var. Dunja, Harris Seeds) were sown directly in mid-June at 0.76 m spacing within each treatment bed. Seven to ten days after sowing, stand counts were taken for each germinated treatment bed. Plots with sparse germination (< 50%) were replanted with greenhouse grown seedlings (sown on the same date as in the field) so there were at least ten plants per plot. Tiffany teff grass (Hancock Seed Company, Dade City, FL) was sown between plastic mulch rows at a rate of 11 kg seed/ha, acting as a living mulch and weed suppressant throughout the experiment. A 9% concentration (total fatty acid) solution commercial blend of caprylic and capric acid (Home Plate non-selective herbicide; Certis USA, Columbia, MD) was applied to bare ground plots to manage weeds on two dates; before squash germination and immediately following germination. Subsequent weed management was accomplished through weekly manual removal of weeds.
Insect Sampling
Approximately three weeks after zucchini germination, we began sampling for squash bug at weekly intervals. Five randomly selected plants within each plot were surveyed for adults, nymphs, and egg masses. Surveys included visual checks of the entire plant and the plastic mulch directly below the plant, specifically focusing on the spaces where the plant stem meets the mulch and along the mulch teff grass margin. Additional consideration was given to these areas because previous research suggested that A. tristis adults and nymphs commonly reside in inconspicuous or hidden areas adjacent to the plants (Palumbo et al. 1991a, Doughty et al. 2016). In total, insect sampling was performed for six consecutive weeks, starting the first week of July and ending the second week in August.
Marketable Fruit Sampling
Once plants began to develop fruit, we harvested all undamaged, marketable zucchini from each plot three times per week from the fourth week of July through the second week of August by manually picking all market sized fruit (220-250 g). Damaged fruit was picked during each harvest date but was discarded and not included in our marketable fruit yield counts. Numerous factors, such as improper pollination, microbial pathogens, and feeding damage from other cucurbit insect species, can influence summer squash fruit quality. Since we did not specifically account for these potential predictors of fruit quality, the number of unmarketable fruit was not recorded as an additional response variable.
Statistical Analysis
Insect count and marketable yield data were separated by year. The number of marketable fruit collected per plot was summed for each sampling week and divided by the number of plants within each plot. Weekly A. tristis counts and marketable fruit yield/plant were normalized using a square-root transformation. To test the fixed effects of mulch treatment, sampling week, and their interaction on insect counts and yield, we used a generalized linear mixed model (GLMM) with block and site location as random factors. Although we were not interested in the effect of sampling week alone, it was included as a fixed factor in order to test its interaction with mulch treatment on A. tristis counts and yield. This interaction allowed us to distinguish whether the effect of mulch treatment was consistent across our A. tristis and yield sampling periods. Multiple comparisons between mulch treatments were conducted using a Tukey’s honestly significant difference (HSD) test (α = 0.05). All statistical analyses were completed using JMP 15.0.0 software.
References Cited:
Andino and Motsenbocker. 2004. Colored plastic mulches influence cucumber beetle populations, vine growth, and yield of watermelon. HortScience. 39:1246-1249.
Caldwell and Clarke. 1999. Repulsion of cucumber beetles in cucumber and squash using aluminum-coated plastic mulch. HortTechnology. 9:247-250.
Cartwright et al. 1990. Influence of crop mulches and row covers on the population dynamics of the squash bug (Heteroptera: Coreidae) on summer squash. J. Econ. Entomol. 83:1988-1993.
Decoteau et al. 1986. Mulch surface color affects yield of fresh-market tomatoes. J. Amer. Soc. Hort. Sci. 114:216-219.
Frank and Liburd. 2005. Effects of living and synthetic mulch on the population dynamics of whiteflies and aphids, their associated natural enemies, and insect transmitted plant diseases in zucchini. Environ. Entomol. 34:857-865.
Gibson et al. 2011. Effect of a living mulch on weed seed banks in tomato. WeedTech. 25:245-251.
Greer and Dole. 2003. Aluminum foil, aluminum-painted, plastic, and degradable mulches increase yields and decrease insect-vectored viral diseases of vegetables. HortTechnology. 13:276-284.
Henshaw et al. 1991. Use of reflective mulches in control of mosaic viruses in summer squash. Natl. Agr. Plastics Congr. Proc. 23:78-83.
Nyoike and Liburd. 2010. Effect of living (buckwheat) and UV reflective mulches with and without imidacloprid on whiteflies, aphids and marketable yields of zucchini squash. Int. J. Pest Manage. 56:31-39.
Wolfenbarger and Moore. 1968. Insect abundances on tomatoes and squash with aluminum and plastic sheetings. J. Econ. Entomol. 61:34-36.
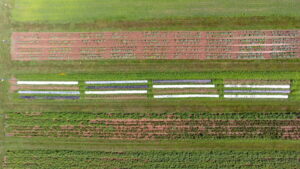
References Cited:
Andino and Motsenbocker. 2004. Colored plastic mulches influence cucumber beetle populations, vine growth, and yield of watermelon. HortScience. 39:1246-1249.
Caldwell and Clarke. 1999. Repulsion of cucumber beetles in cucumber and squash using aluminum-coated plastic mulch. HortTechnology. 9:247-250.
Cartwright et al. 1990. Influence of crop mulches and row covers on the population dynamics of the squash bug (Heteroptera: Coreidae) on summer squash. J. Econ. Entomol. 83:1988-1993.
Decoteau et al. 1986. Mulch surface color affects yield of fresh-market tomatoes. J. Amer. Soc. Hort. Sci. 114:216-219.
Frank and Liburd. 2005. Effects of living and synthetic mulch on the population dynamics of whiteflies and aphids, their associated natural enemies, and insect transmitted plant diseases in zucchini. Environ. Entomol. 34:857-865.
Gibson et al. 2011. Effect of a living mulch on weed seed banks in tomato. WeedTech. 25:245-251.
Greer and Dole. 2003. Aluminum foil, aluminum-painted, plastic, and degradable mulches increase yields and decrease insect-vectored viral diseases of vegetables. HortTechnology. 13:276-284.
Henshaw et al. 1991. Use of reflective mulches in control of mosaic viruses in summer squash. Natl. Agr. Plastics Congr. Proc. 23:78-83.
Nyoike and Liburd. 2010. Effect of living (buckwheat) and UV reflective mulches with and without imidacloprid on whiteflies, aphids and marketable yields of zucchini squash. Int. J. Pest Manage. 56:31-39.
Wolfenbarger and Moore. 1968. Insect abundances on tomatoes and squash with aluminum and plastic sheetings. J. Econ. Entomol. 61:34-36.
Objective 2. Harmonize chemical and biological control in cucurbit systems by integrating plasticulture with living mulches between beds
Agricultural intensification and pesticide use often come at a cost of ecosystem services delivered by beneficial insects. However, re-integrating diverse and structurally complex non-crop habitat may provide food and refuge resources that mitigate non-target effects on natural enemies and enable rapid recolonization of pesticide-treated crop areas, harmonizing chemical and biological control. Here, we manipulate pesticide treatments and living between rows of zucchini crops across four replicated experiments in Georgia, North Carolina, and Virginia, to determine how refuge habitat moderates non-target effects on natural enemies. The experiment was established in 2021 at four locations: Virginia Tech Eastern Shore AREC in Painter, VA; Homefield Farm in Whitethorne, VA; MHCR&EC in Mills River, NC, and Durham Horticultural Research Farm, Athens, GA; to assess the effect of a living mulch plant mixture of teff grass, buckwheat and red clover sown between plastic-mulched rows compared to bare ground between plastic beds on the insect pest and beneficial community in a squash production agroecosystem. We assessed each mulch treatment under routine insecticide applications versus no insecticides. We predicted that a buckwheat, clover, teff grass living mulch will provide habitat resources that can buffer effects of insecticide treatments on beneficial insects (Hooks et al. 1998, Lee et al. 2001, Frank and Liburd 2005). Therefore, we hypothesized that pest pressure would be lowest in living mulch treatments.
Methods
Study sites. Field experiments were conducted in 2021 at four replicated sites at the University of Georgia’s Durham Horticultural Research Farm (33.8869 º N, -83.4211 º W), at North Carolina State University’s Mountain Horticulture Crop Research and Extension Center (35.4172 º N, -82.5613 º W), Virginia Tech’s Kentland Farm (37.198236º N, -80.582786º W), and Eastern Shore Agricultural and Extension Center (37.58403º N, -75.82127º W). Our shared protocol is described below, but see Appendix S1
Experimental design
Four factorial combinations of living mulch and broad-spectrum insecticide treatments (Pyganic® – pyrethrin) in five replicates were set up in a randomized block design at each site to measure interactive effects of living mulches and pesticides on natural enemies, as well as key insect pests. Plots were 5.5 m wide x 7.3 m long with three raised beds randomized within five replicated blocks and 2 m bare soil boundaries between plots (Figure S.1). Zucchini crops (Cucurbita pepo subsp. pepo cv. ‘Yellow Fin’ or ‘Spineless Beauty’) were direct-seeded into plastic-mulched beds at 0.6 m spacing, totaling 12 plants per bed and 36 total plants in each plot. Any un-germinated plants were replanted within two weeks with transplants that were started on the original day of planting in 50-cell transplanting trays. Experimental treatments were as follows: 1) Bare ground between beds + no insecticides, 2) Bare ground between the beds + insecticide treatments, 3) Living mulch between beds + no insecticides, and 4) Living mulch between beds + insecticides.
Living mulch treatments: Living mulch plots were sown with three species: crimson clover (Trifolium incarnatum, Fabaceae; inoculated with PreVail Azospirillum brasilense (nitrogen-fixing, gram-negative bacteria), teff grass (Eragrostis tef, Poaceae), and buckwheat (Fagopyrum esculentum, Polygonaceae) at rates of 27 kg, 22.4 kg, and 56 kg/ha, respectively. Living mulch seed was incorporated between plastic-mulched beds shallowly with a rake, and supplemental irrigation was provided as needed. Management of ‘bare soil’ pathways and the 2 m buffers between beds consisted of hand weeding and use of stirrup and wheel hoes to maintain relatively uniform, weed-free environments. Herbicides and fungicides were applied at the North Carolina site. (See appendix for unique site-management details)
Left: Bare ground (in front) versus living much plots (in back) established in Whitethorne, VA. Right Photo: Living mulch plots in Georgia.
