Final report for GNE21-270
Project Information
Fusarium (Fusarium verticillioides) and Gibberella (Fusarium graminearum) ear and stalk rot are toxicologically important diseases of maize in the Northeast. While Fusarium infection can reduce maize yield, the mycotoxins produced by these pathogens (fumonisin and deoxynivalenol (DON)) are a significant concern, threatening human and animal health. Management is limited to the use of genetic resistance via transgenic Bt maize, which is inaccessible to organic producers. Cover cropping is an accessible alternative practice with potential for Fusarium management. Cover crops are known to modify nutrient cycling, soil biological activity, and plant defense pathways. Mycorrhizal colonization is also influenced by cover crop legacy and has previously been found to activate plant defense against insect herbivory and soilborne pathogens; yet more research is needed to understand how these modifications influence above ground fungal pathogens. This research aims to identify the effect of various cover crop species legacies (triticale, oat, radish, canola, pea, and clover) and mycorrhizal colonization on maize disease severity and mycotoxin contamination by Fusarium spp.
In this research project we investigated the effect of cover crop species legacy on stalk rot severity and mycotoxin accumulation caused by F. graminearum and F. verticillioides. We found that while cover crop legacy can influence mycotoxin accumulation in certain treatments and years, the results were not consistent between years. We also investigated the effect of mycorrhizal colonization on Fusarium disease severity. Results suggest that mycorrhizal colonization may increase fusarium disease severity in greenhouse conditions, however it is unclear whether this result is reflected in a field setting. Our findings reveal that year-to-year and field level variation have the greatest impact on mycotoxin accumulation and the impact of cover crop legacy remains inconclusive. However, mycorrhizal colonization in maize may increase stalk rot disease severity. We hope that this research inspires similar work to understand the role of cover cropping and other crop management techniques on mycotoxin management, as the necessity for affordable and sustainable management remains.
Objective 1: To Identify the effect of cover cropping legacy on mycotoxin accumulation and disease severity in maize during Fusarium and Gibberella stalk and ear rot.
Specific objectives are to:
a. Assess the effect of cover crop legacy on disease severity (lesion size) of maize due to Gibberella (F. graminearum) and Fusarium (F. verticillioides) stalk rot.
b. Assess the effect of cover crop legacy on the DON contamination of ears of maize due to Gibberella ear rot disease (F. graminearum).
c. Assess the effect of cover crop legacy on the Fumonisin B1 and Fumonisin B2 contamination of ears of maize due to Fusarium ear rot disease (F. verticillioides)
Objective 2: To identify the interaction between cover cropping legacy, mycorrhizal colonization, and ear rot disease in maize.
The purpose of this project was to quantify the effect of cover cropping legacy on mycotoxin accumulation and disease severity of maize infected with F. graminearum and F. verticillioides. These are important maize pathogen species in the Northeast, contributing to stalk and ear rot. While fusarium infection can contribute to yield loss during both ear and stalk rot, the production of mycotoxins is of greatest concern. Mycotoxins are non-enzymatic metabolites that are toxic to animals or humans during consumption. Deoxynivalenol (DON) is a significant mycotoxin produced by F. graminearum and can cause vomiting, feed refusal and reduced immune functioning in livestock. The primary mycotoxin produced by F. verticillioides is fumonisin, which causes the fatal livestock diseases equine leukoencephalomalacia, porcine pulmonary edema, and cancer in laboratory animals, and is correlated with esophageal cancer and embryonic developmental defects, and neurological disease in humans. Because maize kernels are used in food products for humans and animals, and stalks may be used for livestock feed as silage, reducing mycotoxin accumulation through Fusarium disease management is critical.
