Final report for GS16-165
Project Information
Seaweed production in the United States has gained attention among farmers and scientists alike due to the high nutritional value, bioremediation properties and potential for integrated aquaculture systems with fish, shrimp and shellfish. The northern Gulf of Mexico is an ideal location for seaweed aquaculture to provide oyster farmers with a secondary product and to reduce eutrophication in the surrounding ecosystem. A variety of macroalgae species found in Alabama were evaluated in terms of their suitability for culture. The candidate species include, Ulva fasciata, Grateloupia taiwanensis, Hydropuntia secunda (formally Gracilaria secunda), and Chondria littoralis. Each species was cultured from an algal seed stock and grown on suspended longlines in coastal Alabama waters at two different sites. Seasonal algal growth rates were compared between open water culture sites and sites around oyster cages, for all four tested species. Nutrient composition of the cultured seaweed was analyzed to determine the nutrient removal capacity and nutritional value. The outcome of this work was presented to oyster farmers, chefs, scientists and consumers to investigate the market acceptance and potential for seaweed aquaculture on the Gulf Coast as a standalone farm or integrated system with oysters.
Objective 1. Compare the growth rate of Ulva fasciata, Grateloupia taiwanensis, Hydropuntia secunda, and Chondria littoralis cultured in the vicinity of oyster cages and in the open ocean.
Objective 2. Compare variations in the seasonal growth rate of Ulva fasciata Grateloupia taiwanensis, Hydropuntia secunda, and Chondria littoralis across each season.
Objective 3. Evaluate the nutrient removal efficiency and nutritional value of each species.
Objective 4. Present the results to oyster farmers, chefs, scientists and consumers to assess the market acceptance and potential for seaweed aquaculture on the Gulf Coast.
Cooperators
Research
1.0 Materials & Methods
1.1 Study area
This study was conducted on the Alabama coast, around Mobile Bay and the Mississippi Sound (Fig. 1). Acclimation, seeding and tank experiments were conducted at Auburn University’s Shellfish Laboratory (AUSL) on Dauphin Island, AL Experiments at sea took place at two oyster farms; Point Aux Pins LLC located in Sandy Bay, AL (30°22'58.9"N 88°18'47.6"W) and Massacre Island Oyster Ranch located off of Dauphin Island, AL (30°15'10.1"N 88°10'04.8"W). These two oyster farms were chosen based on their contrasting environments; the Sandy Bay site is more protected from wave action and has higher turbidity with a muddy bottom, whereas the Dauphin Island site is more exposed, with stronger wave action, clearer water and a sandy bottom.
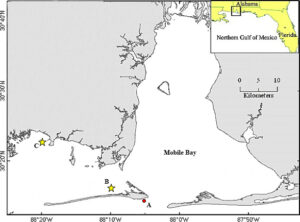
1.2 Objective 1
The four species of macroalgae, Ulva fasciata, Grateloupia taiwanensis, Hydropuntia secunda, and Chondria littoralis were collected from Perdido Pass, AL and transported in insulated coolers of ambient seawater to AUSL. All species were verified by DNA barcoding at Dr. Lopez-Bautista’s PhycoLab at the University of Alabama. For the red algae species, 1.2 kg wet weight was collected to initiate culture at sea via fragmentation, while for U. fasciata, only ~15 g wet weight was collected as this species was cultured via sporulation. Upon arrival to the laboratory, the samples were cleaned gently in freshwater to remove epiphytes. The samples were acclimated in a flow through tank for around two weeks prior to starting the experiments. Problems arose collecting a sufficient amount of H. secunda (minimum 1.2 kg wet weight) for an experimental trial, and therefore results on H. secunda will not be discussed.
Temperature and salinity at AUSL was measured daily throughout the experiment using a handheld YSI Pro30 Conductivity, Salinity Instrument (Yellow Springs Instruments, Yellow Springs, Ohio). In-Situ Inc. Aqua TROLL 600 Multi-Parameter Sondes measured temperature and salinity at Point Aux Pins LLC in Sandy Bay, AL and Massacre Island Oyster Ranch located off of Dauphin Island, AL for the duration of the field experiments.
All statistical analyses were done using R Studio version 1.1.456 of the statistical programming language, R (R Core Team, 2017). All data was analyzed for normality using the Shapiro-Wilk normality test, where P > 0.05 indicates a normal distribution. Results for C. littoralis growth inside and outside the oyster farm were analyzed using a One-Way Analysis of Variance (ANOVA), where P < 0.05 indicates significantly different growth. Data on growth of U. fasciata discs cut from three locations was considered not normal by the Shapiro-Wilk normality test (P < 0.05) and therefore a Kruskal-Wallis rank sum test was used. When significant differences (P < 0.05) were found, significant differences between groups was determined using the Dunn (1964) Kruskal-Wallis multiple comparison test with p-values adjustment by the Benjamini-Hochberg method.