Insecticide treatments: Pesticide applications began in pesticide treatment plots every 10 days, consistent with intense pesticide management in organic cucurbit systems, once pests became prevalent in the field. Pyganic® (OMRI approved) was diluted at a rate of 12 ml per L water, and applied to foliage with a backpack sprayer at an approximate rate of 580 L/ha over the 10 pesticide treatment plots during the evening after zucchini flowers closed.
Insect sampling Visual arthropod sampling occurred every 10 days, approximately 2-3 days after each pesticide application to best observe recolonization from living mulch treatments after pesticides degraded (see appendix for specific sampling dates at each site). Six leaves (two new, two middle-aged leaves, and two mature leaves) from six randomly selected zucchini plants from the middle row of each plot were examined. Counts of the two key zucchini pests: cucumber beetles (Diabrotica spp. and Acalymma vitattum F., Coleoptera: Chrysomelidae) and squash bugs (Anasa spp., Hemiptera: Coreidae) were recorded for each plot along with natural enemies including spiders (Araneae), lady beetles (Coleoptera: Coccinellidae), big-eyed bugs (Hemiptera: Geocoridae), minute pirate bugs (Hemiptera: Anthocoridae), damsel bugs (Hemiptera: Nabidae) and long-legged flies (Diptera: Dolichopodidae). The numbers of natural enemies or insect pests present in the living mulches were not recorded.
Harvest protocol. Once fruits matured, yield assessment occurred twice a week. Fruits larger than 18 cm were harvested from the center 2 m in each plot. Fruits were graded as marketable (<2.2 kg with no visible fruit defects), or unmarketable (cull, overgrown fruit (>2.5 kg), fruits displaying deformity or noticeable insect damage).
Statistical analysis. All analyses were performed in R version 4.0.2. Natural enemies, cucumber beetles, and squash bugs were analyzed as annual sums per plot with generalized linear mixed models assuming a Poisson distribution using the lme4 package of R (Bates et al. 2014). Interactions and main effects of pesticide treatments and living mulch treatments were tested, and site was included as a random effect. Annual sums of marketable zucchini fruits per plot were analyzed similarly, but because yield responses to our treatments varied extremely according to site-specific variables, we included ‘site’ as a fixed effect in this model, and tested a three-way interaction between site, living mulch treatments, and pesticide treatments. Pairwise contrasts were made for each model with Tukey posthoc tests using the multcomp package of R (Hothorn et al. 2016).
Reference cited. Hothorn, T., Bretz, F., Westfall, P., Heiberger, R.M., Schuetzenmeister, A., Scheibe, S., Hothorn, M.T., 2016. Package ‘multcomp.’ Simultaneous inference in general parametric models. Project for Statistical Computing, Vienna, Austria.
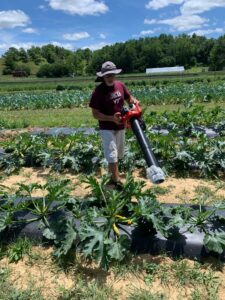
References cited:
Frank and Liburd. 2005. Effects of living and synthetic mulch on the population dynamics of whiteflies and aphids, their associated natural enemies, and insect transmitted plant diseases in zucchini. Environ. Entomol. 34:857-865.
Guner, N., Pesic-VanEsbroeck, Z., Rivera-Burgos, L. A., & Wehner, T. C. (2019). Screening for Resistance to Zucchini yellow mosaic virus in the Watermelon Germplasm. HortScience, 54(2), 206–211. https://doi.org/10.21273/HORTSCI13325-18
Hooks, C. R. R., Valenzuela, H. R., & Defrank, J. (1998). Incidence of pests and arthropod natural enemies in zucchini grown with living mulches. Agriculture, Ecosystems & Environment, 69(3), 217–231. https://doi.org/10.1016/S0167-8809(98)00110-8
Lee et al. 2001. Refuge habitats modify impact of insecticide disturbance on carabid beetle communities. J. Appl. Ecol. 38:472-483.
Objective 3. Assess the impact of augmentative releases of the egg parasitoid Hadronotus pennsylvanicus (formerly Gryon pennsylvanicum) on squash bug populations in squash.
Biological control of squash bugs is largely understudied, specifically the potential of its egg parasitoid, Hadronotus pennsylvanicus (Hymenoptera: Scelionidae), as an augmentative biological control agent.
Squash bug and egg parasitoid Gryon pennsylvanicum (Fig. 1) lab colonies were established from wild collected individuals in Whitethorne, VA during the 2019 field season. From August 2019 to August 2022, G. pennsylvanicum populations were reared on squash bug eggs and lab parasitism data were collected. Our lab rearing data focused on the wasp’s parasitism rates, progeny sex ratio, and development time (i.e., number of days from parasitism to emergence). These data were pivotal to determine how many parasitized eggs would need to be deployed at each release site in order to achieve a release rate of about 2-3 female wasps per plant.
For this reason, we performed field releases of H. pennsylvanicus on organic farms in Virginia as well as North Carolina and Georgia to test whether A. tristis egg parasitism would improve at parasitoid release sites. We chose organic farms growing summer squash as release sites and paired each site with a no-release site where no parasitoids were released. Parasitoids were reared in the lab and deployed as parasitized squash bug egg masses at a rate of 2-3 females wasps per plant in June 2020 and 2021. Following H. pennsylvanicus deployment, biweekly collections of squash bug eggs were conducted at release and non-release sites.
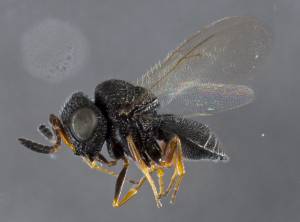
Objective 3b (Impacts of insecticides on squash bug and its parasitoid Hadronotus pennsylvanicus).
Growers can control squash bug by applying insecticides such as pyrethroids or neonicotinoids (Doughty et al. 2016). While these insecticides are effective in controlling squash bug (Eiben et al. 2004, Abney and Davila 2011, Doughty et al. 2016), they can have negative side effects on non-target organisms including pollinators and arthropod natural enemies (Smith and Stratton 1986, Slosser et al. 1989, Kilpatrick et al. 2005, Desneux et al. 2007). Thus, there is impetus for reduced risk alternatives to pyrethroids and neonicotinoids for control of squash bug.
Here we evaluated several reduced risk insecticides and one broad spectrum insecticide for their toxicity to A. tristis nymphs as well as Hadronotus pennsylvanicus (formerly Gryon pennsylvanicum) (Ashmead) (Hymenoptera: Scelionidae), a key parasitoid of squash bug. Treatments (Table 1) included the neonicotinoid acetamiprid (IRAC 2016, Group 4A), which has a lower toxicity to pollinators compared to other neonicotinoids (Decourtye and Devillers 2010); sulfoxaflor (IRAC 2016, Group 4C) and flupyradifurone (IRAC 2016, Group 4D), of which both act on the nicotinic acetylcholine receptor (Casida and Durkin 2013); three compounds pyrifluquinazon (IRAC 2016, Group 9), afidopyropen (IRAC 2016, Group 9D), and flonicamid (IRAC 2016, Group 29), which act on the chordotonal organ impacting the ability of hemipterans to feed (Morita et al. 2007; Nesterov et al. 2015)); and finally cyclaniliprole a broadspectrum diamide (IRAC 2016, Group 28). With the exception of cyclaniliprole, most of the aforementioned insecticides are generally used to target sucking hemipterans such as aphids, psyllids, and whiteflies, but which thorough information is lacking on their efficacy against squash bug. The pyrethroid, λ-cyhalothrin was tested as a commercial standard.
References cited
Abney, M. R., and R. Davila. 2011. Evaluation of foliar and soil applied insecticides for control of squash bug on zucchini squash, 2010. Arthrop. Manag. Tests 36 (1): E68 (DOI: http://dx.doi.org/10.4182/amt.2011.E68)
Casida, J. E., and K. A. Durkin. 2013. Neuroactive insecticides: targets, selectivity, resistance, and secondary effects. Annu. Rev. Entomol. 58: 99-117.
Chapman, A., T. Kuhar, P. Schultz, T. Leslie, S. Fleischer, G. Dively, and J. Whalen. 2009. Integrating Chemical and Biological Control of European Corn Borer in Bell Pepper. J. Econ. Entomol. 102: 287-295.
Decourtye, A. and J. Devillers. 2010. Ecotoxicity of neonicotinoid insecticides to bees. In: Thany, S.H. (ed) Insect Nicotinic Acetylcholine Receptors. Advances in Experimental Medicine and Biology, vol 683. Springer, New York, NY, pp 85–95. https://doi.org/10.1007/978-1-4419-6445-8_8
Desneux N, A. Decourtye, and J. M. Delpuech 2007. The sublethal effects of pesticides on beneficial arthropods. Annu. Rev. Entomol. 52: 81–106.
Doughty, H. B., J. M. Wilson, P. B. Schultz, and T. P. Kuhar. 2016. Squash bug (Hemiptera: Coreidae): biology and management in cucurbitaceous crops. J. Integr. Pest Manag. 7: 1.
Eiben, J., C. Mackey, W. Roberts, and J. V. Edelson. 2004. Foliar applied insecticides for controlling squash bug, 2003. Arthrop. Manag. Tests 29 (1): E74 (DOI: http://dx.doi.org/10.1093/amt/29.1.E74)
Fairbrother, A., J. Purdy, T. Anderson, and R. Fell. 2014. Risks of neonicotinoid insecticides to honeybees. Environ. Tox. Chem. / SETAC 33: 719-731.
