Final report for ONE16-276
Project Information
Three years of monitoring the inputs and outputs of water and nitrogen at the Brooklyn Grange, an urban rooftop farm, suggested that increasing the water holding capacity of the soil could reduce the need for these supplements while at the same time reducing losses to leaching. In preliminary laboratory experiments, we tested the water holding capacity of 26 soilless materials with potential usefulness for rooftop farming. Subsequently, we selected the 2 best performing soilless materials, made 5 mixes, and compared them with Rooflite (the commercial blend the Grange had been using) in a greenhouse experiment to see how a leaf lettuce crop would respond to a 2.5 cm weekly irrigation, corresponding to the average weekly precipitation during the growing season. This management strategy should both maximize the amount of precipitation that could be absorbed by the soil and reduce the need for irrigation with potable water.
In the greenhouse, Rooflite performed worst in terms sof yield and quality while a mix of coir, biochar and compost performed best. Plants growing in Rooflite showed symptoms of drought stress under this minimum once weekly irrigation regime. It is important to note that the Grange had been applying unlimited irrigation to Rooflite. The soil mix with the highest yield (Mix 1: 66% coir, 9% biochar, 25% compost), and another soil mix with the minimum nitrogen leaching (Mix 2: 66% coir, 33% compost) were selected for testing on the Grange roof. In terms of water loss, Mix 1 lost 6% of the applied irrigation and Mix 2 lost essentially none. In comparison, Rooflite lost 11% of the water applied. In terms of nitrogen leachate losses, Mix 1 lost 0.1 g N m2, Mix 2 lost 0.4 g N m2 while Rooflite was essentially nil. During the 2016 growing season, both mixes were tested in 500 ft2 beds on the Grange roof. Lettuce in this practical field trial showed symptoms of nutrient deficiencies after 2, 1-month production cycles.
This package of information is the first of this kind and has informed cities with major rooftop farming initiatives, including NYC, Boston, Jersey City, Chicago, and Seattle. Managers and staff at Brooklyn Grange number 25 and learned and have applied the results with a new mix. Brooklyn Gange is a pilot project funded by the Community-Based Green Infrastructure Program of the New York City Department of Environmental Protection ($592,730 in 2012), and our research project directly informed the NYCDEP Best Management Practice Inventory and future projects funded by the program. Additionally, because the Grange has an internship and green job-training programs for college students, underrepresented local residents, and international visitors, this project has a ready-made, face-to-face extension audience. Information has be made available to a diverse stakeholder audience including the Cornell Small Farms Program, and the Grange website and presentations at both national and international meetings.
Laboratory Experiment. Conduct a preliminary screening of 26 artificial soil mixes and their components to determine their water holding capacity across a range of moisture tensions by using stacked soil rings and a tension table.
Greenhouse Experiment. Make 5 mixes by using two best performing soilless materials from the laboratory experiment. Compare these 5 mixes with Rooflite (a commercial soil product currently used at the Brooklyn Grange Navy Yard farm) by raising leafy lettuce under a minimum irrigation regime (25 mm / week) applied to pots 10cm and 30 cm deep. Determine crop yield, leachate volume, and their nitrogen contents.
Field Experiment. Select 1 soil mixe that produced the highest yield, and another mix that has the minimum nitrogen leaching, and install each to a planting bed at the Brooklyn Navy Yard farm. Monitor water and nitrogen use of planting beds composed of these two mixes, and Rooflite.
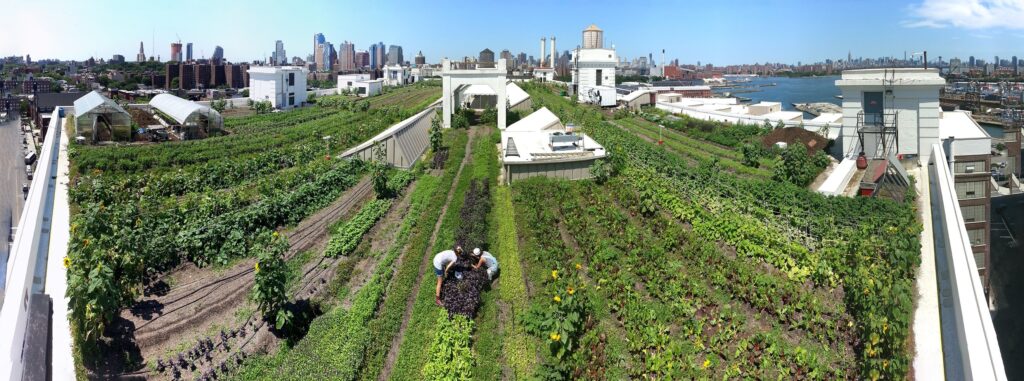
Context. Cities present a growing demand for fresh, nutritious, local vegetables, which has stimulated the growth of urban farms across America. Available space would appear to be a limiting factor, yet considering that New York City alone has over 8600 ha of flat roof space (25X the area of Central Park) that could support production agriculture, it is easy to imagine that commercial urban farming is both practical and a growth sector in urban economies. A rooftop farm differs from both in-ground farming, greenhouse production, and extensive green roofs, so production models used in these more typical systems require approaches that have yet to be formalized and that we are only now starting to understand. Among the many practical challenges facing a rooftop farm is the need for almost constant irrigation and fertilizer, with the parallel need to avoid water pollution from runoff drainage. At the center of both these issues is the need for a sustainable, soil-less growing medium for vegetables that retains water and nutrients instead of discharging them into storm sewers. Since May, 2014, we have been monitoring water use and nutrient fluxes at the Brooklyn Grange, a 0.6 ha farm on the roof of the Brooklyn Navy Yard, and found that annual discharge (1034 mm) was 100% of precipitation (1038 mm), and annual N leaching from soil (389 kg N ha-1) was 97% of fertilizer N application (403 kg N ha-1). This indicates the substantial opportunity to increase resource use efficiency.