1.2.2 Red algae species
Both red algae species (C. littoralis and G. taiwanesis) were cultured by similar methods, involving the following sites: fragmentation, insertion or attachment of the fragments into rope, culture of the ropes at two oysters farms, and assessment of growth after 4 weeks and 6 weeks (Fig. 2). For C. littoralis, fragment bundles of 10 g were tied with 150 mm cable ties and inserted into 12.7 mm diameter polypropylene rope, with 10 bundles evenly distributed across every 1 meter of rope (Fig. 3, left). For G. taiwanensis individual fragments (100 g in total) were inserted into the polypropylene rope and evenly distributed across 1 meter in length (Fig. 3, right). For both species, 6- 1 m rope fragments were tied across a 1 x 1 m floating PVC (50.8 mm diameter) raft. At each oyster farm, rafts were anchored 100 mm below the water surface, with one raft per species adjacent to the oyster cages and a second raft per species anchored 30 m upstream from the farm (Fig. 4). The rafts were deployed on February 17th, 2017 at both oyster farms. After four weeks, March 17th, half of the ropes were harvested (3 ropes cultured inside the oyster farm and 3 ropes cultured upstream from the oyster farm) and after an additional two weeks the remaining ropes were harvested (Fig. 4). The daily growth rate (DGR) was calculated using the formula: DGR (% day-1) = [(Wf/Wi)1/t – 1] x 100 (Hiraoka & Oka, 2008; Mantri et al., 2011), where Wf is the final wet weight per meter, Wi is the initial wet weight per meter (100 g) and t is the days of cultivation (28 or 42 days).
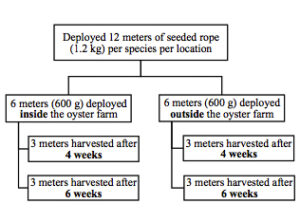
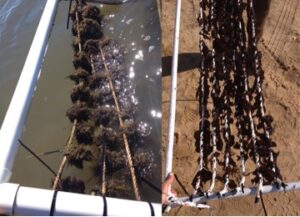
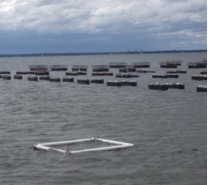
1.2.3 Ulva fasciata
Culture of U. fasciata involved evaluating differences in spore release and growth of spores between discs exercised at three thallus locations and covered three stages of production: sporulation, nursery, and grow-out (Fig. 5).
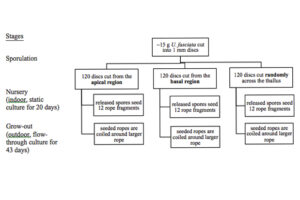
1.2.3.1 Sporulation
Spore release was induced by cutting blades (17 – 14 mm in length) into 1 mm discs using a Stiletto apparatus (Harris Uni-core, USA) at three different locations; “basal” region located near the holdfast, “apical” region located along the thallus margin, and “mixed”, discs cut randomly across the thallus (Fig. 6). For each blade position, 120 discs were cut. The discs were incubated in Petri dishes (10 discs per dish) with 10 ml of filtered seawater (1 µm) and F/2 media (0.129 ml/L). To prevent the growth of diatoms in the culture, a saturated solution of germanium dioxide (250 mg GeO2 in 1 L deionized water) was added at a concentration of 2 ml/L of enriched seawater prior to adding the discs (Redmond et al., 2014). The dishes were kept under cool, white florescent lighting (100 µmol photons m-2 s-1) and on a 12 h L : 12 h D photoperiod (Fig. 7). The temperature and salinity was maintained at 22.4 ± 0.4 °C and 21.7 ± 0.4 ppt respectively. Additional filtered seawater was added to account for evaporation during sporulation.
Photographs of the excised discs were used to measure the rate of sporulation every 12 hours for a total of 60 hours. For each location on the thallus (basal, apical, mixed), 60 discs were photographed at every time point using a phase contrast microscope at 100x magnification (Motic BA410). Sporulation was evaluated based on the percentage of the disc that was transparent, indicating that the vegetative spores had been released and only the transparent cell wall remained. Sporulation was rated on a 6-point scale as follows: 0%, 1-20%, 21-40%, 41-60%, 61-80%, 81-100%.