Fargo, W. S., P. E. Rensner, E. L. Bonjour, and T. L. Wagner. 1988. Population dynamics in the squash bug (Heteroptera: Coreidae) squash plant (Cucurbitales: Cucurbitaceae) system in Oklahoma. J. Econ. Entomol. 81: 1073-1079.
Kilpatrick, A. L., A. M. Hagerty, S. G. Turnipseed, M. J. Sullivan, and W. C. J. Bridges. 2005. Activity of selected neonicotinoids and dicrotophos on nontarget arthropods in cotton: Implications in Insect Management. J. Econ. Entomol. 98: 814-820.
Kuhar, T. P., J. Speese, R. J. Cordero and V. M. Barlow. 2005. Evaluation of insecticides in pumpkins, 2004. Arthrop. Manag. Tests 30: E70.
Kuhar, T. P., and H. Doughty. 2016. Evaluation of Foliar and Soil Insecticides for the Control of Foliar Insects in Summer Squash in Virginia, 2015. Arthropod Management Tests 41: tsw023.
Morita, M., T. Ueda, T. Yoneda, T. Koyanagi, and T. Haga. 2007. Flonicamid, a novel insecticide with a rapid inhibitory effect on aphid feeding. Pest Manag. Sci. 63: 969-973.
Nauen, R., P. Jeschke, R. Velten, M. E. Beck, U. Ebbinghaus-Kintscher, W. Thielert, K. W⍤lfel, M. Haas, K. Kunz, and G. Raupach. 2015. Flupyradifurone; a brief profile of a new butenolide insecticide. Pest Manag. Sci. 71: 850-862.
Neal, J. J. 1993. Xylem transport interruption by Anasa tristis feeding causes Cucurbita pepo to wilt. Entomol. Exp. Appl. 69: 195-200.
Nesterov, A., C. Spalthoff, R. Kandasamy, R. Katana, N. B. Rankl, M. Andres, P. Jahde, J. A. Dorsch, L. F. Stam, F. J. Braun, B. Warren, V. L. Salgado, and M. C. Gopfert. 2015. TRP channels in insect stretch receptors as insecticide targets. Neuron 86: 665-671.
Pisa, L. W., V. Amaral-Rogers, L. P. Belzunces, J. M. Bonmatin, C. A. Downs, D. Goulson, D. P. Kreutzweiser, C. Krupke, M. Liess, M. McField, C. A. Morrissey, D. A. Noome, J. Settele, N. Simon-Delso, J. D. Stark, J. P. Van der Sluijs, H. Van Dyck, and M. Wiemers. 2014. Effects of neonicotinoids and fipronil on non-target invertebrates. Environ. Sci. Poll. Res. Int. 22: 68-102.
Slosser, E., W. E. Pinchak, and D. R. Rummel. 1989. A review of known and potential factors affecting the population dynamics of the cotton aphid. Southwest. Entomol. 14: 302-313.
Smith, T. M. and G. W. Stratton. 1986. Effects of synthetic pyrethroid insecticides on nontarget organisms. Residue Reviews: Reviews of Environmental Contamination and Toxicology: 93-120.
Wyenandt, A., T. P. Kuhar, G. C. Hamilton, M. J. VanGessel, E. Sanchez, and D. Dugan. 2016. 2016 Mid-Atlantic commercial vegetable production recommendations. Virginia Cooperative Extension. Publication #456-420.
Objective 4. We were unable to do the economic analysis for these studies.
Objective 1. Effects of colored plastic mulch on cucurbit production and insect communities.
Our research showed similar results as others with black and silvery reflective mulches having a beneficial impact on squash yield compared to bare ground beds in one of the years. The reasons for these differences are not completely known, but are likely related to increased soil temperature and moisture retention resulting in greater plant growth and productivity. However, greater densities of squash bugs were found on plastic mulch plots compared to bare ground beds. Squash bug adults like to seek shelter in the planting hole of plastic mulch and consequently may deposit more eggs on those plants. Our study offers new insights for cucurbit growers to use when considering whether they should implement plasticulture in their growing systems.
In our study, we observed significantly more A. tristis adults and egg masses on summer squash grown with white, black, or reflective mulch compared to bare ground, yet no differences in adult or egg mass numbers among the three mulch color treatments (Fig.1, 2). The observed similarity in population dynamics for adults and egg masses was expected, as the two life stages are highly correlated throughout much of the growing season (Harmon et al. 2003). More specifically, once adults reach peak density, there is a subsequent peak in egg mass density (Fargo et al. 1988; Palumbo et al. 1991b). Our findings also support a preference by A. tristis adults for squash grown in plastic mulched systems, which appears to be unaffected by mulch color. Preference for plastic mulch systems may be a result of A. tristis adults’ propensity to congregate near the crown and lower, older leaves of their host plant (Palumbo et al. 1991a). During the initial weeks of the summer squash vegetative growth phase, the plants are small and offer inadequate refuge for the colonizing adults. Holes in the plastic mulch where squash is planted provide shelter for adults to feed on the young plant’s main stem and mate relatively protected from natural enemies. One of the most prominent natural enemies of A. tristis adults, parasitoid fly Trichopoda pennipes Fabr. (Diptera: Tachnidae), actively searches for the bugs in the canopy of host plants (Beard 1940). However, it is unknown whether T. pennipes will forage for host bugs underneath the plastic mulch. Squash bug copulation can last > 20 h for a single adult pair, leaving the bugs vulnerable for extended periods of time (Sears et al. 2020). The protective cover of plastic mulch may then allow A. tristis to optimize its fitness and fecundity early in the season when shelter near host plants is sparse. Future research is needed to completely discern if squash bug adults experience decreased rates of predation and parasitism when using plastic mulch as refuge.
Nymphal densities were highly variable and did not follow the pattern of the adult and egg mass numbers. Mulch treatment was only a significant predictor of nymph counts in 2020 (Table 1; Fig. 2c). Previous research suggests that while all A. tristis life stages exhibit an aggregated distribution, nymphs show the greatest degree of aggregation (Palumbo et al. 1991c). For this reason, nymph distribution may be patchier and require additional sampling to accurately determine nymphal abundance (Palumbo et al. 1991c). Therefore, a potentially patchy distribution of nymphs in conjunction with identical numbers of plants surveyed for all life stages may explain inconsistent results among nymphs between and within treatment groups.
Even with significant differences in A. tristis adult and egg mass numbers between mulched and bare ground plots, differences among mulch treatments observed in marketable fruit yield varied between years, as plants in plastic mulch produced more marketable fruit than bare ground plants only in 2019 (Table 1, Fig. 1d, 2d). As mentioned earlier, the use of plastic mulch in vegetable systems provides numerous horticultural advantages (Jabran 2019). In cucurbit crops, mulch increases yield and plant biomass, lowers soil moisture evaporation, and improves irrigation efficiency (Conway et al. 1989, Kirnak and Demirtas 2006, Torres-Olivar et al. 2018). The benefits of plastic mulch may have had a compensatory effect in 2019, increasing yield to even exceed reductions resultant from increased squash bug pressure. Contrarily, even with reduced A. tristis pressure, bare ground zucchini produced similar marketable fruit yield to mulched plants in 2020, further suggesting a compensatory/beneficial effect of plastic mulch on yield. Disentangling relationships among insect pressure, plastic mulch, and yield has the added difficulty of research lacking a well-established relationship between squash bug pressure and yield. To date, research-based threshold values for adults, egg masses, and nymphs have not been firmly established. While we did find some differences in insect pressure, mulch, and marketable yield between treatments, our study did not include numbers of fruit discarded due to A. tristis damage. It is possible that mulched plants with large densities of squash bugs incurred greater fruit damage than those grown in bare ground with lower bug densities. In order to correctly assess yield loss from squash bug damage, additional fruit quality predictor variables like pollination success, presence/absence of microbial pathogens, and counts of other cucurbit insect pests are required. Future experimentation attempting to connect squash bug and its influence on damaged fruit numbers should include the aforementioned predictors to accurately elucidate how A. tristis specifically influences overall marketable yield in summer squash.
Despite many differences in methodology and experimental design, our study and Cartwright et al. (1990) identified similar patterns in squash bug presence and yield across different mulch treatments. Both studies observed more adults and egg masses on squash in plastic mulch versus bare soil plots, and inconsistent nymph presence among treatments between years. Although we demonstrated differences in yield between mulch treatments, methodological ambiguity in Cartwright et al. (1990) makes comparing yield between the two studies difficult. For example, they only recorded total yield in the second year of their study with no known differentiation between marketable and damaged fruit. Additionally, they offered no explanation of how or for what duration they conducted their yield collections and varied their squash planting dates and subsequent A. tristis sampling time frames dramatically between years. Overall, our study clarifies and expands the results described in Cartwright et al. (1990) by using uniform planting dates, increasing mulch treatment and sequential sampling replication, and including two years of marketable yield data.
When considering the implementation of plasticulture in summer squash production, growers should be mindful of the entire cucurbit pest complex, particularly which species are routinely economically destructive in their region. Despite the possible horticultural attributes provided by plastic mulch, our research conducted in southwest Virginia suggests plastic mulch can negatively impact squash bug management, especially in regions with high A. tristis pressure. Growers in such areas looking to minimize A. tristis presence may benefit from forgoing the use of plastic mulch, and instead, utilize a system that uses herbicide applications, cover crops, or other weed suppression tactics. On the other hand, growers in locations that experience significant pressure from cucumber beetles, aphids, or whiteflies can help protect their cucurbits from pest colonization with the repellency effects offered by reflective plastic mulch (Summers et al. 1995, Caldwell and Clark 1999, Vincent et al. 2003, Frank and Liburd 2005). Availability of essential farm equipment (e.g., plastic mulch layer) and added costs of specific plastic mulch colors (e.g., reflective, red, blue, etc.) will also inevitably impact whether a certain plasticulture system is implemented. In most cases, cucurbit growers will face a mixed assemblage of insect pests throughout the growing season, requiring them to conduct cost-benefit analyses of all possible cultural, biological, and chemical control strategies. Using the newly acquired knowledge put forth by this study, growers can more confidently decide if plasticulture fits within their squash bug management plans.