Background. Several coinciding problems motivated this project. First is environmental pollution. “Dead zones” in the Chesapeake Bay and the Gulf of Mexico resulting from agricultural runoff are well known. Could they have been avoided if we had developed Best Management Practices (BMPs) to prevent the problem in the first place? Regarding roof top farms, we are on the rising limb of the curve for this new technology and have the extraordinary opportunity to develop BMPs that avoid excessive water use and nutrient runoff before they become problems. Drainage from the Grange discharges into the East River/Open Waters catchment, designated as Impaired by NYDEC due to combined sewer overflows. Second, rooftop vegetable production is sufficiently different from conventional in-ground farming, greenhouse production, and extensive green roofs that conventional practices adopted from these other systems are not a good fit. At the few rooftop farms in existence, fertilization and water requirements have been borrowed from in-ground vegetable farming in native mineral soil. Natural precipitation is insufficient to support vegetable production on a roof, so irrigation with potable water is needed even in the humid NE. From green roofs, rooftop farms have borrowed the lightweight, fast draining rooting medium to avoid overloading the roof. Green roof media resemble artificial greenhouse media, with the important difference that they are not replaced at the end of each cropping cycle. Importantly, on a conventional green roof, rapid growth and vegetable quality are not considerations. Third, the absence of a mineral soil component in the roof mix causes leaching of potassium and corresponding deficiency symptoms in leafy vegetables that affect quality. Further, high concentrations of ammonium detected in plants suggests that drought in the growing medium inhibits nitrification despite daily irrigation. Clearly, we need a novel cultural system specifically suited to rooftop farming. In this light, the challenge is to develop an optimal growing medium that retains more water, potassium and nitrogen than the current mix without exceeding the bearing capacity of an industrial roof. This will both avoid excess runoff and nutrient leaching.
Past work. A variety of studies have reported rates of drainage N output from agricultural, forested, and urban land uses. Whittinghill et al. (2016) reports the rate of drainage N output from an operational rooftop farm in NYC (33.6 kg N ha-1y-1) to be about three times greater than rates of maximum drainage N output from urban watersheds (11.4 kg N ha-1y-1, excluding wastewater treatment effluent), which falls within the wide range of N-saturated forests (0.04 - 47 kg N ha-1y-1) (Fenn et al., 1998; Howarth et al., 2002; Campbell et al., 2004; Groffman et al., 2004; Berndtsson et al., 2006; Gregoire and Clausen, 2011; Likens, 2013; Whittinghill et al., 2016). The rate of N loss from rooftop farms (33.6 kg N ha-1y-1) falls within the range of in-ground agriculture (2 – 353.7 kg N ha-1y-1), in-ground organic farming (25 – 36 kg N ha-1y-1), and in-ground farming using BMPs for no-tillage system with legume cover crops (4.1 – 33.9 kg N ha-1y-1), and the low end of in-ground intensive vegetable production (32.8 – 353.7 kg N ha-1y-1) (Goulding et al., 2000; Eriksen et al., 2004; Jayasundara et al., 2007; Zhao et al., 2010; Min et al., 2012; Pärn et al., 2012; Syswerda et al., 2012; Cameron et al., 2013; Whittinghill et al., 2016; Zhang et al., 2017). These observations suggest that relatively low N drainage from rooftop farming could be achieved but the best approaches to achieve this goal have not been determined.