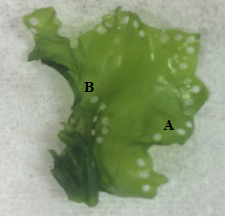
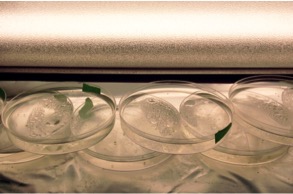
1.2.3.2 Nursery Culture
After 60 hours, the dishes of released spores were used to seed the rope fragments with one dish (10 discs) per rope fragment. Twelve polypropylene rope fragments (0.8 mm in diameter, 1.75 m in length) were seeded for each thallus location (apical, basal, mixed) and were attached with sticky tack to completely cover the base of a plastic 10L tank (Fig. 8). The tanks were filled with 4 L of filtered seawater (1 µm) and F/2 media (0.129 ml/L). This amounted to twelve dishes of released spores per plastic tank and a total of 12 seeded rope fragments per thallus location. The tanks were kept under controlled laboratory conditions for two weeks, under white florescent lights and a 12 h L : 12 h D photoperiod at 25.3 ± 0.5 °C and 22.2 ± 0.6 ppt. The water was changed every two days for a total of 20 days.
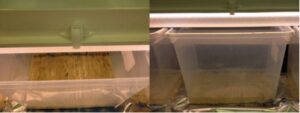
1.2.3.4 Outdoor Culture
After the nursery period, the rope fragments were carefully detached from the tanks and coiled around 12.7 mm diameter twisted polypropylene rope, 1 m in length (Fig. 8). The ropes were strung across a PVC raft positioned 100 mm below the water surface in an outdoor, flow-through 1,000 L tank (~787 L seawater, surface area of 2.2 m2) with natural lighting and heavy aeration (Fig. 9). The tank was drained daily and the ropes were rinsed gently with saltwater to reduce sedimentation and limit growth of opportunistic algae. The ropes were harvested after 43 days of outdoor culture and uncoiled off the twisted polypropylene rope. In order to not damage the thalli and to account for small fronds (1-3 mm), final wet weight was determined by gently drying each 0.8 mm diameter rope on paper towels, subtracting the average weight of the damp rope (2.03 g), and dividing by the length of the 1.75 m rope to obtain a wet weight per meter of rope (g FW m-1 rope). The daily growth rate (DGR) for each thallus location was calculated using the formula: DGR (% day-1) = [(Wf/Wi)1/t – 1] x 100 (Hiraoka & Oka, 2008; Mantri et al., 2011), where Wf is the final wet weight per meter, Wi is the initial wet weight per meter (~0.001 g) and t is the days of nursery and outdoor cultivation (63 days).
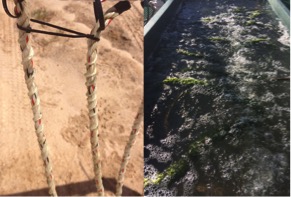
1.4 Objective 3
Samples of the tested species, G. taiwanensis and C. littoralis were sent to Auburn University’s Soil and Plant Laboratory for nutrient analysis. Depending on the species availability, samples were taken from the collection site (Perdido Pass, AL), after two weeks of acclimation at AUSL and after four weeks of culture at the oyster farms. Water samples from the two oyster farms and the AUSL flow-through tank were tested for nitrate, nitrite, ammonia and phosphorus by analytical technicians at the Dauphin Island Sea Lab.
1.5 Objective 4
Samples of the culture species were sent to a local chef to determine potential consumer acceptance. Personal recipes with U. fasciata were also tested with the local community.
2.0 Results
2.1 Objective 1
2.1.1 Red algae species
Growth of C. littoralis seeded lines was only observed at Point Aux Pins LLC located in Sandy Bay and culture of G. taiwanesis was unsuccessful at both sites (Sandy Bay and Dauphin Island). After four weeks of culture at Sandy Bay, C. littoralis achieved a mean DGR (% day-1) of 5.3 ± 0.1 % or 10.5 g FW m-1 day-1 inside the oyster farm and 5.1 ± 0.1 % or 9.8 g FW m-1 day-1 outside the oyster farm (Fig. 10B). After an additional two weeks, the DGR decreased to 4.3 ± 0.1 % inside the oyster and 4.1 ± 0.0 % outside the oyster farm. The difference in growth inside and outside the oyster farm was not significant at either sampling times (P > 0.05). Biofouling, primarily barnacles, was minimal for the first four weeks at Sandy Bay but increased by six weeks of culture (Fig. 10C). Bleaching, biofouling and overall loss of biomass was observed during attempts to culture G. taiwanesis at both oyster farms (Fig. 11) and C. littoralis at Dauphin Island (Fig. 10A).