Publication produced:
Boyle, S. M., A. M. Alford, K. C. McIntyre, D. C. Weber, and T. P. Kuhar. 2022. Effect of plastic mulch colors on Anasa tristis (Hemiptera: Coreidae) population dynamics in summer squash (Cucurbita pepo). J. Econ. Entomol. 115(3): 808–813. https://doi.org/10.1093/jee/toac036
Objective 2. Harmonize chemical and biological control in cucurbit systems by integrating plasticulture with living mulches between beds
We predicted that living mulches and pesticides would have interactive effects on natural enemies, with negative pesticide effects being attenuated in plots with untreated living mulches serving as refugia between crop rows. Surprisingly, we found that living mulches instead magnified non-target effects by reducing natural enemy densities on pesticide-treated crops adjacent to living mulches, relative to bare plots. Pesticides had no effect on spotted and striped cucumber beetles, while living mulches directly reduced them by 25%. Conversely, pesticides reduced squash bug pressure by 50%, while living mulches had no effect. Although crops were grown in plastic mulch to protect them from competition, living mulches reduced zucchini yields by 54% at sites where living mulches were less-managed. Alternatively, living mulches had more neutral effects on yields at sites where mulches were mowed monthly, suggesting that living mulches require management to minimize competition with crops. These results suggest that the grass-dominated living mulches we tested did little to harmonize chemical and biological control. While non-crop plant diversity has clear benefits for natural pest suppression in many systems, these benefits cannot be generalized across all plant and insect taxa. Future efforts to fine-tune management of non-crop habitat within fields will be strengthened by consideration of traits of key pests and their specific responses to both pesticide applications and plant diversity.
Results
Natural enemies: In contrast to our prediction, living mulches did not mitigate pesticide-mediated reductions in natural enemies in the zucchini cash crop across our sites. We observed a significant interaction between living mulches and pesticides, but instead of increasing natural enemies where pesticides were applied, living mulches decreased them by 20% relative to untreated plots (significant pesticide * living mulch interaction, Fig. 1, Table 1a). Living mulches had no effect in the absence of pesticides, and neither living mulch nor pesticides had significant main effects on natural enemies generally (Table 1a).
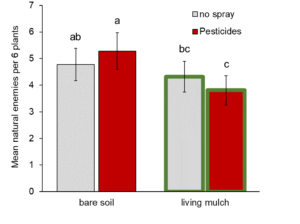
Key zucchini pests: Pesticide treatments had no impact on cucumber beetles, while living mulches reduced them by 25% (Fig. 2A, Table 1b). Pesticides reduced squash bugs by 50%, while living mulches subtly increased them (Fig 2B., Table 1c).
Zucchini yield: A significant three-way interaction between site, pesticide, and living mulch treatments revealed that site-specific management and environmental variables led to complex and varied effects of pesticides and living mulches (Fig. 4, Table 2). For example, living mulches led to strong yield losses at all sites but UGA, where they were mowed to keep living mulches less than 0.3 m tall to limit competition (Fig. 4). Sites with high squash bug pressure (Coastal and Inland Virginia, Appendix Fig.1) had decreased yield in the absence of pesticide applications (Fig. 4), while pesticides provided relatively limited yield benefits at sites where cucumber beetles dominated the herbivore community (Georgia and North Carolina).
Discussion
Pesticides are widely used to protect crops from arthropod pests, but can come at a cost of reducing natural enemy biodiversity (Stark et al. 2007; Mills et al., 2016). Integrating structurally and ecologically complex plants alongside crops might buffer natural enemies from collateral damage by providing refuge and augmenting food resources (Lee et al., 2001; Pozzebon et al., 2014; Gurr et al., 2017). Recent work in large-scale agronomic production reveals that intercropping can impose stronger control on pests and stronger benefits for natural enemies that outweigh effects of pesticide treatments (Alarcon-Segura 2022). By integrating living mulches with pesticide applications in zucchini crops, we expected to enhance natural enemies and partially mitigate harmful non-target effects. Instead, we discovered that living mulches backfired to magnify negative effects of pesticides on natural enemies, reducing them by 20% relative to pesticide-treated plots without living mulches (Fig. 1).
Chemical and biological control are notoriously difficult to implement concurrently (Roubos et al., 2014a; Schmidt-Jeffris et al., 2021). One factor that might have contributed to the failure of our living mulch to augment natural enemies was the composition and structure of the living mulch itself. At most sites, teff grass dominated the living mulch community (Fig S3); grasses provide few nectar/pollen resources (Ramsden et al. 2015; Toivonen et al., 2018) and may have formed a barrier to movement for many natural enemies, counteracting the expected benefits of rapid recolonization after pesticide treatments (Roubos et al., 2014a; Gontijo, 2019). In some scenarios, natural enemy numbers increase in living mulches but do not move nor provide any biological control services in the neighboring cash crops (Lopez and Liburd 2021). Therefore, our intercrops may have served as a sink, rather than a source of natural enemies in adjacent zucchini plants (Schellhorn et al. 2014).
A final potential explanation for why our non-crop habitat may have failed to ameliorate non-target effects of pesticides on natural enemies could be that dense living mulch vegetation could have slowed the residual deterioration of pesticides by blocking light and/or airflow between crop rows (Takahashi et al., 2017; Leach et al., 2017). Typically, organic pesticides like pyrethrins are short-lived, degrading within 24-48 hours (Barry et al., 2005), and recolonization by natural enemies increases greatly after pesticide residuals decline to relatively benign levels (Roubos et al., 2014b). However, if drying and photodegradation of pyrethrins were delayed by living mulches, this would extend residual activity and the window of pesticide vulnerability for natural enemies. Because predators are typically more susceptible pesticides than herbivores (Starks et al. 2007. Douglas et al., 2015; Tooker et al., 2020), our living mulches may have inadvertently exacerbated non-target effects on predators without improving control of pests.
Notably, the organic pesticide we applied had no impact on cucumber beetle pests across sites. Cucumber beetles are particularly difficult to control with insecticides due to the timing of their life cycle, as larvae are protected from pesticide exposure belowground, and highly mobile adults can emerge from soil in multiple waves (MacIntyre Allen et al., 2001; Andow et al., 2016). In our study, living mulch reduced cucumber beetle numbers by 25% compared with bare-soil plots, which may indicate that intercrops served as a barrier for dispersal and recolonization and/or impaired beetles’ ability to locate their host plants (Bach 1980, Holmes and Barrett, 1997). Indeed, chrysomelid pests are commonly found to decline in intercropped systems regardless of natural enemy pressure, although declines vary depending on species (Andow 1991; Hinds and Hooks 2013; Kahl et al., 2019). Resource-mediated herbivore declines caused by intercrops might also be attributed to reduction in suitable microclimate (Hinds and Hooks 2013), or decreased host suitability from companion plant competition (Iverson et al., 2014; Kahl et al., 2019).
In contrast to patterns observed with cucumber beetles, pesticides reduced squash bug numbers by 50% compared to unsprayed plots, while living mulches increased them slightly (Fig. 2b). Organic pesticides are sometimes inadequate in controlling squash bugs (Seaman et al., 2015), but our pyrethrin applications kept them below economic injury levels across all pesticide-treated plots (Doughty et al., 2016). In contrast to our prediction, squash bugs increased slightly in the presence of living mulches compared to bare plots, potentially because the mulches formed a structural barrier to movement of natural enemies between crop rows, or provided sufficient alternative prey resources that prevented their wide dispersal (Redlich et al., 2018; Fair and Braman, 2017). Additionally, the living mulch did not provide many floral resources that would typically attract parasitoids, a key natural enemy of squash bugs (Wilson and Kuhar, 2017; Cornelius et al., 2019). Both of the Virginia sites had high yield costs in the absence of pesticides, where squash bug pressure was relatively high, whereas pesticide applications in Georgia and North Carolina had relatively limited impacts on yield, where cucumber beetles were dominant (Fig. S2). Contrasting responses to living mulches and pesticide treatments between the two key pests of zucchini highlight the need to carefully consider unique life history traits (e.g. dispersal capacity, diet breadth) and differential patterns of susceptibility among dominant herbivores when making management decisions (Cornelius et al., 2019; Kahl et al., 2019). Recommendations for managing pests and non-crop biodiversity should not be generalized across wide geographic ranges, even in similar crop systems, because the dominant pest(s) can vary by location and region (Karp et al., 2018; Hooks et al., 1998).
At all sites except in Georgia, living mulches treatments led to yield losses of up to 72%. Living mulches were relatively unmanaged at North Carolina and Virginia sites and exceeded the canopy of the zucchini crops, causing competition for light, water, and nutrients (Gurr et al., 2017). Even where living mulches were actively managed with mowing at UGA, it is important to note that they did not increase yield, and even reduced it by 35% in pesticide-managed plots. These patterns highlight the need for active management of non-crop diversity in agroecosystems (Himanen et al., 2016), as well as a need to consider added labor costs in the deployment of living mulches (Huss et al., 2022). Economic benefits of biodiversity-based tools are highly variable across systems (Griffiths et al., 2008; Letourneau et al., 2011, Gurr et al. 2017). While ecological intensification can lead to increased and stable yields in the long-term, short-term financial incentives may be needed to facilitate wide-scale adoption and mitigate initial losses for farmers as they troubleshoot management (Rosa-Schleich et al., 2019). Economic interests of farmers are important to consider, as it is difficult to incentivize ecologically-based practices solely for the benefit of biodiversity without financial safety nets to offset potential costs (Bybee-Finley and Ryan, 2018).