While management options for Fusarium ear and stalk rot disease exist, they are often limited in efficacy and are typically focused on conventional production systems. Plant genetic resistance is the most important management tactic for mycotoxin reduction in maize. While targeted genetic resistance to Fusarium has proven to be illusive, Bt maize (a transgenic variety that produces insect toxins derived from the soil bacterium Bacillus thuringiensis) has effectively reduced both ear and stalk rot through a reduction in insect herbivory. Nonetheless, Bt maize is currently not an option for organic production and is also becoming less effective for conventional growers due to increasing insect resistance to Bt. Alternative approaches must be explored for the reduction of Fusarium disease.
Various cultural management practices have been shown to impact mycotoxin development in maize and other field crops. While the implementation of cover cropping systems (cultivating non-cash crops over winter periods between cash crop seasons) in PA has been steadily increasing, limited research has been conducted on the effect of this practice on Fusarium disease. Cover crops provide many agronomic benefits, including increased fertilizer and irrigation retention and weed suppression. In some cases, they have been shown to reduce insect pest and pathogen damage in the following crop. These disease interactions are nuanced, and the exact effect of the cover crop for disease suppression depends on the cover crop species, pathogen, and host plant. I hypothesized that different cover cropping species would have differing effects on mycotoxin and Fusarium disease severity due to the unique ecosystem services they provide. Species that reduced disease could be leveraged as a sustainable management option for mycotoxin reduction, which would contribute to improved human and animal health and increased farmer income, as these diseases result in an annual yield loss in maize 340,170 bushels costing $1.4 million in PA.
Cooperators
- (Researcher)
Research
To quantify the effect of cover cropping on Fusarium disease severity and mycotoxin contamination in maize, a combination of field and greenhouse experiments were performed. Experiments investigated ear and stalk rot disease in maize grown in legacies of different cover crop monocultures. Field experiments were conducted at the Cover Crop Cocktails site at The Russell E. Larson Agricultural Research Center at Rock Springs, PA. This research site consisted of a randomized complete block design with four replications of the following crop rotation: cover crop -> maize -> rye cover crop -> soybean -> wheat -> cover crop. Cover crops include two cereal cover crops (triticale, oats), two leguminous cover crops (winter pea, clover) and two brassicaceous cover crops (canola, forage radish).
With the financial support of this grant, I hired undergraduate students to assist in establishing and maintaining greenhouse experiments, Fusarium inoculations, disease severity, and mycorrhizal colonization analyses. Through these experiences undergraduate students were involved with multiple stages of the scientific process. They gained the laboratory and field research skills necessary to conduct independent scientific research in the future.
For Objective 1a (Assess the effect of cover crop legacy on disease severity of successive maize due to Gibberella and Fusarium stalk rot), soil was collected from the following cover crop plots; triticale, radish, pea, and fallow plots directly after cover cropping and before maize planting in May 2022. In September 2022, forty corn plants (MC-3890 variety) were grown directly in each soil type in the greenhouse. Stems from twenty plants in each soil treatment were inoculated with potato dextrose agar (PDA) plugs containing F. verticillioides tissue or sterile PDA as a control. Inoculations occurred at the V9-10 growth stage once nodes were detectable by touch. A sterile needle was used to create a hole in the center of each stem, and a plug of treatment agar was pressed on the hole and secured with Parafilm. After two weeks, the stem was split in half vertically along the line of the hole, and photographs were taken with a ruler for calibration. The area of the rot lesion was measured by using ImageJ software. The relationship between cover crop legacy and lesion area was analyzed using a one-way ANOVA in Rstudio (v4.2.1) with the p-value set to 0.05. Mean comparisons of treatments will be made using a Tukey's Honestly Significant Difference (Tukey-HSD) test.
Fig 1. Field soil from the cover crop cocktail field site was collected with the help of members of a collaborating lab and stored in a cold room until experimental use.
To address Objective 1b (Assess the effect of cover crop legacy on the DON contamination of ears of successive maize due to Gibberella ear rot disease), maize ears were inoculated with F. graminearum in each maize plot as part of the cover crop cocktail trial during the 2021 and 2022 growing seasons. Ten plants per plot were inoculated with F. graminearum or H2O (control). This was performed on all four replicate plots of individually cover cropped treatments (triticale, oats, canola, forage radish, winter pea, clover), as well as the four fallow treatment plots.