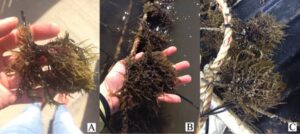
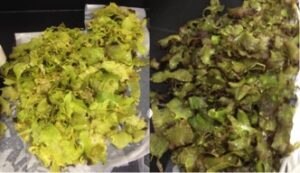
2.1.2 Ulva fasciata
From 12 to 60 hours of culture, the progression of spore release (measured from 0% to 81-100%) in exercised discs was successfully monitored (Fig. 12). The rate of spore release and number of discs at each stage varied depending on the location of the exercised disc (Fig. 13). At 12 hours, no evidence of sporulation was observed in 73% of discs cut randomly across the thallus, 63% of discs cut from the basal region and 60% cut from the apical region. Only one disc, cut from the apical region, had greater than 81% spore release at 12 hours after the initial cut. By 24 hours, there was a higher proportion of discs showing signs of sporulation, with no spore release found in 46% of discs cut from the basal region, 40% of discs cut randomly across the thallus and 38% of discs cut from the apical region. At 60 hours, 45% of the discs cut from the apical region and 35% of the discs cut randomly across the thallus showed 81-100% spore release. None of the discs taken from the basal region had above 80% sporulation and only 2% of the discs showed 61-80% spore release (Fig. 13).
During the nursery phase, daily temperature ranged from 21.5°C to 26.7 °C with a mean of 25.3 ± 0.6 °C. Salinity ranged from 20.3 to 29.5 ppt with a mean of 22.5 ± 0.7 ppt. At the grow-out phase, average daily temperatures began to drop as the end of fall and beginning of winter was approaching. Average temperature over the 43-day grow-out period was 19.6 ± 0.5 °C and ranged from a minimum of 14.9 °C to a maximum temperature of 23.4 °C. Average salinity was 22.7 ± 0.8 ppt and ranged from 14.6 to 27.4 ppt.
Seeded ropes in the outdoor culture system at AUSL steadily increased in biomass over the 43-day grow-out (Fig. 14). At completion of the grow-out period, growth was significantly greater in ropes seeded with discs cut from the apical region (0.58 ± 0.03 g FW m-1 day-1) than ropes seeded with discs cut randomly across the thallus (0.32 ± 0.02 g FW m-1 day-1, P = 0.014) and significantly greater than ropes seeded with discs cut from the basal region (0.04 ± 0.01 g FW m-1 day-1, P < 0.001) (Fig. 15-16). Additionally, ropes seeded with discs cut randomly across the thallus grew significantly faster than ropes seeded with discs cut from the basal region (P = 0.012) (Fig. 15-16). Daily growth rates (DGR % day-1) from the nursery period to the end of outdoor culture followed the same pattern, with an average 17.39 ± 0.12 % for ropes seeded with discs cut from the apical region, 16.29 ± 0.14 % for ropes seeded with discs cut randomly across the thallus, and 13.06 ± 0.18 % for ropes seeded with discs cut from the basal region.
Seeded ropes deployed at the oyster farms during spring 2017 were unsuccessful and were quickly taken over by filamentous algae (Fig. 17).
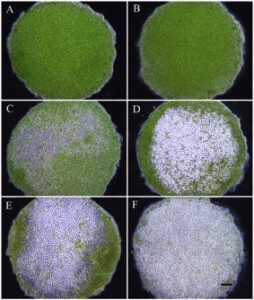
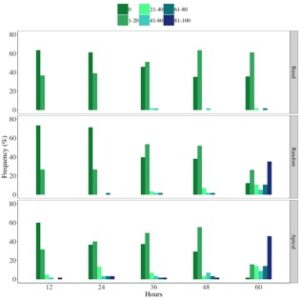
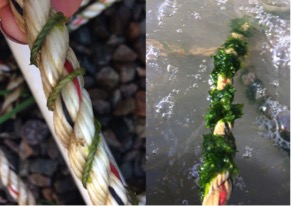
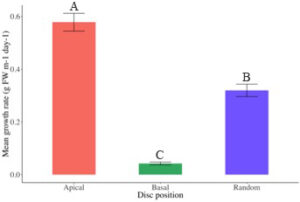
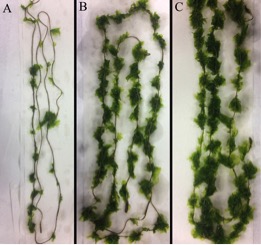
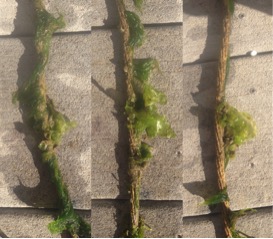
2.2 Objective 2
Out of the four potential species for culture, C. littoralis and U. fasciata were the most feasible. The species, G. taiwanensis, did not grow at either culture site and culture experiments with H. secunda were not possible as not enough wild biomass could be collected. From the species cultured, U. fasciata had the greater DGR with a maximum of 17.39 ± 0.12 % for ropes seeded with discs cut from the apical region compared to C. littoralis which had a DGR of 5.3 ± 0.1 % inside the oyster farm and 5.1 ± 0.1 % outside the oyster farm.