There are many potential management practices urban farmers could use to reduce drainage output of nutrients into nearby waterways. For example, reducing drainage volume can reduce drainage N output from soil-plant systems on roofs (Di and Cameron, 2002; Hartz, 2006; Cameron et al., 2013). However, this effort can be difficult when expanded shale, clay and slate (ESCS) is used as the base material in rooftop soils, ESCS holds less water and nutrients than field soils, amplifying the potential for drainage output (Emilsson et al., 2007; Ampim et al., 2010; Best et al., 2015; Harada et al., 2018). Even in non-production green roofs where plant yield and quality are arguably less important, fertilizer and irrigation inputs during the initial establishment period can increase drainage output of nutrients (Emilsson et al., 2007; Berndtsson, 2010; Rowe, 2011; Driscoll et al., 2015). In comparison to vegetables, however, non-crop vegetation such as Sedum spp. requires less water and nutrients, thus drainage losses of nutrients from these roofs can be reduced after establishment by reducing or terminating water and nutrient subsidies (see Congreves and Van Eerd, 2015; Shock and Wang, 2011; Van Mechelen et al., 2015; Emilsson et al., 2007). In rooftop farms, however, economic viability relies on the yield and quality of diverse vegetables with high market value, such as salad greens, tomatoes, peppers, and eggplants, which have high requirements for water and nutrients (Shock and Wang, 2011; Ackerman et al., 2013; Congreves and Van Eerd, 2015). Since not all of the added nutrients are taken up by these vegetables, greater rates of fertilizer application can lead to greater drainage output of nutrients (Cameron et al., 2013).
These observations suggest that relatively low N in drainage from rooftop farming could be accomplished, but the best approaches to achieve this goal have not been determined. We have partnered with the Brooklyn Grange (Figure 1) since 2013, and they are now in the process of installing a third farm. The Brooklyn Grange is a pilot project of the Community-Based Green Infrastructure Program funded by the New York City Department of Environmental Protection. Construction of the farm cost $592,730 in 2012.
Cooperators
Research
Laboratory Experiment. We determined the available moisture held in 26 soil media and their components in preliminary lab tests, in which, the volumetric water contents was measured at 1 kPa by gravitational drainage using stacked soil rings replicated 6 times, and at 10 kPa and 30 kPa by using a sand table replicated 36 times respectively. Measurement at 1 kPa provided the estimates of water holding capacity of each media around the surface of 10cm-deep pots used in the subsequent greenhouse trial. Measurement at 10 kPa provided the estimates of water holding capacity commonly used for soilless media. Measurement at 30 kPa provided the estimates of lowest volumetric water contents that could acceptable yields of leafy vegetables with relatively high irrigation demands (Shock and Wang, 2011).
Greenhouse Experiment. We grew leaf lettuce as an indicator crop in growth media composed of composted food waste mixed with varying proportions of biochar and coconut coir, and each of these mixes was installed at depths of 10cm and 30cm. Six combinations of soil mixes at each depth, each replicated 5 times, were tested in the greenhouse at Cornell, with 25mm-irrigation applied once a week, for 5 weeks (Table 2). The response variables included 1) leachate volume 2) nitrogen leaching, and 3) plant yield and its nitrogen content. The mix that had the highest yield, and another mix that had the minimum nitrogen leaching, were tested in plots at the Brooklyn Navy Yard to validate findings and allow us to develop guidelines for irrigation and fertilization schedules.
Media type |
Volumetric Ratio (%) |
||
Coconut Coir |
Compost |
Biochar |
|
experimental mix |
100 |
0 |
0 |
experimental mix |
50 |
50 |
0 |
Commercial mix |
66 |
34 |
0 |
Commercial mix |
66 |
25 |
9 |
experimental mix |
33 |
34 |
33 |
Commercial mix (RoofLite) |
the soil mix the Brooklyn Grange had been using |
Table 2. media composition used in the greenhouse experiment
Field Experiment: Site Description. The site for our field study is the Brooklyn Grange Navy Yard farm, an irrigated rooftop vegetable farm on an 11-story building at the former Brooklyn Navy Yard, New York City, USA (Figure 1). The Community-Based Green Infrastructure Program of the NYC Department of Environmental Protection funded $592,730 for the construction, anticipating that stormwater management would be an equally important goal of the farm in addition to the vegetable production (NYC DEP 2011). While the farm grows over 60 crops, leaf lettuce (Lactuca sativa), mustard greens (Brassica juncea), arugula (Eruca sativa), and tomato (Solanum lycopersicum) can account for over the half of planting bed area, sales, and yield in fresh weight.
Field Experiment: Soil Description. The soil used on the site is Rooflite® Intensive Ag (Rooflite hereafter), a commercial soil blend of expanded shale, animal manure compost, and the spent substrate from mushroom production, which is commonly made of animal manure and lignocellulosic materials such as cereal straws and saw dusts (Kong et al. 2015; Paredes et al. 2009; Phan and Sabaratnam 2012; Skyland USA LLC 2015). The flat tops of planting beds (250 mm deep) are typically 1000-mm wide. Bare soil between planting beds (25-50 mm deep) are typically 430-mm wide. Soil is underlain by filter fabric, a drainage layer (100-150 mm deep) filled with expanded shale, and a water-proofing membrane.