Ultimately, our results emphasize the need to acknowledge and clarify where biodiversity might function to strengthen crop protection and where it might fail (Holmes and Blubaugh, in review, Tscharntke et al. 2017). While a rich body of evidence reveals pest control benefits of living mulches and intercrops in cucurbit agroecosystems (Hinds and Hooks, 2013; Hooks et al., 1998; Hooks and Wright, 2008), and in agroecosystems generally (Iverson et al., 2014; Albrecht et al., 2020, Alarcon-Segura 2022), integrating our living mulches in pesticide-treated crops failed to augment natural enemy services and incurred significant yield losses. Increased plant diversity in agroecosystems does not always lead to increased biological control (Gontijo et al., 2018; Karp et al., 2018; Lopez and Liburd, 2021), and our contrasting results with cucumber beetles and squash bugs in zucchini clarify how variability in non-crop habitat quality and unique pest characteristics contribute to context dependency in benefits of non-crop habitats in different environments (Griffiths et al., 2008).
Reducing the frequency and increasing the selectivity of pesticides (Bergeron and Schmidt-Jeffris 2020; Bilbo et al. 2022) and improving intercrop selection and management for our system may improve harmony between chemical and biological control, but our results reinforce that ‘silver bullet’ solutions are elusive in integrated pest management (Tooker et al., 2017; Koricheva and Hayes, 2018). Indeed, pests commonly diverge in their responses to biodiversity (Begum et al., 2006; Balzan et al., 2016, Alarcon-Segura 2022), and adopting practices effective against one pest may likely increase crop susceptibility to another (Simon et al., 2011; Blubaugh et al. 2021). Ecological interactions can be highly nuanced in biodiverse agroecosystems, and growers may benefit from making management decisions that reflect the unique life history traits of dominant pests on their farms. Future research must examine whether trait-informed management decisions improve the predictability of success for ecological pest management tools.
- References
Alarcón-Segura, V., Grass, I., Breustedt, G., Rohlfs, M., Tscharntke, T., 2022. Strip intercropping of wheat and oilseed rape enhances biodiversity and biological pest control in a conventionally managed farm scenario. J. Appl. Ecol. 59, 1513–1523. https://doi.org/10.1111/1365-2664.14161
Albrecht, M., Kleijn, D., Williams, N.M., Tschumi, M., Blaauw, B.R., Bommarco, R., Campbell, A.J., Dainese, M., Drummond, F.A., Entling, M.H., Ganser, D., Arjen de Groot, G., Goulson, D., Grab, H., Hamilton, H., Herzog, F., Isaacs, R., Jacot, K., Jeanneret, P., Jonsson, M., Knop, E., Kremen, C., Landis, D.A., Loeb, G.M., Marini, L., McKerchar, M., Morandin, L., Pfister, S.C., Potts, S.G., Rundlöf, M., Sardiñas, H., Sciligo, A., Thies, C., Tscharntke, T., Venturini, E., Veromann, E., Vollhardt, I.M.G., Wäckers, F., Ward, K., Westbury, D.B., Wilby, A., Woltz, M., Wratten, S., Sutter, L., 2020. The effectiveness of flower strips and hedgerows on pest control, pollination services and crop yield: a quantitative synthesis. Ecol. Lett. 23, 1488–1498. https://doi.org/10.1111/ele.13576
Andow, D.A. 1991. Vegetational diversity and arthropod population response. Annu. Rev. Entomol, 36, 561-6.
Andow, D.A., Pueppke, S.G., Schaafsma, A.W., Gassmann, A.J., Sappington, T.W., Meinke, L.J., Mitchell, P.D., Hurley, T.M., Hellmich, R.L., Porter, R.P., 2016. Early detection and mitigation of resistance to Bt maize by western corn rootworm (Coleoptera: Chrysomelidae). J. Econ. Entomol. 109, 1–12. https://doi.org/10.1093/jee/tov238
Bach, C.E., 1980. Effects of plant density and diversity on the population dynamics of a specialist herbivore, the striped cucumber beetle, Acalymma vittata (Fab). Ecol., 61, 1515-1530.
Balzan, M.V., Bocci, G., Moonen, A.-C., 2016. Utilisation of plant functional diversity in wildflower strips for the delivery of multiple agroecosystem services. Entomol. Exp. Appl. 158, 304–319. https://doi.org/10.1111/eea.12403
Barry, J.D., Sciarappa, W.J., Teixeira, L.A., Polavarapu, S., 2005. Comparative effectiveness of different insecticides for organic management of blueberry maggot (Diptera: Tephritidae). J. Econ. Entomol., 98, 1236-1241.
Bates, D., Mächler, M., Bolker, B., Walker, S., 2014. Fitting linear mixed-effects models using lme4. arXiv preprint arXiv:1406.5823.
Begum, M., Gurr, G.M., Wratten, S.D., Hedberg, P.R., Nicol, H.I., 2006. Using selective food plants to maximize biological control of vineyard pests. J. Appl. Ecol. 43, 547–554. https://doi.org/10.1111/j.1365-2664.2006.01168.x
Bergeron, P.E., Schmidt‐Jeffris, R.A., 2020. Not all predators are equal: miticide non‐target effects and differential selectivity. Pest Manag. Sci., 76, 2170-2179.
Bilbo, T.R., Owens, D.R., Golec, J.R., Walgenbach, J.F., 2022. Impact of insecticide programs on pests, the predatory mite Phytoseiulus persimilis, and staked tomato profitability. Pest Manage. Sci. 78, 2390–2397
Blubaugh, C.K., Carpenter-Boggs, L., Reganold, J.P., Snyder, W.E., 2021. Herbivore-herbivore interactions complicate links between soil fertility and pest resistance. Basic Appl. Ecol., 52, 57-67.
Bommarco, R., Kleijn, D., Potts, S.G., 2013. Ecological intensification: harnessing ecosystem services for food security. Trends Ecol. Evol. 28, 230–238. https://doi.org/10.1016/j.tree.2012.10.012
Bybee-Finley, K.A., Ryan, M.R., 2018. Advancing intercropping research and practices in industrialized agricultural landscapes. Agric. 8, 80. https://doi.org/10.3390/agriculture8060080
Cornelius, M.L., Vinyard, B.T., Gates, M.W., 2019. Use of flowering plants to enhance parasitism and predation rates on two squash bug species Anasa tristis and Anasa armigera (Hemiptera: Coreidae). Insects 10, 318. https://doi.org/10.3390/insects10100318
Doughty, H.B., Wilson, J.M., Schultz, P.B., Kuhar, T.P., 2016. Squash bug (Hemiptera: Coreidae): Biology and management in cucurbitaceous crops. J. Integr. Pest Manag. 7, 1. https://doi.org/10.1093/jipm/pmv024
Douglas, M.R., Rohr, J.R., Tooker, J.F., 2015. Neonicotinoid insecticide travels through a soil food chain, disrupting biological control of non-target pests and decreasing soya bean yield. J. Appl. Ecol. 52, 250–260. https://doi.org/10.1111/1365-2664.12372
Fair, C.G., Braman, S.K., 2017. Assessment of habitat modification and varied planting dates to enhance potential natural enemies of Anasa tristis (Hemiptera: Coreidae) in squash. Environ. Entomol. 46, 291–298. https://doi.org/10.1093/ee/nvx043
Geiger, F., Bengtsson, J., Berendse, F., Weisser, W.W., Emmerson, M., Morales, M.B., Ceryngier, P., Liira, J., Tscharntke, T., Winqvist, C., Eggers, S., Bommarco, R., Pärt, T., Bretagnolle, V., Plantegenest, M., Clement, L.W., Dennis, C., Palmer, C., Oñate, J.J., Guerrero, I., Hawro, V., Aavik, T., Thies, C., Flohre, A., Hänke, S., Fischer, C., Goedhart, P.W., Inchausti, P., 2010. Persistent negative effects of pesticides on biodiversity and biological control potential on European farmland. Basic Appl. Ecol. 11, 97–105. https://doi.org/10.1016/j.baae.2009.12.001
Gontijo, L.M., Saldanha, A.V., Souza, D.R., Viana, R.S., Bordin, B.C., Antonio, A.C., 2018. Intercropping hampers the nocturnal biological control of aphids. Ann. Appl. Biol. 172, 148–159. https://doi.org/10.1111/aab.12407
Gontijo, L.M., 2019. Engineering natural enemy shelters to enhance conservation biological control in field crops. Biol. Contr. 9.
Griffiths, G.J.K., Holland, J.M., Bailey, A., Thomas, M.B., 2008. Efficacy and economics of shelter habitats for conservation biological control. Biol. Control, Conservation Biol. Contr. 45, 200–209. https://doi.org/10.1016/j.biocontrol.2007.09.002
Gurr, G. M., Z. Lu, X. Zheng, H. Xu, P. Zhu, G. Chen, X. Yao, J. Cheng, Z. Zhu, J. L. Catindig, S. Villareal, H. Van Chien, L. Q. Cuong, C. Channoo, N. Chengwattana, L. P. Lan, L. H. Hai, J. Chaiwong, H. I. Nicol, D. J. Perovic, S. D. Wratten, and K. L. Heong. 2016. Multi-country evidence that crop diversification promotes ecological intensification of agriculture. Nature Plants. 2: 16014.
Gurr, G.M., Wratten, S.D., Landis, D.A., You, M., 2017. Habitat management to suppress pest populations: Progress and prospects. Annu. Rev. Entomol. 62, 91–109. https://doi.org/10.1146/annurev-ento-031616-035050
Haan, N.L., Iuliano, B.G., Gratton, C., Landis, D.A., 2021. Designing agricultural landscapes for arthropod-based ecosystem services in North America, in: Advances in Ecological Research. Elsevier, pp. 191–250. https://doi.org/10.1016/bs.aecr.2021.01.003
Himanen, S.J., Mäkinen, H., Rimhanen, K., Savikko, R., 2016. Engaging farmers in climate change adaptation planning: Assessing intercropping as a means to support farm adaptive capacity. Agric. 6, 34. https://doi.org/10.3390/agriculture6030034
Hinds, J., Hooks, C.R.R., 2013. Population dynamics of arthropods in a sunn-hemp zucchini interplanting system. Crop Prot. 53, 6–12. https://doi.org/10.1016/j.cropro.2013.06.003
Holmes, D.M., Barrett, G.W., 1997. Japanese beetle (Popillia japonica) dispersal behavior in intercropped vs. monoculture soybean agroecosystems. Am. Midl. Nat. 137, 312–319. https://doi.org/10.2307/2426850
Hooks, C.R., Wright, M.G., 2008. Use of living and dying mulches as barriers to protect zucchini from insect-caused viruses and phytotoxemias. Plant Dis., 1-8.