In terms of seasonal growth, wild C. littoralis was only found during the spring (February to May) and suggests one growing season. However, if cultures of C. littoralis were kept under controlled conditions over the hottest summer months, then culture in the fall might be feasible. Wild U. fasciata was abundant during the spring (February to May) and fall (September to November) and suggests the potential for two growing seasons. Initial experiments in the spring not included in this report, and the fall experiment described in Objective 1 support this hypothesis.
2.3 Objective 3
There was a high amount of variation in tissue nitrogen and phosphorus between sites, suggesting a relationship between tissue composition and condition (Table 1). The highest N/P ratios were observed at the collection site and represent the composition of healthy blades. When looking specifically at G. taiwanesis tissue samples, a similar N/P ratio was found at AUSL (13.81) compared to 18.20 at the collection site, Perdido Pass and both these growing sites had the healthiest looking blades (i.e. little to no bleaching). The lowest N/P ratios were observed at the Massacre Island Oyster Ranch off of Dauphin Island (2.96), which suggests that the site could be nitrogen limited. Evidence for nitrogen limitation is supported by the water samples, which show a low DIN (3.40 µM) compared to Perdido Pass 10.15 µM) and Point Aux Pins LLC in Sandy Bay (8.22 µM) (Table 2).
In terms of nutrient removal efficiency, unfortunately samples were unable to be collected for C. littoralis at Sandy Bay, AL and therefore an accurate assessment of nutrient removal cannot be made. However, we can estimate the nutrient removal efficiency if we assume that the percentage of nitrogen and phosphorus in C. littoralis at Sandy Bay is between that of tissue samples at Perdido Pass (3.56% nitrogen, 0.17% phosphorus) and Dauphin Island (1.86% nitrogen, 0.23% phosphorus), as the Sandy Bay site showed better growth than the Dauphin Island site but signs of bleaching unlike the Perdido Pass site. For instance, if we estimate that the percent nitrogen in C. littoralis tissue at Sandy Bay is 2.71% and the percent phosphorus in C. littoralis tissue is 0.20% then the approximate nutrient removal would be 28.46 g/m/day nitrogen and 2.10 g/m/day phosphorus inside the oyster farm and 26.56 g/m/day nitrogen and 1.96 g/m/day phosphorus outside the oyster farm. Nutrient removal efficiency cannot be calculated for G. taiwanesis as no growth was observed at either oyster farm.
One of the surprising outcomes of the analysis was the high level of arsenic found in seaweed samples from the collection site (Perdido Pass, AL), with 60 ppm found in G. taiwanesis and 34 ppm found in C. littoralis compared to less than 1 ppm at all other sites and for all other toxic metals such as, lead and cadmium. While these concentrations of arsenic seem alarming, it is important to understand the difference between highly toxic inorganic arsenic, and the potentially toxic or non-toxic organic arsenic commonly found in seaweed. The majority of arsenic taken up by seaweed is stored as arsenosugars, which is considered less toxic than inorganic arsenic, but still potentially toxic as arsenosugars react differently than harmless arsenobetaines (Feldmann & Krupp 2011). For most seaweed species, inorganic arsenic accounts for 15% of the total measured arsenic and normally below the acceptable 2 ppm (WHO 2011). There is currently no guidelines regarding the acceptable level of arsenic (inorganic and organic) in seaweed products in the United States, however, work is currently being done in the US and around the world in setting food regulations for seaweed-related products (Taylor et al. 2017). What is critical to note about the levels of arsenic in these species is that the concentrations appeared to be site specific, with only one of the four sites sampled having detectable levels. Therefore, when looking at the feasibility of culturing seaweed species for human consumption, the presence of arsenic and the influence of the culture site must be considered.
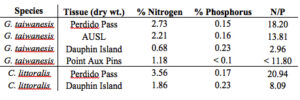

2.4 Objective 4
All four species were presented to the executive chef of Fisher’s Upstairs in Orange Beach, Alabama for sampling. Based on his feedback, the species, G. taiwanensis and U. fasciata, have the most potential to be sold to restaurants. The consumer acceptance of U. fasciata was additionally tested after the growth experiment in fall 2017, whereby the product of the experiment was turned into a seaweed salad and given to local residents alongside raw oysters (Fig. 18). The positive feedback of the seaweed salad further supports a potential market for the species.
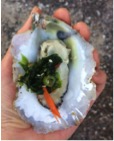
3.0 Discussion
One of the main conclusions from this work is that Alabama oyster farms do not appear to be suitable for culture of the three tested species (G. taiwanesis, U. fasciata and C. littoralis), with the exception of C. littoralis grown in Sandy Bay, AL (Point Aux Pins LLC). There are two likely explanations for the lack of growth: 1) lower and highly variable salinity at the oyster farms based on a comparison of the environmental conditions between sites and 2) increased herbivory, sedimentation and competition by opportunistic algae based on personal observations between the results at the oyster farms and productivity in the flow-through tank at AUSL.