Field Experiment: Nitrogen and Water balance. The nitrogen and water balance of the Brooklyn Grange has been monitored between the 2015 and 2016 growing seasons, using a micro meteorology station, online irrigation controllers, a micro v-notch weir with sonic distance sensor, wireless soil moisture sensors, passive atmospheric deposition collectors with ion exchange resin, and mesh bags containing ion exchange resins buried at the bottom of the soil beds. Resin was collected and analyzed every 6 weeks.
Field Experiment: Improved Growing Media. Based on the greenhouse trials, two best performing media were selected for field trial in 500 m2 beds at the Grange. The input, retention, loss from harvested vegetables, and leaching of nutrients and water are being monitored in the ongoing biogeochemical monitoring since May 2014. Year 1 (2016) of the proposed study was intended to determine which growing medium achieved the best crop performance while reducing nutrient leaching. The optimum design was scheduled to be applied to the entire farm in 2018 after this grant terminates. The biogeochemical performance of the farm will continue to be monitored by the Grange in the framework of ongoing experimentation.
Water holding capacity of soilless materials. In comparison to other soilless materials tested in this study, coconut coir, biochar, and their blends held more water (> 40%) between 1-30kPa of soil water tension, which is the estimated volume of water readily available for water-sensitive leafy vegetables at the surface of 10cm-deep media (Figure 3).
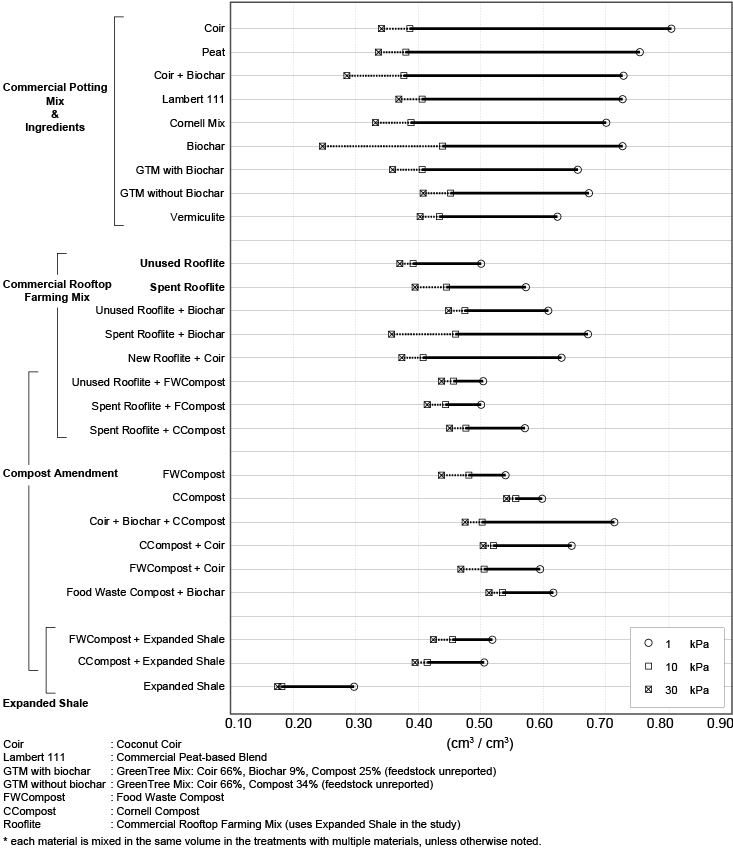
Water balance. While differences between the greenhouse and rooftop experiments make it impossible to make direct comparisons, it is informative to compare general trends and patterns. In the annual water balance of field monitoring on rooftop between December 1, 2015 – November 30, 2016, discharge was 100% of precipitation and was 75% of the total water input (precipitation + irrigation) (Table 4). For this field monitoring, the media used was Rooflite, and the depths of planting bed ranged 20 – 30cm. In the greenhouse trial, 0-21% of irrigation applied was lost by leaching (Table 5). Maximum leachate volume was observed for Rooflite regardless of depth while the minimum volume was from the GreenTree mix (Table 5). Rooflite at 30cm depth in greenhouse trial lost 11% of irrigation by leaching, which is much less than the leaching (75%) in the field monitoring on rooftop. Among the possible factors is the irrigation rates in the field, which was adjusted based on the farmer’s visual observation of plants vigor and growth, while in the greenhouse trial, irrigation rate was the same (25mm weekly) during the study. The irrigation rate in greenhouse trial was insufficient for the Rooflite, in which the yield was nil in both depths.