In 2021, inoculations were performed via silk channel injection during the first 6 days after silk emergence, when ears are the most susceptible. A liquid spore suspension (5 x 105 spores/mL) was injected directly into the silks with a blunt tipped 18.5 gauge syringe. Maize ears were harvested from the field at maturity, and ear rot severity was evaluated using the rating scale described by Reid et al. (1992). Severity will be estimated as 1 = no infection, 2 = 1–3% of kernels infected, 3 = 4–10%, 4 = 11–25%, 5 = 26–50%, 6 = 51–75%, and 7 = 76%+.
Due to observations of extremely high disease pressure in 2021, the inoculation strategy and disease severity measurement was modified for 2022. A toothpick inoculation method was performed rather than a silk channel injection. Sterilized toothpicks were colonized with F. graminearum by incubating for three weeks in flasks with an 0.5mm layer of liquid Czapek-Dox media and an F. graminearum spore suspension or just Czapek-Dox media (control). Fourteen days after silk emergence, when kernels were developing and beginning to milk, one toothpick was stabbed into the side of each ear and left in the ear until harvest (Fig 2).
During both years, inoculated ears from each replicate plot were pooled, as well as a group of non-inoculated ears in each plot. These samples were ground and analyzed for mycotoxin levels. Two analyses were performed per sample to ensure analysis accuracy. The major mycotoxin associated with F. graminearum is deoxynivalenol (DON). DON accumulation was measured through high pressure liquid chromatography – ultraviolet detection (HPLC-UV) following an immunoaffinity cleanup.
The relationship between cover crop legacy and mycotoxin contamination was analyzed using a two-way ANOVA test in Rstudio with the p-value = 0.05. Mean comparisons of treatments were made using a Tukey-HSD test. Regression models were used to characterize the relationship between individual cover crop treatments and DON contamination.
Fig. 2A At the silking stage, corn plants were inoculated with Fusarium pathogens through silk channel injection method.
Fig. 2B The assistance of fellow M.S. student Tyler McFeaters and friend Ashley Fogelsanger was crucial to completing inoculations in one day.
Fig. 2C A toothpick inoculation method was performed in 2022 rather than a silk channel injection
To address Objective 1c (Assess the effect of cover crop legacy on the fumonisin B1 and fumonisin B2 contamination of ears of successive maize due to Fusarium ear rot disease), field inoculations and disease severity ratings occurred as described in Objective 1b. In this experiment, however, F. verticillioides was inoculated, and samples were analyzed for FB1 and FB2 contamination. Sample preparation was the same as for Objective 1b. During both years, inoculated ears from each replicate plot were pooled, as well as a group of non-inoculated ears in each plot. These samples were ground and analyzed for mycotoxin levels. The major mycotoxins associated with F. verticillioides are fumonisin B1 (FB1) and fumonisin B2 (FB2). FB1 and FB2 accumulation was measured through high pressure liquid chromatography – fluorescent detection (HPLC-FLD) following an immunoaffinity cleanup and naphthalene-2,3-dicarboxaldehyde (NDA) derivatization.
The relationship between cover crop legacy, ear rot severity, and fumonisin contamination will be analyzed using a two-way ANOVA in Rstudio with the p-value = 0.05. Mean comparisons of treatments will be made using a Tukey-HSD test. Regression models will be used to characterize the relationship between individual cover crop treatments and fumonisin contamination.