Firstly, the salinity was lower at the oyster farms over the course of the spring (February to April) experiments compared to the collection site, with an average salinity of 21.8 ppt at Sandy Bay (Point Aux Pins LLC) and 23.1 ppt off Dauphin Island (Massacre Island Oyster Ranch) compared to 27.8 ppt in Perdido Pass. Between the two oyster farms, the daily salinity fluctuations were larger, on average, at Massacre Island Oyster Ranch than Point Aux Pins LLC and could help to explain the difference in growth of C. littoralis at these two sites. For example, from February 19th- 21st daily salinity, at Massacre Island Oyster Ranch ranged from a maximum of 25.6-22.4 ppt and a minimum of 15.6-12.9 ppt while daily salinity at Point Aux Pins LLC ranged from a maximum of 23.6-22.4 ppt and a minimum of 21.6-19.4 ppt. Salinity fluctuations lead to osmotic stress and require algal cells to use more energy to maintain a stable ionic composition, therefore leaving less energy available for growth (Lartigue et al., 2003; Nejrup & Pedersen, 2012; Kumar et al., 2014). Previous work in Mobile Bay, AL, by Lartigue et al. (2003), found that U. lactuca had reduced net oxygen production following sudden changes in salinity. Furthermore, feedback from experts following a presentation of this research at the World Aquaculture Society conference in June 2017 agreed that frequent changes in salinity was the likely cause of bleaching and subsequent mortality of the cultured seaweed.
Herbivory, sedimentation and competition with opportunistic algae are also potential causes of lack of growth, and likely occur together, whereby herbivory occurs first and makes room for filamentous algae and sediment to take over. This was particularly apparent for U. fasciata growth trials in the spring, as the ropes of small seedlings were easily taken over by filamentous algae and sediment within a few weeks. Extensive herbivory by grazing amphipods had been observed in seawater ponds in Florida culturing Gracilaria tikvahiae on ropes (Hanisak, 1987). Additionally, herbivory could have been increased due to the close proximity of the oyster farm. The structure of off-bottom oyster aquaculture and the growth of macroalgae/epifauna on the plastic mesh has been known to alter fish species abundance diversity by providing refuge from predation, habitat diversity and can attract larger, mobile fish by providing a food source (Forrest et al., 2009; Dumbauld et al., 2009; Powers et al., 2007). The impact of herbivory, sedimentation and competition is further supported by the results of rope cultures of U. fasciata in the flow-through tank at AUSL. Cultures at AUSL showed good growth and were free from noticeable herbivory, as well as, rinsed daily to reduce sedimentation and the growth of filamentous algae. Future experiments on seaweed rope culture at oyster farms could consider using cages instead of floating rafts in order to discourage herbivory and, similarly, culture practices at sites with high turbidity should involve daily rinsing to reduce sedimentation and growth of filamentous algae.
To determine the feasibility of each species for aquaculture in the northern Gulf of Mexico, biological criteria (growth rate, culture method, hardiness) and factors influencing market potential (appearance, texture, nutritional composition) were considered. Out of the three species tested, U. fasciata had the most potential for aquaculture. In terms of the first biological trait, growth rate, the DGR (% day-1) for U. fasciata cultured from discs cut from the apical region (17.39 ± 0.12 %) was comparable to a previous study on U. fasciata, which reported a DGR of 16.1 ± 0.28% when grown under ambient temperature and salinity (24°C, 30 ppt) in a static culture for 10 days (Mantri et al., 2011). However, growth rates were lower than studies on tubular, filamentous Ulva species. Carl et al. (2004) reported a maximum DGR of 67.1 ± 12.2 % for Ulva sp. 3 after 7-10 days of cultivation and Hiraoka & Oka (2008) reported a maximum DGR of 54.5% for U. prolifera after 7 days of cultivation at 22°C. The slow growth in this study could be attributable to the time of year the experiment took place, resulting in cooler water temperatures (average 25.3°C for nursery, 19.6°C for outdoor culture). Faster growth is likely earlier in the season at average temperatures around 24-25°C, similar to the temperature range used in Mantri et al. (2011). Additionally, the length of the nursery period can have significant effects on the growth rate, as Carl et al. (2004) observed with Ulva sp. 3 that an extended nursery period (10 days) does more harm than good due to the limited resources under static culture. However, Carl et al. (2004) was using a faster growing filamentous species, and the optimum length of the nursery period will need to be determined for U. fasciata.