Period |
Precipitation mm |
Irrigation mm |
Discharge mm |
12/1/2015 – 11/30/2016 |
1038 |
337 |
1034 |
Table 4. Annual water balance of the Brooklyn Grange Navy Yard farm, NYC. Soil mix was Rooflite.
|
Coir 100% |
Coir 66% |
Coir 50% |
Coir 33% |
Coir 66% |
Rooflite |
||
30 cm |
input |
irrigation |
100 |
100 |
100 |
100 |
100 |
100 |
output |
Leaching from soil |
7 (73) |
6 (62) |
7 (73) |
7 (73) |
0 (0) |
11 (114) |
|
10 cm |
input |
irrigation |
100 |
100 |
100 |
100 |
100 |
100 |
output |
Leaching from soil |
15 (156) |
10 (104) |
17 (177) |
13 (135) |
9 (94) |
21 (218) |
Table 5. Greenhouse Water Balance for the 5-week experiment. All numbers are expressed in mm of water depth applied to the pots. Numbers in parentheses are expressed as the equivalent drained from the pots over a 52-week period. The first and last irrigations were intentionally irrigated to excess and are not included in the balance.
Nitrogen balance. In the annual N balance of field monitoring on rooftop, N leaching from the soil was 97-115% of fertilizer application, and N output by leaching was 170-259 % of N output by vegetable harvest (Table 6). In the greenhouse trial, N output by leaching was nil for 30cm-deep Rooflite, yet N output by the vegetable harvest was very little due to the severely reduced yield (Table 7, 8). Among the possible factors for the reduced N leaching from Rooflite in the greenhouse trial, is the irrigation rate of 25mm per week, while in the field monitoring on rooftop, irrigation was applied as needed, which increased the leachate volume and the N loss by leaching (Table 4, 6). The ranking of yield in fresh weight, dry weight, and N output by the vegetable harvest depended on the soil type, and highest for GreenTree mix with biochar, which also had highest N output by leaching (Table 7). The yield and N output by harvest were second highest for GreenTree mix without biochar, which also had lowest N output by leaching among the treatments with acceptable yields (Table 7). GreenTree mixes with and without biochar was selected for the field test on rooftop.
|
Expressed for |
||
2014-2015 |
2015-2016 |
||
input |
atmospheric deposition* |
9 |
9 |
fertilizer application |
325 |
403 |
|
total input |
333 |
413 |
|
output |
leaching from soil |
375 |
389 |
vegetable harvest |
145 |
229 |
|
total output |
520 |
618 |
Table 6. Annual N balance of the Brooklyn Grange Navy Yard farm, NYC between November 12, 2014– November 12, 2015, and between November 12, 2015 – November 12, 2016. Soil mix was RoofLite. Daily average values of each sampling period were summed to give estimates of annual fluxes, including winter when no crops were being produced. *Bulk collection 1.5m above the soil surface. Note that the units differ from the units used in the small scale greenhouse experiment.
|
Coir 100% |
Coir 66% |
Coir 50% |
Coir 33% |
Coir 66% |
Rooflite |
||
30 cm |
input |
Initial |
252.0 |
499.0 |
244.0 |
468.0 |
585.0 |
532.0 |
output |
vegetable harvest |
0.0 (0) |
6.9 (72) |
0.6 (6) |
7.8 (81) |
17.3 (178) |
0.1 (1) |
|
Leaching |
0.4 (4) |
0.1 (1) |
0.1 (1) |
0.1 (1) |
0.4 (4) |
0.0 (0) |
||
10 cm |
input |
Initial |
91.0 |
194.0 |
84.0 |
163.0 |
183.0 |
172.0 |
output |
vegetable harvest |
0.0 (0) |
1.0 (10) |
0.9 (9) |
3.2 (33) |
12.0 (125) |
0.1 (1) |
|
Leaching |
0.1 (1) |
0.1 (1) |
0.1 (1) |
0.1 (1) |
8.0 (83) |
0.1 (1) |
||
Ranking of Yield |
6 |
2 |
4 |
3 |
1 |
5 |
Table 7. Greenhouse N balance. Initial Soil N is expressed as g N m-2. N outputs are expressed as g N m-2 5 weeks-1, corresponding to the duration of the experiment. For comparison, numbers in parentheses are calculated for a 52 week period, corresponding to the units for the field observations at the Grange.
Coir 100% |
Coir 33% + Biochar 33% + Compost 34% |
![]() |
![]() |
Coir 66% + Compost 34% |
Coir 66% + Biochar 9% + Compost 25% |
![]() |
![]() |
Coir 50% + Biochar 50% |
Rooflite |
![]() |
![]() |
Figure 8. Growth of lettuce after 5 week greenhouse trial
We accomplished all of our planned experiments, sample collection, and sample preparation. Sample analysis has been delayed due to a change in lab personnel at the service lab operated by our collaborator, Pamela Templer, at Boston University. While this delayed our ability to report ultimate outcomes and conclusions until winter, 2018, we are pleased to submit our final report at this time.