To address Objective 2 (To identify the interaction between cover cropping legacy, mycorrhizal colonization, and ear rot disease in successive maize) a greenhouse study was conducted. An experiment was conducted to determine the effect of mycorrhizal colonization on the severity of stalk rot disease in maize. In this experiment, one hundred and twenty short flowering maize plants (Early Sunglow Variety, Burpee) were planted in pots containing sterile soil, half of which were inoculated with 1.0g of a commercial arbuscular mycorrhizal fungi (AMF) inoculum, MycoGrow (Fungi Perfecti) placed beneath the seed at planting. This commercial inoculum contained six species of AMF fungi. Plants were allowed to grow until the V9-10 growth stages, and stalks were inoculated with pathogen treatments through a stab inoculation method as described in Objective 1a. Within each AMF treatment (+AMF and NoAMF), twenty plants were inoculated with F. verticillioides, twenty with F. graminearum, and twenty with a sterile PDA plug. After two weeks, stalks were harvested and lesion size was measured as stated in Objective 1a.
Results
Objective 1A
The effect of cover crop legacy on Fusarium Stalk Rot severity
When maize stalks which were grown in soil from different cover crop legacies were inoculated with F. verticillioides, mean lesion size was not structured by the cover crop treatment. Mean lesion size was however greater in plants inoculated with F. verticillioides than in stalks inoculated with a control treatment, regardless of cover crop treatment (Fig. 3). Stunting, chlorosis, and drought stress was observed in maize plants prior to inoculation (Fig. 4).
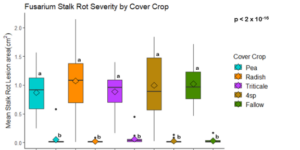
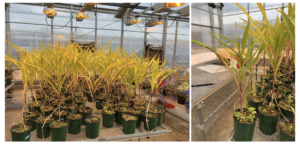
Objective 1B &1C
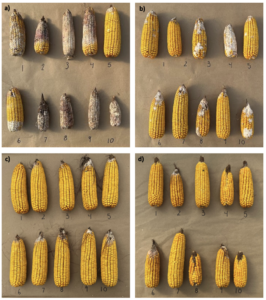
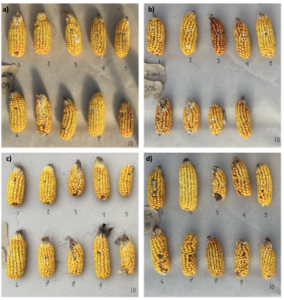
The effect of cover crop on fumonisin accumulation
Fumonisin accumulation was not structured by cover crop legacy (p > 0.05) in maize inoculated with F. verticillioides during 2021 or 2022 (Fig. 7-8). Fumonisin accumulation was also assessed in maize which remained uninoculated, and instances of no fumonisin accumulation were removed for analysis. This was done to assess fumonisin accumulation only in samples where natural Fusarium infection occurred. In naturally infected maize samples from 2021, the mean total fumonisin was higher in maize following a canola legacy than all other cover crop treatments with exception to Radish. Fumonisin accumulation was also higher in maize in a radish legacy when compared to pea, triticale, or fallow legacies. In naturally infected maize samples from 2022, the mean total fumonisin was higher in maize following a triticale legacy than those following canola, radish, or oat. Mean total fumonisin was also lower in naturally infected maize following a canola legacy than fallow in 2022 (Fig. 9b).
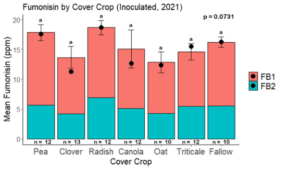
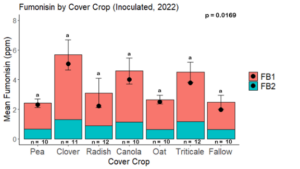
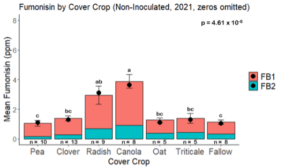
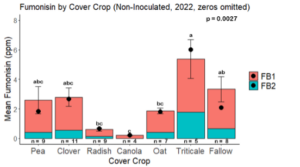
The effect of cover crop on trichothecene accumulation
While DON levels trended lower in maize following a clover or triticale legacy in maize inoculated with F. graminearum in 2021, DON levels were not significantly driven by cover crop legacy (p = 0.0790) (Fig. 11b). In 2022 however, DON was higher following a canola legacy in comparison to other cover crop legacies (Fig. 12). DON accumulation was also assessed in maize which remained uninoculated, and instances of no trichothecene accumulation were removed for analysis. This was done to assess trichothecene accumulation only in samples where natural Fusarium infection occurred. In 2021, DON was higher in maize following a radish legacy when compared to a canola legacy. In 2022, DON was higher in maize following a clover legacy than following any other cover crop legacy. Additionally, DON was higher following a fallow legacy when compared to a radish, canola, oat, or triticale legacy.