In terms of the other biological criteria, the efficiency of the seeding technique for U. fasciata could be well adapted to large-scale production, but the species lacked hardiness when it came to culture at the oyster farms. As a result, while the species has potential for commercial culture, more suitable growing environments need to be considered. In terms of market potential, the species had an attractive appearance and texture, with thick blades that are more desirable than other Ulva species such as U. lactuca. Nutrient analysis of U. fasciata was not possible in this study, however reports on a similar species, U. lactuca, have reported 27.2% protein, 60.5% dietary fiber in dry weight and a good source of essential amino acids (Ortiz et al. 2006). Similarly, a study comparing four Ulva species (U. lactuca, U. pertusa, rigida, and U. reticulata) found protein levels (% dry weight) to be from 10-26% and dietary fiber (% dry weight) to be from 29-65.7% (Pereira, 2011).
Out of the three species tested at the oyster farms, C. littoralis was the only species that grew, suggesting a superior hardiness and tolerance to the conditions common of oyster farms in the northern Gulf of Mexico. The disadvantage of the culture methods for C. littoralis was the large amount of wild biomass (1.2 kg) needed to initiate culture by fragmentation. This limitation also prevented growth trials of H. secunda as not enough biomass could be collected. If more efficient culture methods were developed, such as methods similar to the seeding technique for U. fasciata, then the species would be more suitable for large-scale production. Furthermore, despite the fast growth rates and hardiness of C. littoralis, there was little market interest in this species. The species has an undesirable appearance and texture for human consumption. Nevertheless, the species has potential as a source of protein (22.3% crude protein dry weight at collection site) in nutritional supplements or animal feed. C. littoralis could also be cultured for its antibiotic properties as it has been found to contain a complex called chonalgin which is similar to the sarginin complex originally isolated in Sargassum natans (Martinez-Nadal et al., 1964). The complex is know to have strong antimicrobial properties against Gram positive, Gram negative bacteria and fungi and chondalgin, specifically, can control E. coli and C. albicans (Hernandez, 1967; Martinez-Nadal et al., 1964). While there is commercial potential for C. littoralis, markets would need to be established in order for production to be feasible.
The last species tested, G. taiwanesis, was the most promising for the edible seaweed market. The species has long, thick blades similar to several edible kelp species and could readily be accepted into the growing U.S. kelp market. The local production of kelp and rising market in the United States has developed over the past 12 years, since the first open-water kelp farm was established in the Gulf of Marine in 2006 (NPR, 2012). As kelp is a cold-water species, the culture of G. taiwanesis, could be a suitable alternative for farmers in the northern Gulf of Mexico and an opportunity to join this exciting new market. However, the culture sites tested in this study were unsuccessful and more research needs to be done to determine the suitable growing conditions. Stocks in the outdoor tank at AUSL could be maintained without significant signs of bleaching and therefore land-based, flow-through culture of this species might be the most efficient method of culture.
4.0 References
Carl, C., de Nys, R., Paul, N.A. 2014. The Seeding and Cultivation of a Tropical Species of Filamentous Ulva for Algal Biomass Production. PLoS ONE 9(6): e98700.
Dumbauld, B.R., Ruesink, J.L., Rumrill, S.S. 2009. The ecological role of bivalve shellfish aquaculture in the estuarine environment: a review with application to oyster and clam culture in West Coast (USA) estuaries. Aquaculture, 290: 196-223
Feldmann, J., Krupp, E.M., 2011. Critical review or scientific opinion paper: Arsenosugars—a class of benign arsenic species or justification for developing partly speciated arsenic fractionation in foodstuffs? Analytical and Bioanalytical Chemistry 399, 1735–1741.
Forrest, B.M., Keeley, N. B., Hopkins, G. A., Webb, S. C., Clement, D. M. 2009. Bivalve aquaculture in estuaries: Review and synthesis of oyster cultivation effects. Aquaculture. 298: 1-15.
Hanisak MD (1987) Cultivation of Gracilaria and other macroalgae in Florida for energy production. In: Bird KT, Benson PH (eds) Seaweed cultivation for renewable resources. Developments in aquaculture and fisheries science, 16. Elsevier, Amsterdam, pp 191–218
Hiraoka, M., Oka, N. 2008. Tank cultivation of Ulva prolifera in deep seawater using a new ‘‘germling cluster’’ method. Journal of Applied Phycology. 20: 97–102.
Hernandez, A. T. 1967. Presence of sarganin complex in members of the family Sargassacea. Bios. 38: 32-36.
Kumar, M., Kumari, P., Reddy, C., & Jha., B., 2014. Chapter four - Salinity and desiccation induced oxidative stress acclimation in seaweeds. Advances in Botanical Research. 71: 91–123.
Lartigue, J., Neill, A., Hayden, B. L., Pulfer, J., & Cebrian, J., 2003. The impact of salinity fluctuations on net oxygen production and inorganic nitrogen uptake by Ulva lactuca (Chlorophyceae). Aquatic Botany. 75: 339–350.