Moisture release curves comparing 26 different media and components indicate that expanded shale, the major component of the commercial RoofLite mix, retains the least water between 1, 10, and 30 kPa (Figure. 3), while coconut coir retains the most. The commercial Rooflite mix is in the middle of the field. Taken together, it appears that coir could be substituted for expanded shale to increase moisture holding capacity and thus reduce the amount of water and nutrients lost to drainage.
The greenhouse experiment with leafy lettuce clearly illustrates the impact that growing medium composition and depth have on crop yield (Figure. 8). Despite the fact that the coconut coir has high water holding capacity, the treatment of 100% coconut coir does not produce maximum plant growth. It is noteworthy that the yield of standard RoofLite mix is virtually indistinguishable from yield in coir. For the treatment of 100% coconut coir, the limited growth potential is probably because the lack of compost limited the plant avainable nitrogen, by reducing the mineralization rate of organic nitrogen. For Rooflite, the limited growth potential is probably the result of low moisture contents, because Rooflite achieved the acceptable yield and quality in the Brooklyn Grange, where irrigation was applied as needed.
In media with large pore radii like RoofLite, greater depth increases hydraulic head which causes more drainage from the shallower part of the profile, causing water stress that in turn reduces growth (Figure. 3). In media that include a range of pore sizes, like the Coir + Char + Compost mix, growth is increased regardless of depth (Table 7). Addition of the compost increases the available organic N which is otherwise largely absent in the media.
References
Ackerman, K., Dahlgren, E., and Xu, X. (2013). "Sustainable Urban Agriculture: Confirming Viable Scenarios for Production, Final Report No.13-07".).
Ampim, P.A., Sloan, J.J., Cabrera, R.I., Harp, D.A., and Jaber, F.H. (2010). Green roof growing substrates: types, ingredients, composition and properties. Journal of Environmental Horticulture 28(4), 244.
Berndtsson, J.C. (2010). Green roof performance towards management of runoff water quantity and quality: A review. Ecological Engineering 36(4), 351-360.
Berndtsson, J.C., Emilsson, T., and Bengtsson, L. (2006). The influence of extensive vegetated roofs on runoff water quality. Science of the Total Environment 355(1), 48-63.
Best, B.B., Swadek, K.R., and Burgess, L.T. (2015). "Soil-Based Green Roofs," in Green Roof Ecosystems, ed. K.R. Sutton. (Cham: Springer International Publishing), 139-174.
Cameron, K., Di, H., and Moir, J. (2013). Nitrogen losses from the soil/plant system: a review. Annals of Applied Biology 162(2), 145-173.
Campbell, J.L., Hornbeck, J.W., Mitchell, M.J., Adams, M.B., Castro, M.S., Driscoll, C.T., et al. (2004). Input-output budgets of inorganic nitrogen for 24 forest watersheds in the northeastern United States: A review. Water Air and Soil Pollution 151(1-4), 373-396. doi: 10.1023/b:wate.0000009908.94219.04.
Congreves, K., and Van Eerd, L. (2015). Nitrogen cycling and management in intensive horticultural systems. Nutrient cycling in agroecosystems 102(3), 299-318.
Di, H., and Cameron, K. (2002). Nitrate leaching in temperate agroecosystems: sources, factors and mitigating strategies. Nutrient cycling in agroecosystems 64(3), 237-256.
Driscoll, C.T., Eger, C.G., Chandler, D.G., Davidson, C.I., Roodsari, B.K., Flynn, C.D., et al. (2015). Green Infrastructure: Lessons from Science and Practice. A publication of the Science Policy Exchange. 32.
Emilsson, T., Berndtsson, J.C., Mattsson, J.E., and Rolf, K. (2007). Effect of using conventional and controlled release fertiliser on nutrient runoff from various vegetated roof systems. Ecological engineering 29(3), 260-271.
Eriksen, J., Askegaard, M., and Kristensen, K. (2004). Nitrate leaching from an organic dairy crop rotation: the effects of manure type, nitrogen input and improved crop rotation. Soil Use and Management 20(1), 48-54.
Fenn, M.E., Poth, M.A., Aber, J.D., Baron, J.S., Bormann, B.T., Johnson, D.W., et al. (1998). Nitrogen excess in North American ecosystems: predisposing factors, ecosystem responses, and management strategies. Ecological Applications 8(3), 706-733.
Goulding, K.W.T., Poulton, P.R., Webster, C.P., and Howe, M.T. (2000). Nitrate leaching from the Broadbalk Wheat Experiment, Rothamsted, UK, as influenced by fertilizer and manure inputs and the weather. Soil Use and Management 16(4), 244-250.
Gregoire, B.G., and Clausen, J.C. (2011). Effect of a modular extensive green roof on stormwater runoff and water quality. Ecological Engineering 37(6), 963-969.
Groffman, P.M., Law, N.L., Belt, K.T., Band, L.E., and Fisher, G.T. (2004). Nitrogen fluxes and retention in urban watershed ecosystems. Ecosystems 7(4), 393-403.