Hooks, C.R.R., Valenzuela, H.R., Defrank, J., 1998. Incidence of pests and arthropod natural enemies in zucchini grown with living mulches. Agric. Ecosyst. Environ. 69, 217–231. https://doi.org/10.1016/S0167-8809(98)00110-8
Hothorn, T., Bretz, F., Westfall, P., Heiberger, R.M., Schuetzenmeister, A., Scheibe, S., Hothorn, M.T., 2016. Package ‘multcomp.’ Simultaneous inference in general parametric models. Project for Statistical Computing, Vienna, Austria.
Huss, C.P., Holmes, K.D., Blubaugh, C.K., 2022. Benefits and risks of intercropping for crop resilience and pest management. J. Econ. Entomol. 115, 1350–1362. https://doi.org/10.1093/jee/toac045
Iverson, A.L., Marín, L.E., Ennis, K.K., Gonthier, D.J., Connor-Barrie, B.T., Remfert, J.L., Cardinale, B.J., Perfecto, I., 2014. REVIEW: Do polycultures promote win-wins or trade-offs in agricultural ecosystem services? A meta-analysis. J. Appl. Ecol. 51, 1593–1602. https://doi.org/10.1111/1365-2664.12334
Kahl, H.M., Leslie, A.W., Hooks, C.R.R., 2019. Effects of red clover living mulch on arthropod herbivores and natural enemies, and cucumber yield. Ann. Entomol. Soc. Am. 112, 356–364. https://doi.org/10.1093/aesa/say036
Karp, D.S., Chaplin-Kramer, R., Meehan, T.D., Martin, E.A., DeClerck, F., Grab, H., Gratton, C., Hunt, L., Larsen, A.E., Martínez-Salinas, A., O’Rourke, M.E., Rusch, A., Poveda, K., Jonsson, M., Rosenheim, J.A., Schellhorn, N.A., Tscharntke, T., Wratten, S.D., Zhang, W., Iverson, A.L., Adler, L.S., Albrecht, M., Alignier, A., Angelella, G.M., Zubair Anjum, M., Avelino, J., Batáry, P., Baveco, J.M., Bianchi, F.J.J.A., Birkhofer, K., Bohnenblust, E.W., Bommarco, R., Brewer, M.J., Caballero-López, B., Carrière, Y., Carvalheiro, L.G., Cayuela, L., Centrella, M., Ćetković, A., Henri, D.C., Chabert, A., Costamagna, A.C., De la Mora, A., de Kraker, J., Desneux, N., Diehl, E., Diekötter, T., Dormann, C.F., Eckberg, J.O., Entling, M.H., Fiedler, D., Franck, P., Frank van Veen, F.J., Frank, T., Gagic, V., Garratt, M.P.D., Getachew, A., Gonthier, D.J., Goodell, P.B., Graziosi, I., Groves, R.L., Gurr, G.M., Hajian-Forooshani, Z., Heimpel, G.E., Herrmann, J.D., Huseth, A.S., Inclán, D.J., Ingrao, A.J., Iv, P., Jacot, K., Johnson, G.A., Jones, L., Kaiser, M., Kaser, J.M., Keasar, T., Kim, T.N., Kishinevsky, M., Landis, D.A., Lavandero, B., Lavigne, C., Le Ralec, A., Lemessa, D., Letourneau, D.K., Liere, H., Lu, Y., Lubin, Y., Luttermoser, T., Maas, B., Mace, K., Madeira, F., Mader, V., Cortesero, A.M., Marini, L., Martinez, E., Martinson, H.M., Menozzi, P., Mitchell, M.G.E., Miyashita, T., Molina, G.A.R., Molina-Montenegro, M.A., O’Neal, M.E., Opatovsky, I., Ortiz-Martinez, S., Nash, M., Östman, Ö., Ouin, A., Pak, D., Paredes, D., Parsa, S., Parry, H., Perez-Alvarez, R., Perović, D.J., Peterson, J.A., Petit, S., Philpott, S.M., Plantegenest, M., Plećaš, M., Pluess, T., Pons, X., Potts, S.G., Pywell, R.F., Ragsdale, D.W., Rand, T.A., Raymond, L., Ricci, B., Sargent, C., Sarthou, J.-P., Saulais, J., Schäckermann, J., Schmidt, N.P., Schneider, G., Schüepp, C., Sivakoff, F.S., Smith, H.G., Stack Whitney, K., Stutz, S., Szendrei, Z., Takada, M.B., Taki, H., Tamburini, G., Thomson, L.J., Tricault, Y., Tsafack, N., Tschumi, M., Valantin-Morison, M., Van Trinh, M., van der Werf, W., Vierling, K.T., Werling, B.P., Wickens, J.B., Wickens, V.J., Woodcock, B.A., Wyckhuys, K., Xiao, H., Yasuda, M., Yoshioka, A., Zou, Y., 2018. Crop pests and predators exhibit inconsistent responses to surrounding landscape composition. Proc. Natl. Acad. Sci. 115. https://doi.org/10.1073/pnas.1800042115
Kleijn, D., Bommarco, R., Fijen, T.P.M., Garibaldi, L.A., Potts, S.G., van der Putten, W.H., 2019. Ecological Intensification: Bridging the Gap between Science and Practice. Trends in Ecology & Evolution 34, 154–166. https://doi.org/10.1016/j.tree.2018.11.002
Koricheva, J., Hayes, D., 2018. The relative importance of plant intraspecific diversity in structuring arthropod communities: A meta-analysis. Funct. Ecol. 32, 1704–1717. https://doi.org/10.1111/1365-2435.13062
Landis, D., Wratten, S., Gurr, G., 2000. Habitat management to conserve natural enemies of arthropod pests in agriculture. Annu. Rev. Entomol. 45, 175–201. https://doi.org/10.1146/annurev.ento.45.1.175
Leach, H., Wise, J.C., Isaacs, R., 2017. Reduced ultraviolet light transmission increases insecticide longevity in protected culture raspberry production. Chemosphere 189, 454–465. https://doi.org/10.1016/j.chemosphere.2017.09.086
Lee, J.C., Menalled, F.D., Landis, D.A., 2001. Refuge habitats modify impact of insecticide disturbance on carabid beetle communities. J. Appl. Ecol. 38, 472–483. https://doi.org/10.1046/j.1365-2664.2001.00602.x
Letourneau, D.K., Armbrecht, I., Rivera, B.S., Lerma, J.M., Carmona, E.J., Daza, M.C., Escobar, S., Galindo, V., Gutiérrez, C., López, S.D., Mejía, J.L., Rangel, A.M.A., Rangel, J.H., Rivera, L., Saavedra, C.A., Torres, A.M., Trujillo, A.R., 2011. Does plant diversity benefit agroecosystems? A synthetic review. Ecol. Appl. 21, 9–21. https://doi.org/10.1890/09-2026.1
Lopez L., Liburd, O.E., 2021. Can the introduction of companion plants increase biological control services of key pests in organic squash? Entomol. Exp. Appl. 170: 402- 418. https://doi.org/10.1111/eea.13147
Mac Intyre Allen, J.K., Scott-dupree, C.D., Tolman, J.H., Harris, C.R., 2001. Evaluation of application methods for the chemical control of striped cucumber beetle (Coleoptera: Chrysomelidae) attacking seedling cucurbits. J. Veg. Crop Prod. 7, 83–95. https://doi.org/10.1300/J068v07n02_09
Matson, P.A., Parton, W.J., Power, A.G., Swift, M.J., 1997. Agricultural intensification and ecosystem properties. Sci. 277, 504–509. https://doi.org/10.1126/science.277.5325.504
Mills, N.J., Beers, E.H., Shearer, P.W., Unruh, T.R., Amarasekare, K.G., 2016. Comparative analysis of pesticide effects on natural enemies in western orchards: A synthesis of laboratory bioassay data. Biol. Control, Enhancing biological control in western orchards: a five-year project 102, 17–25. https://doi.org/10.1016/j.biocontrol.2015.05.006
Mpanga, I.K., n.d. Cultivation of Mixed Summer Cover Crops 6.
Olivier, T., Thébault, E., Elias, M., Fontaine, B., Fontaine, C. 2020. Urbanization and agricultural intensification destabilize animal communities differently than diversity loss. Nature Communications, 11, 1-9.
Pozzebon, A., Ahmad, S., Tirello, P., Lorenzon, M., Duso, C., 2014. Does pollen availability mitigate the impact of pesticides on generalist predatory mites? BioControl 59, 585–596. https://doi.org/10.1007/s10526-014-9598-3
Ramsden, M. W., Menéndez, R., Leather, S. R., Wäckers, F., 2015. Optimizing field margins for biocontrol services: the relative role of aphid abundance, annual floral resources, and overwinter habitat in enhancing aphid natural enemies. Agric., Ecosyst. Environ., 199, 94-104.