Mantri, V.A., Singh, R.P., Bijo, A.J., Kumari, P., Reddy, C. R. K., Jha, B. 2011. Differential response of varying salinity and temperature on zoospore induction, regeneration and daily growth rate in Ulva fasciata (Chlorophyta, Ulvales). Journal of Applied Phycology. 23: 243-250.
Martinez-Nadal, N.G., Rodriguez, L. V., Casillas, C. 1964. Sarganin and chonalgin, new antibiotic substances from marine algae from Puerto Rico. Antimicrobial Agents and Chemotheraphy, American Society of Microbology. 68-72.
Nejrup, L. B., Pedersen, M. F., 2012. The effect of temporal variability in salinity on the invasive red alga Gracilaria vermiculophylla. European Journal of Phycology. 47: 254-263.
NPR. 2012. Kelp for farmers: Seaweed becomes a new crop in America. https://www.npr.org/sections/thesalt/2012/10/12/162728509/kelp-for-farmers-seaweed-becomes-a-new-crop-in-america Accessed: Aug. 10th 2018.
Ortiz, J., Romero, N., Robert, P., Araya, J., Lopez-Hernandez, J., Bozzo, C., Navarrete, E., Osorio, A., Rios, A. 2006. Dietary fiber, amino acid, fatty acid and tocopherol contents of the edible seaweeds Ulva lactuca and Durvillaea antarctica. Food Chemistry. 99: 98-104.
Pereira, L. 2011. Chapter 2: A review of the nutrient composition of selected edible seaweeds. Seaweed: ecology, nutrient composition and medicinal uses. Nova Science Publishers Inc. pp. 15-47.
Powers, M.J., Peterson, C.H., Summerson, H.C., Powers, S.P. 2007. Macroalgal growth on bivalve aquaculture netting enhances nursery habitat for mobile invertebrates and juvenile fishes. Marine Ecology Progress Series, 339: 109-122.
R Core Team. 2017. R: A Language and Environment for Statistical Computing.
https://www.R-project.org/
Redmond, S., L. Green, C. Yarish, J. Kim, and C. Neefus. 2014. New England seaweed culture handbook- nursery systems. Connecticut Sea Grant CTSG-14-01. 92 pp. Accessed online 8/13/18: http://digitalcommons.uconn.edu/seagrant_weedcult/1
Taylor, V., Goodale, B., Raab, A., Schwerdtle, T., Reimer, K., Conklin, S., Karagas, M.R., Francesconi, K.A., 2017. Human exposure to organic arsenic species from seafood. Science of The Total Environment 580, 266–282.
WHO (2011) Safety evaluation of certain contaminants in food/ prepared by the Seventy-second meeting of the Joint FAO/WHO Expert Committee on Food Additives (JECFA). 63. World Health Organization and Food and Agriculture Organization of the United Nations, Geneva. http://whqlibdoc.who.int/publications/2011/9789241660631_eng.pdf. Accessed 22 July 2018
Educational & Outreach Activities
Participation Summary:
Three oyster farms (Point Aux Pins LLC, Massacre Island Oyster Ranch, Pensacola Bay Oyster Co.) participated in this research, providing space on their farm and farmers assisted with deployment and sampling. Only two farms (Point Aux Pins LLC and Massacre Island Oyster Ranch) were discussed in the results as trials with Pensacola Bay Oyster Co. were unsuccessful due to unusually low salinity.
Results from the fall 2016 and spring 2017 experiments were presented at the World Aquaculture Society conference in June 2017.
Project Outcomes
This project provides an initial analysis of the potential of seaweed aquaculture in the Gulf of Mexico and aims to spark more interest on the subject. We have received encouraging feedback and interest from farmers, consumers and researchers in starting seaweed farms, polyculture systems at oyster farms and businesses for seaweed products along the Gulf coast. The evaluation of the four species tested in this project in terms of suitability for culture can be used as a framework to base future research off of.
The project was extended to attempt a crop of Ulva in 2019, working with oyster farmers in the Florida Panhandle where environmental conditions were believed to be more suitable (e.g., higher salinity and water clarity). A crop of Ulva was, in fact, cultured successfully and allowed to attach to lines. There was high initial growth and arrangements were made with two oyster farms in Florida to receive the seeded lines. State regulatory authorities, however, indicated that the farmers' existing permits did not allow the culture of anything other than shellfish. Based on this ruling, the importation and deployment of the Ulva was prohibited. The crop of Ulva subsequently died off in the nursery system before the permitting issue could be resolved. Despite this disappointing result, importantly the Florida Division of Aquaculture is actively revising current permits to allow the culture of native seaweeds on shellfish farms. We anticipate following up in 2020 with interested farmers to assess the grow-out of Ulva at several oyster farms in Florida.
Based on the results of this project, the potential for culture of at least one of these species on oyster farms in the region may be worth investigating in other parts of the region, where environmental conditions may be more suitable.