Harada, Y., Whitlow, T.H., Todd Walter, M., Bassuk, N.L., Russell-Anelli, J., and Schindelbeck, R.R. (2018). Hydrology of the Brooklyn Grange, an urban rooftop farm. Urban Ecosystems. doi: 10.1007/s11252-018-0749-7.
Hartz, T. (2006). Vegetable production best management practices to minimize nutrient loss. HortTechnology 16(3), 398-403.
Howarth, R.W., Sharpley, A., and Walker, D. (2002). Sources of nutrient pollution to coastal waters in the United States: Implications for achieving coastal water quality goals. Estuaries 25(4), 656-676.
Jayasundara, S., Wagner-Riddle, C., Parkin, G., von Bertoldi, P., Warland, J., Kay, B., et al. (2007). Minimizing nitrogen losses from a corn–soybean–winter wheat rotation with best management practices. Nutrient Cycling in Agroecosystems 79(2), 141-159. doi: 10.1007/s10705-007-9103-9.
Kong, A.Y., Rosenzweig, C., and Arky, J. (2015). Nitrogen Dynamics Associated with Organic and Inorganic Inputs to Substrate Commonly Used on Rooftop Farms. HortScience 50(6), 806-813.
Likens, G.E. (2013). Biogeochemistry of a forested ecosystem. Springer Science & Business Media.
Min, J., Zhang, H., and Shi, W. (2012). Optimizing nitrogen input to reduce nitrate leaching loss in greenhouse vegetable production. Agricultural water management 111, 53-59.
NYC DEP (2011). DEP Awards $3.8 Million in Grants for Community-Based Green Infrastructure Program Projects. NYC DEP Webpage [Online] <http://www.nyc.gov/html/dep/html/press_releases/11-46pr.shtml#.VvIn6fkrKUk> (Accessed on April 01, 2016).
Paredes, C., Medina, E., Moral, R., Pérez‐Murcia, M.D., Moreno‐Caselles, J., Angeles Bustamante, M., et al. (2009). Characterization of the different organic matter fractions of spent mushroom substrate. Communications in soil science and plant analysis 40(1-6), 150-161.
Pärn, J., Pinay, G., and Mander, Ü. (2012). Indicators of nutrients transport from agricultural catchments under temperate climate: A review. Ecological indicators 22, 4-15.
Phan, C.-W., and Sabaratnam, V. (2012). Potential uses of spent mushroom substrate and its associated lignocellulosic enzymes. Applied Microbiology and Biotechnology 96(4), 863-873. doi: 10.1007/s00253-012-4446-9.
Rowe, D.B. (2011). Green roofs as a means of pollution abatement. Environmental Pollution 159(8), 2100-2110.
Rowe, D.B., Kolp, M.R., Greer, S.E., and Getter, K.L. (2014). Comparison of irrigation efficiency and plant health of overhead, drip, and sub-irrigation for extensive green roofs. Ecological Engineering 64, 306-313.
Shock, C.C., and Wang, F.-X. (2011). Soil water tension, a powerful measurement for productivity and stewardship. HortScience 46(2), 178-185.
Skyland USA LLC (2015). "Rooflite Intensive Ag Product Specification" <http://www.rooflitesoil.com/products/intensive-ag>accessed June 01, 2016.
Syswerda, S., Basso, B., Hamilton, S., Tausig, J., and Robertson, G. (2012). Long-term nitrate loss along an agricultural intensity gradient in the Upper Midwest USA. Agriculture, Ecosystems & Environment 149, 10-19.
Van Mechelen, C., Dutoit, T., and Hermy, M. (2015). Adapting green roof irrigation practices for a sustainable future: A review. Sustainable Cities and Society 19, 74-90.
Whittinghill, L.J., Hsueh, D., Culligan, P., and Plunz, R. (2016). Stormwater performance of a full scale rooftop farm: Runoff water quality. Ecological Engineering 91, 195-206.
Zhang, B., Li, Q., Cao, J., Zhang, C., Song, Z., Zhang, F., et al. (2017). Reducing nitrogen leaching in a subtropical vegetable system. Agriculture, Ecosystems & Environment 241, 133-141.
Zhao, C., Hu, C., Huang, W., Sun, X., Tan, Q., and Di, H. (2010). A lysimeter study of nitrate leaching and optimum nitrogen application rates for intensively irrigated vegetable production systems in Central China. Journal of Soils and Sediments 10(1), 9-17.
Education & Outreach Activities and Participation Summary
Participation Summary:
Yoshiki Harada, a student of PI Whitlow, presented the results of this study in the session for urban agriculture at the annual meeting of Ecological Society of America 2015, Baltimore MA, and the presentation was entitled “Biogeochemistry of the Brooklyn Grange, an Urban Rooftop Farm”. He also presented “What we learned from the Brooklyn Grange” in the annual meeting of Grey to Green 2016, Toronto, Canada, which is the association of landscape designers and urban planners around the topics of urban green infrastructure projects.