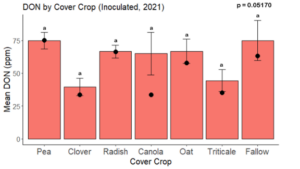
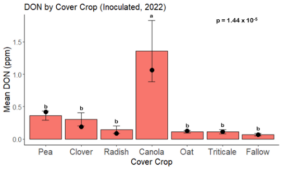
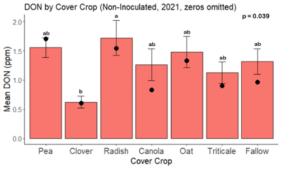
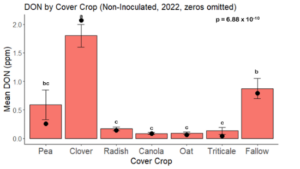
Objective 2
Mycorrhizal colonization may increase Fusarium disease severity.
A trend was observed that stalk rot lesion area was larger in maize plants grown without AMF in the soil in comparison to those grown in the presence of AMF for stalks inoculated with both pathogens (p = 0.38 for F. verticillioides and p = 0.61 for F. graminearum inoculated stalks) (Fig. 15). All pathogen inoculated treatments had a greater lesion area than those inoculated with a water control (Fig. 15)
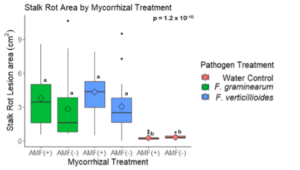
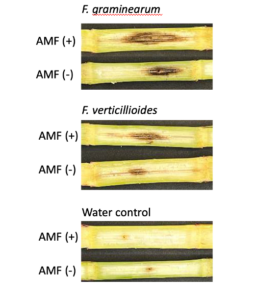
Discussion
Objective 1A
The effect of cover crop on stalk rot due to F. verticillioides was also assessed in a greenhouse experiment. In our study, maize plants were grown in cover crop soil in the greenhouse and inoculated while the plant was still living. Stalk rot severity was not structured by cover crop legacy in our study (Fig. 3). However, all plants were severely stunted and nutritionally deficient in this experiment which likely greatly influenced their susceptibility to stalk rot disease. Therefore, it is difficult to draw any conclusions from this experiment. Future stalk rot experiments could be performed in the field to avoid nutrient deficiencies and stress associated with growing maize in field soil in a greenhouse environment.
Objective 1B & 1C
Overall, F. graminearum and F. verticillioides ear inoculations were more successful in 2021 than in 2022. Regardless of cover crop treatment, disease severity and mycotoxin accumulation for both diseases were greater in inoculated samples over non-inoculated samples and water controls in 2021 but were not significantly different from the controls in 2022. This year-to-year difference was likely attributed to differences in inoculation strategy and environmental conditions between the two years. Our study found little success when toothpick inoculation was implemented in 2022. This was likely due to improper conditions at the time of inoculation, as Rock Springs, PA experienced drought conditions (higher than average heat and little precipitation) during the time of inoculation and in the following weeks. Toothpick inoculation strategies offer the inoculum little protection from the external environment and therefore, the inoculation success is more sensitive to poor environmental conditions when compared to a silk channel method. To manage the tradeoffs in either method for future experimentation, I would suggest either using the silk injection method as performed in 2021, but reducing the amount of inoculum injected, or inserting a colonized toothpick directly into the silk channel, which would reduce inoculum pressure, but allow for environmental protection from the husk.