Redlich, S., Martin, E.A., Steffan-Dewenter, I., 2018. Landscape-level crop diversity benefits biological pest control. J. Appl. Ecol. 55, 2419–2428. https://doi.org/10.1111/1365-2664.13126
Rosa-Schleich, J., Loos, J., Mußhoff, O., Tscharntke, T., 2019. Ecological-economic trade-offs of diversified farming systems – A review. Ecol. Econ. 160, 251–263. https://doi.org/10.1016/j.ecolecon.2019.03.002
Roubos, C.R., Rodriguez-Saona, C., Holdcraft, R., Mason, K.S., Isaacs, R., 2014a. Relative toxicity and residual activity of insecticides used in blueberry pest management: Mortality of natural enemies. J. Econ. Entomol. 107, 277–285. https://doi.org/10.1603/EC13191
Roubos, C.R., Rodriguez-Saona, C., Isaacs, R., 2014b. Mitigating the effects of insecticides on arthropod biological control at field and landscape scales. Biol. Contr. 75, 28–38. https://doi.org/10.1016/j.biocontrol.2014.01.006
Rusch, A., Chaplin-Kramer, R., Gardiner, M.M., Hawro, V., Holland, J., Landis, D., Thies, C., Tscharntke, T., Weisser, W.W., Winqvist, C., Woltz, M., Bommarco, R., 2016. Agricultural landscape simplification reduces natural pest control: A quantitative synthesis. Agric. Ecosyst. Environ. 221, 198–204. https://doi.org/10.1016/j.agee.2016.01.039
Schmidt‐Jeffris, R.A., Beers, E.H., Sater, C., 2021. Meta‐analysis and review of pesticide non‐target effects on phytoseiids, key biological control agents. Pest Manage. Sci., 77, 4848-4862.
Seaman, A., Lange, H., Shelton, A., 2015. Squash bug and striped cucumber beetle control with insecticides allowed for organic production, 2014. Arthrop. Manag. Tests 40, E49. https://doi.org/10.1093/amt/tsv105
Simon, S., Bouvier, J.-C., Debras, J.-F., Sauphanor, B., 2011. Biodiversity and pest management in orchard systems, in: Lichtfouse, E., Hamelin, M., Navarrete, M., Debaeke, P. (Eds.), Sustainable Agriculture Volume 2. Springer Netherlands, Dordrecht, pp. 693–709. https://doi.org/10.1007/978-94-007-0394-0_30
Stark, J.D., Banks, J.E., 2003. Population-level effects of pesticides and other toxicants on arthropods. Annu. Rev. Entomol. 48, 505-519.
Stark, J. D., Vargas, R., Banks, J. E. 2007. Incorporating ecologically relevant measures of pesticide effect for estimating the compatibility of pesticides and biocontrol agents. J. Econ. Entomol., 100, 1027-1032.
Takahashi, D., Yamanaka, T., Sudo, M., Andow, D.A., 2017. Is a larger refuge always better? Dispersal and dose in pesticide resistance evolution. Evol. 71, 1494–1503. https://doi.org/10.1111/evo.13255
Tooker, J.F., Douglas, M.R., Krupke, C.H., 2017. Neonicotinoid seed treatments: Limitations and compatibility with integrated pest management. Agric. Environ. Lett. 2, ael2017.08.0026. https://doi.org/10.2134/ael2017.08.0026
Tooker, J.F., O’Neal, M.E., Rodriguez-Saona, C., 2020. Balancing disturbance and conservation in agroecosystems to improve biological control. Annu. Rev. Entomol. 65, 81–100. https://doi.org/10.1146/annurev-ento-011019-025143
Tscharntke, T., Karp, D.S., Chaplin-Kramer, R., Batáry, P., DeClerck, F., Gratton, C., Zhang, W. 2016. When natural habitat fails to enhance biological pest control–Five hypotheses. Biol. Conserv., 204, 449-458.
van den Bosch, R., Messenger, P.S., Gutierrez, A.P., 1982. The history and development of biological control, in: van den Bosch, R., Messenger, P.S., Gutierrez, A.P. (Eds.), An Introduction to Biological Control. Springer US, Boston, MA, pp. 21–36. https://doi.org/10.1007/978-1-4757-9162-4_3
Wilson, J.M., Kuhar, T.P., 2017. A survey of the species of squash bug (Hemiptera: Coreidae) egg parasitoids in Virginia and their distribution. J. Econ. Entomol. 110, 2727–2730. https://doi.org/10.1093/jee/tox276
Results were presented in 2022 at the International IPM symposium. We also published one review article (Huss, Holmes, and Blubaugh 2022) on the risks and benefits of living mulch intercrops in the Journal of Economic Entomology.
Publication:
Huss, C.P. , K.D. Holmes, and C.K. Blubaugh. 2022. Benefits and risks of intercropping for crop resilience and pest management. Journal of Economic Entomology. toac045, https://doi.org/10.1093/jee/toac045
Hudson, TB, AM Alford, TR Bilbo, SC Boyle, TP Kuhar, L Lopez, AK Stawara, KC McIntyre, JF Walgenbach, C Walls, C Blubaugh. 2023. Can living mulches harmonize chemical and biological control? Submitted to Agriculture, Ecosystems and Environment. Accepted with revisions
Symposium Presentation:
Blubaugh, C., A. Stawara, T. Kuhar, J. Walgenbach, T. Bilbo, H. Doughty, L. Lopez, C. Walls, S. Boyle, A. Alford. 2022. Can living mulches buffer natural enemies from non-target effects of pesticides? IPM 2022: 10TH INTERNATIONAL IPM SYMPOSIUM, February 28–March 3, 2022, Denver, Colorado.
Objective 3. Assess the impact of augmentative releases of the egg parasitoid Hadronotus pennsylvanicus (formerly Gryon pennsylvanicum) on squash bug populations in squash.
In both years, we found greater parasitism rates of A. tristis eggs collected at Virginia release sites compared to no-release sites. While all eggs collected at release and no-release sites before parasitoid deployment displayed low levels of H. pennsylvanicus parasitism, eggs from release sites were significantly more parasitized than eggs from no-release sites within two weeks post-deployment.
Table: Ratio of parasitized: unparasitized A. tristis egg masses collected at each release and no-release site in 2020. Combined ratios (bold values) were compared between release and no release treatments (Fishers Exact Test of Independence). Asterisks indicate significantly larger parasitized: unparasitized egg mass ratio (*P < 0.01, *** P < 0.0001) per sample date.
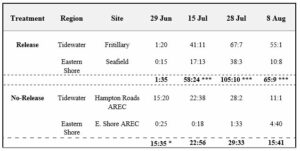
Our two-year study demonstrates that the releases of lab-reared H. pennsylvanicus can increase A. tristis egg parasitism rates and subsequently decrease successful nymph hatch rates in early summer squash plantings.
Objective 3b (Impacts of insecticides on squash bug and its parasitoid Hadronotus pennsylvanicus).
Pyrethroids or neonicotinoids have been the insecticides of choice for chemical control of squash bug for the past three decades (Doughty et al. 2016, Wyenandt et al. 2016). Although these insecticides are efficacious at controlling squash bug nymphs (Eiben et al. 2004, Kuhar et al. 2005, Abney and Davila 2011, Kuhar and Doughty 2016), most are also toxic to non-target arthropods and are generally not compatible with IPM or pollinator protection plans (Smith and Stratton 1986, Fairbrother et al. 2014, Pisa et al. 2014). In this study, acetamiprid, lambda-cyhalothrin, sulfoxaflor, and flupyradifurone all provided effective control of squash bug nymphs in lab bioassays. These insecticides have also resulted in significant reductions in squash bug densities in field experiments (Kuhar and Doughty 2016).
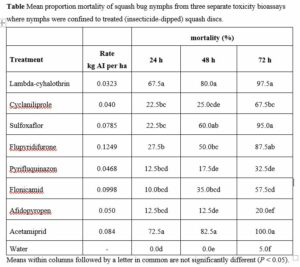
Acetamiprid, sulfoxaflor, and flupyradifurone are considered less toxic to pollinators than pyrethroids, while also providing control of aphids, whiteflies, and other small piercing-sucking pests. Repeated applications of pyrethroids, on the other hand, often lead to outbreaks of aphids by suppressing natural enemy abundance and promoting insecticide resistance (Slosser et al. 1989, Chapman et al. 2009).
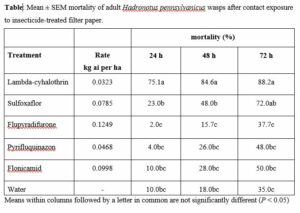
With regards to insecticide impact on the parasitoid H. pennsylvanicus, lambda-cyhalothrin and sulfoxaflor caused the highest mortality of adult wasps compared to flupyradifurone, pyrifluquinazon, and flonicamid. High rates of H. pennsylvanicus mortality in the lambda-cyhalothrin treatment was not surprising, as lethal and non-lethal effects have been observed with other scelionid egg parasitoid species (Salerno et al. 2002, Bayram et al. 2010). It was surprising to observe high mortality rates for adult parasitoids exposed to sulfloxaflor since it is absorbed systemically by plant tissues and acts on sucking pests that imbibe the compound when feeding on treated plant fluids (Sparks et al. 2013). The H. pennsylvanicus adults were exposed to all treatments via contact by walking on treated filter papers. There is recent evidence that supports this result, as detrimental effects of contact exposure to sulfoxaflor were found with three distinct Trichogramma parasitoid species (Jiang et al. 2019). Future research should add field-related complexity to laboratory assays, such as different insecticide application methods for whole C. pepo plants (e.g., foliar, chemigation, seed treatments), to elucidate more realistic insecticide exposure effects on H. pennsylvanicus adults.
Based on our bioassays, flupyradifurone was the only reduced risk insecticide found to be both highly toxic to A. tristis nymphs and nontoxic to H. pennsylvanicus adults. Our laboratory bioassays align with results from field trials displaying significant reductions in A. tristis abundance on summer squash plants sprayed with flupyradifurone (Kuhar and Doughty 2018). Although no previous research has studied the effects of flupyradifurone exposure on scelionid parasitoids, Tabebordbar et al. 2020 showed that parasitoid Trichogramma evanescens has reduced lethal and sub-lethal effects from contact exposure to field rates of the flupyradifurone compared to pyrethroid deltamethrin exposure. Additional studies are needed to identify if there are sub-lethal behavioral and life history effects of insecticide exposure on H. pennsylvanicus. In particular, research focused on determining potential effects on H. pennsylvanicus ability to successfully search for and locate A. tristis egg masses, as well as on parasitoid fecundity and longevity, would provide valuable information concerning the overall risk broad and narrow spectrum insecticides pose to H. pennsylvanicus.