This package is the first of this kind and will immediately inform cities with major rooftop farming initiatives, including NYC, Boston, Jersey City, Chicago, and Seattle. Because the Grange is a pilot project funded by the Community-Based Green Infrastructure Program of the New York City Department of Environmental Protection ($592,730 in 2012), our project will also directly inform the NYCDEP Best Management Practice Inventory and future projects funded by the program. Additionally, because the Grange has an internship and green job-training programs for college students, underrepresented local residents, and international visitors, this project has a ready-made, face-to-face extension audience. Information will be made available to a diverse stakeholder audience including the Cornell Small Farms Program, and the Grange website and presentations at both national and international meetings.
We are pleased to report that the first of 2 papers dealing with rooftop agriculture has been accepted for publication:
Harada, Yoshiki, Thomas H. Whitlow, Nina L. Bassuk, and Jonathan Russell-Anelli. "Biogeochemistry of rooftop farm soils." Urban Soils. Advances in Soil Science Series. Taylor & Francis Group, Portland Google Scholar (2017).
Harada, Yoshiki, Thomas H. Whitlow, M. Todd Walter, Nina L. Bassuk, Jonathan Russell-Anelli, and Robert R. Schindelbeck. "Hydrology of the Brooklyn Grange, an urban rooftop farm." Urban Ecosystems (2018): 1-17.
Learning Outcomes
The balance of water and nitrogen was shared with all managing farmers of the Brooklyn Grange in November 2015, with emphasis on the suboptimal management of water and nitrogen, and the need of alternative soil to improve the efficiency of the management.
In March 2016, Benjamin Flanner visited Cornell University to see the greenhouse experiment, where production of leafy lettuce was significantly more in the alternative soil (coconut coir 66%, compost 25%, biochar 8%) than in Rooflite, under the limited irrigation (25 mm weekly). Flanner also visited the factory of GreenTree Garden Supply, to see the base materials and manufacturing process of the alternative soil. During this visit, Flanner decided to switch all potting mix products used in the Grange’s greenhouse to the alternative soil and reuse the spent alternative soil to amend existing soil.
In the early 2016 growing season, farmers and interns recognized that the amendments increased the water holding capacity, which improved the germination rates and growth of vegetables. Based on this experience, the farmers agreed to install the planting beds, and test the alternative soil under field condition, which started in June 2016.
During this field test, farmers learned 3 lessons. Firstly, the alternative soil requires less irrigation, while achieving more germination and growth of vegetables. Secondly, farmers found that the drip tape is more efficient than sprinkler to irrigate the alternative soil, whereas sprinkler is more efficient for Rooflite. This agrees with the findings of Rowe et al. (2014), who tested various combinations of irrigation methods and soil type for green roofs. Lastly, the 150mm-deep planting beds of the alternative soil subsided for 50mm over the 2016 growing season. This means that the new soil must be imported to the planting beds every year, and the farm-wide application could be expensive. Currently, the Grange is planning to increase the planting beds of the alternative soil, while continuing to amend existing planting beds of Rooflite with the spent alternative soil from greenhouse.
Project Outcomes
Impacts
The immediate outcome of this study will be a new soilless medium for rooftop farming, accompanied by guidelines for optimum irrigation, and nutrient application, referenced to produce yield and quality, and rates of water and nutrient loss. This study also made scientific approaches as an integral part of the farming operation at the Brooklyn Grange, where farmers learned how to collect and transport soil and vegetable samples for laboratory tests, and how to interpret and apply knowledge from the test results by collaborating with faculty members and students from Cornell.
In the process of making and testing new soilless media, the collaboration of this study expanded to include Skyland, the manufacturer of Rooflite, and GreenTree, a soilless blend manufacturer, Ithaca, NY. The Brooklyn Grange, Skyland, and GreenTree exchanged information about the cost and availability of diverse soilless materials, and the process of making new soilless blends. This became a foundation for the Brooklyn Grange to continue research and development of new soilless media for the rooftop vegetable production.
The Brooklyn Grange will be installing its third rooftop farm this summer and plans to use the coir/char/compost mix we developed in this project.
Nicole Smaranda, a undergraduate student of Jonathan Russell-Anelli, was included in this collaboration. For her undergraduate thesis project, she conducted the greenhouse experiment to determine the effective modification of material composition for Rooflite, in order to increasing the water holding capacity and plant available water for leafy lettuce. Philip Duvall, a graduate student of Todd Walter, is also included in this collaboration.
Subsequent trials using 5% of pond sediment will be conducted by the Grange during the 2018 growing season, independent of this project. An important finding of this research is that experiments under actual farm conditions are necessary to determine the optimum soil mix.