While cover crop affected mycotoxin accumulation in some combinations of inoculation treatment and year, the result was not consistent across inoculation treatment or year. Cover crops which had reduced mycotoxin accumulation in one year seemed to increase ear rot severity and mycotoxin accumulation in the following year, and vice versa. For example, in 2021, when ears were inoculated with F. graminearum DON trended lowest following clover and triticale, while in 2022 DON was highest following canola (Fig. 11 – 12). Likewise, in naturally infected (non-inoculated) ears, DON was lowest following clover in 2021, but highest in 2022 (Fig. 13 – 14). Similar conflicting results were seen for Fusarium ear rot in naturally infected maize, as fumonisin accumulation was highest following brassicaceous cover crops canola or radish 2021, but lowest following either of these cover crops in 2022.
A possible explanation for variability in the effect of cover crop species legacy on disease between seasons is the interference of multiple confounding variables which obscure the impact of the cover crop treatment. Variables which could be impacting the results of this study include inconsistent plot environments, weed presence, irregular climatic conditions, and limitations due to small sample size and sampling error. A few major limitations of the experimental site were observed over the course of the study. The Cover Crop Cocktail (CCC) experimental site has a complex experimental layout containing 144 main treatment plots (12 cover crop treatments x 4 replicates x 3 entries) that are each 24 m x 27 m. Due to the topography of this experimental site, soil type and elevation varied across experimental plots. This led to variability in soil compaction and drainage across plots which was not attributed to the cover crop treatment. Aspects of soil type like aggregation and pore connectivity can influence soil factors like water retention, nutritional availability, and soil microbiome, which can all impact both plant health and plant defense. The experimental site is also managed as a fully organic trial, and as a result experiences high weed pressure. Most notably, there was a high level of wheat emerging as a weed in cover crop plots in both years. Therefore, each cover crop treatment is not a true monoculture, and in some cases would resemble more of a two species mixture with wheat, further interfering with the effects of the intended cover crop treatment.
Objective 2:
The results of this study suggest that AMF colonization may increase stalk rot disease severity under greenhouse conditions. During the greenhouse trial, a trend was observed that mean stalk rot lesion area was greater in plants with AMF compared to those without AMF when inoculated with either F. verticillioides (p = 0.38) or F. graminearum (p = 0.61) (Fig. 15). Fusarium pathogens have been associated with the salicylic acid (SA) mediated defense pathway in other research (Jung & Martinez-medina, 2012). Because AMF has been shown to increase expression of defense compounds associated with jasmonic acid (JA) mediated plant defense, it is possible that the result seen in this experiment is due to trade-offs between the expression of multiple defense pathways. Further investigation of the role of AMF in maize resistance to Fusarium pathogens should include the analysis of JA, SA, and other defense-related compounds to distinguish the effects of defense activation from other factors.
It is important in mycorrhizal research to confirm colonization rates in experimental treatments, as other factors can influence mycorrhizal colonization, including viability of inoculum (if inoculated with AMF), host genetics, and soil dynamics. Unfortunately, I was unable to observe or quantify mycorrhizae in the AMF-treated roots. If this experiment were to be repeated, it would be recommended to use inoculum from cultures maintained by The University of Kansas’s International Collection of (Vesicular) Arbuscular Mycorrhizal Fungi, INVAM (Sidney L Stürmer et al., 2021). Additionally, improving AMF confirmation via staining or molecular identification would be ideal for future experimentation. The sample size was low in this greenhouse experiment, as each treatment (AMF x Pathogen) was only n = 20. Repeating this experiment with a larger sample size could improve the statistical power of the experiment and reveal whether this trend holds over an experimental repeat.