Education
Three graduate students are being trained under this grant project:
Ph.D. student, Sean Boyle, Department of Entomology, Virginia Tech - began his program in 2019.
M.S. student, Courtney Walls, Department of Entomology, Virginia Tech - began her program in 2020.
M.S. student, Allison Stawara, Department of Entomology, University of Georgia - began her program in 2021.
Educational & Outreach Activities
Participation Summary:
Wilson, James M.; Day, Eric; Kuhar, Thomas P. 2000. Striped Cucumber Beetle. Virginia Cooperative Extension Publication No. AEE-72. https://www.pubs.ext.vt.edu/content/dam/pubs_ext_vt_edu/ENTO/ENTO-61/ENTO-61-pdf.pdf
Presentations:
Boyle, Sean, Thomas Kuhar, and Donald Weber. 2000. Reevaluating thresholds for squash bug, Anasa tristis, life stages in summer squash (Cucurbita pepo) systems2020 Entomology Virtual Meeting: Annual Meeting of the Entomological Society of America, November 11-25, 2020, Orlando, FL - Virtual.
Walls, Courtney, James Wilson, and Thomas Kuhar. 2020. What’s the buzz in Virginia cucurbits: Three trapping methods to survey pollinators. 2020 Entomology Virtual Meeting: Annual Meeting of the Entomological Society of America, November 11-25, 2020, Orlando, FL - Virtual.
Walls, Courtney. 2020. Beekeepers at Virginia Tech Virtual Meeting,– “Pollinators you can find in Virginia Cucurbits” October 27, 2020 – Virtual - Attendance: 25
Walls, Courtney, James Wilson, and Thomas Kuhar. 2021. Virginia Pumpkin Growers Association– “ What we found in Virginia Pumpkins” February 9, 2021- Virtual - Attendance: 30
Walls, Courtney. 2021. "Ask an Entomologist Virtual Field Trip", North Wilkes Middle School (Wilkes Country Schools, NC),– “Bees around you” – March 18, 2021 -Virtual -Audience: 100+
Kuhar, T. 2000. Insect pest update. Cucurbit Crops Session: Southeast Virginia Fruit and Vegetable Conference, Chesapeake, VA, Feb 27, 2000.
Kuhar, T. 2000. Vegetable pest IPM info in virtual Zoom Meeting on Vegetable Gardening Resources – VCE, Virginia Beach, VA, March 27 – 40 people
Kuhar, T. 2000. Vegetable IPM update. VCE Ag Today meeting focused on vegetable production, May 7 – 40 people
Kuhar, T. 2000. Insect pest control. IPM Ask the Expert – Teleconference, Radio show – Mid-Atlantic U.S., May 27 – 50 people
Kuhar, T. P. and D. Owens. 2020. Seasonal Insect Management for Vegetable Crops: Protecting Beneficial Insects While Minimizing Insect That Damage Vegetable Crops. 2020 Virtual Vegetable Grower Meetings, Virginia Coop. Ext., June 24, 2020.
Kuhar, T. 2000. Vegetable IPM update. Northern Virginia Virtual Vegetable Meeting, June 24, 40 people
Kuhar, T. P. 2020. Update on insect management. LATE SEASON Virtual PUMPKIN MEETING, Virginia Pumpkin Growers Association, S E P T EMB E R 1 6, 2020.
Kuhar, T. P. 2020. Fall Vegetable Pest Updates, VCE AG Today Virtual Meeting, October, 29, 2020.
Kuhar, T. P. 2020. Fall Vegetable Pest Updates, Shenandoah Valley Vegetable Grower Virtual Meeting, October, 29, 2020.
Kuhar, Thomas. 2020. Pest Management Update for Cucurbits. Northern Neck Vegetable Growers Association Meeting, Virtual. https://bit.ly/2020NNVGACucurbits
Kuhar, T., J. Wilson, and C. Walls. 2021. Insect control and pollinators. Virginia Pumpkin Growers Association 2021 Annual Meetings. Virtual. Hillsville, VA. Feb 9, 2021.
Virginia Biological Farming Virtual Conference. March 18, 2021
Kuhar, T. 2001. Organic options for battling insect pests. Organic Production Agent In-service Training. Roanoke, VA. March 24 & 25, 2021. Virtual.
Kuhar, T. 2021. Managing insect pests. Vegetable Crop Production for Urban Agriculture. Virginia Cooperative Extension In-Service Agent Training, March 2 and 3, 2021. Virtual.
Alford, A. 2020. Demonstrated colored mulch research plots. Southwest Minnesota State University Field Day. Marshall, MN. Aug 5, 2020.
Alford, A. 2020. Rotary club presentation of the experimental system/results of 2020 study. Marshall, MN. Oct 23rd 2020.
Learning Outcomes
Knowledge of importance of squash bug as a pest.
Knowledge of effects of different colored plastic mulches on yield and potentially pests such as squash bug.
Awareness of Hadronotus pennsylvanicum as an important biological control agent that should be preserved.
Knowledge of living mulches as an option for between plastic rows, but also the varying effects on the cropping system.
Knowledge of more selective insecticides that control squash bug with minimal impact on the parasitoid.
Project Outcomes
After evaluating squash and cucumbers grown on four different mulch treatments (silver reflective, white plastic, black plastic, bare ground) at two locations in 2019 and three locations in 2020, we found a significant effect of mulch treatment on yield of squash and cucumbers with black and silvery reflective mulch tending to yield significantly more fruit. However, greater densities of squash bugs were found on the plastic mulch plots compared with bare ground. These results suggest that in addition to aiding in weed suppression, colored plastic mulches positively impact crop yield of cucurbits, but also may result in greater squash bug populations. This information can aid in the decision making for growers to invest in the cost of plastic mulch for their cucurbit production.
In another experiment, the squash bug parasioid, Gryon pennsylvanicum was reared in Blacksburg Virginia and augmentative releases were made on two farms in eastern Virginia, while two nearby farms included as non-release controls. Parasitism of squash bug egg masses was increased significantly at the release sites. While more data are needed, these results are promising for the potential to enhance biological control of squash bug.
Cucurbit Growers impacted by project: Green Hearts project urban farm [email protected] Rachel Meek Rooting Down Farms [email protected] Harleston Towles Joseph Fields Farm [email protected] Joseph Fields Crescent Farms [email protected] Margie Levine and Holly Welch Elysian Fields [email protected] Elise Bortz Seeds [email protected] Trevor Hyde Somerset [email protected] Olly & Frederick Perry-Winkle Farm [email protected] Cathy Jones Flow Farm [email protected] Mark Epstein New Ground [email protected], [email protected] Millard and Connie Locklear Agroecology Education farm [email protected] Alison Reves, Michelle Schroeder-Moreno Hilltop farms [email protected] Fred Miller Infinity Hundred Farms [email protected] David McConnell Raleigh City Farm [email protected] Lisa Grele Barrie Three Porch Farm [email protected] Woodland Gardens [email protected] Celia Barss Farm in the Wildwood [email protected] Danielle Pereira
- Our research on plastic mulch showed that black and silvery reflective mulches had a beneficial impact on squash yield compared to bare ground beds in one of the years. The reasons for these differences are not completely known, but are likely related to increased soil temperature and moisture retention resulting in greater plant growth and productivity. However, greater densities of squash bugs were found on plastic mulch plots compared to bare ground beds. Squash bug adults like to seek shelter in the planting hole of plastic mulch and consequently may deposit more eggs on those plants. Our study offers new insights for cucurbit growers to use when considering whether they should implement plasticulture in their growing systems.
-
Our research on living mulches revealed that although we predicted that biodiversity might mitigate collateral damage to natural enemies in pesticide-treated crops. Instead of attenuating non-target effects of pesticides, living mulches magnified them. Living mulches decreased cucumber beetles, but had no effect on squash bugs. Pest management should consider unique responses of locally dominant pests.
-
Our research on augmentative releases of the squash bug egg parasitoid Hadronotus pennsylvanicus showed that in both years, we found greater parasitism rates of A. tristis eggs collected at release sites compared to no-release sites. While all eggs collected at release and no-release sites before parasitoid deployment displayed low levels of H. pennsylvanicus parasitism, eggs from release sites were significantly more parasitized than eggs from no-release sites within two weeks post-deployment.
-
Based on our bioassays, flupyradifurone was the only reduced risk insecticide found to be both highly toxic to A. tristis nymphs and nontoxic to H. pennsylvanicus adults.
Information Products
- Effect of plastic mulch colors on Anasa tristis (Hemiptera: Coreidae) population dynamics in summer squash (Cucurbita pepo). Journal of Economic Entomology. 2022 in press (Peer-reviewed Journal Article)
- Benefits and risks of intercropping for crop resilience and pest management. Journal of Economic Entomology. toac045, (Peer-reviewed Journal Article)
- Boyle, S. M., A. M. Alford, K. C. McIntyre, D. C. Weber, and T. P. Kuhar. 2022. Effect of plastic mulch colors on Anasa tristis (Hemiptera: Coreidae) population dynamics in summer squash (Cucurbita pepo). J. Econ. Entomol. 115(3): 808–813. (Peer-reviewed Journal Article)
- Sean Boyle, Thomas P Kuhar, James Wilson, Donald C Weber. 2022. Effects of Some Selective Hemipteran-Targeting Insecticides and One Broad-Spectrum Insecticide on the Parasitoid Hadronotus Pennsylvanicus, 2018 Arthropod Management Tests, Volume 47, Issue 1, 2022, tsac074, (Peer-reviewed Journal Article)
- 4. Sean Boyle, Thomas P Kuhar, Donald C Weber. 2022. Effects of Selective Hemipteran-Targeting Insecticides and One Broad-Spectrum Insecticide on Squash Bug, 2018. Arthropod Management Tests, Volume 47, Issue 1, 2022, tsac073, (Peer-reviewed Journal Article)