The effect of cover crop legacy on stalk rot and mycotoxin accumulation is inconclusive. While cover crop structured mycotoxin accumulation in specific combinations of inoculation treatment and year, the result was not consistent across inoculation treatment or year. Future research must focus to minimize the confounding variables associated with field site, inoculation strategy and annual weather patterns. My recommendation to farmers would be that cover crop species does not seem to influence mycotoxin accumulation in maize due to Fusarium infection, and that cover crops should be selected based on other ecosystem service considerations. AMF colonization on the other hand, may increase stalk rot disease severity under greenhouse conditions, however additional research must be conducted to confirm this in the field. While this thesis work has specifically focused on the ability of different cover crop species to drive AMF colonization, many other crop management strategies can affect AMF colonization, such as tillage, fertilization, and pesticide use. Understanding the role of AMF in plant health and disease defense is important not only to inform cover cropping practices but a multitude of crop management practices. These findings could provoke further investigation into the role of AMF colonization in the management of maize foliar fungal pathogens.
Education & Outreach Activities and Participation Summary
Participation Summary:
Results from this research have been shared with a broad audience of stakeholders through various presentations over the course of this research. This research was presented during a fifteen-minute oral presentation made for other plant pathologists at the Northeast Regional American Phytopathological Society (APS) meeting in 2023, as well as a thirty minute presentation at the national APS meeting, where Ryan presented this research during a prestigious symposium highlighting the work of five students annually. By sharing our results with the research community, we hope to add to the knowledge base on the ecosystem services provided by cover cropping systems and promote further study of the relationship between cover crops and plant pathogens.
Additionally, poster presentations of this research were displayed at Regional and National APS meetings, various Penn State Research Expos, and The Penn State Agricultural Board of Trustees Election in 2023. By presenting this research at the Board of Trustees meeting, Ryan was able to share his research with Farm Bureau members and policy members across the state, including the Pennsylvania Secretary of Agriculture, Russel Redding.
This research has also been shared through extension activities including a Cover Crop workshop hosted at the Penn State Cover Crop Cocktail field site, where Ryan presented these findings to an Audience of farmers and crop consultants interested in organic field cropping. Ryan has also began a new role as a Penn State Extension Educator, where he will include this research in his programming, which includes doing presentations for local stakeholders, hosting cover crop workshops, and writing newsletter articles.
A journal article will also be published for this work (In progress).
Project Outcomes
While our research did not find a strong correlation between cover cropping systems and mycotoxin accumulation over the course of the two year research period, we met major limitations due to field level and climatic variation. This work is one of a very limited few studies on the impact of cover crop on above ground disease in cash crops. Research on the impact of cover cropping systems on below ground pathogens and insect pests is more common, and we hope that this research can inspire more investigation on the implications of cover crops for plant disease management.
Our research indicating potential tradeoffs of AMF colonization for increased susceptibility to Fusarium are also novel, and enhance our understanding of the ecological complexities underlying practices that modify the soil environment, including cover crop practices. While the adoption of cover cropping in Pennsylvania is high, species selection is often based on agronomic decisions and little is understood about how these different crops impact biological soil factors. By striving to improve our understanding of the ecosystem services provided by cover cropping, we can improve both adoption and our understanding of how to sustainably utilize cover crops for maximum benefit.
Over the course of this project we greatly enhanced our knowledge of the agronomic and pest management challenges associated with organic and no-till production systems, and further realized the necessity of research to address these challenges. While weed management challenges greatly affected our ability to research the effects of individual cover crops on disease in this study, this also revealed to us a great challenge in organic production systems- striking the balance between managing crops, weeds, disease, and reduced tillage without the use of conventional chemicals. It is clear that more research must be conducted to develop management tools for these farmers. Additionally, as significant mycotoxin contamination was found in naturally infected organic grain during our research, it continues to be a necessity to develop mycotoxin management strategies accessible to organic operations.
This research assisted in my completion of a Master's Degree in Plant pathology, and I have since begun a career in Extension, as a Penn State Extension Agronomy Educator. In my new role, I have the opportunity to share the findings of my work and my understanding of mycotoxins, cover crops, and organic production with Pennsylvania farmers and agricultural industry.