Final report for GW21-218
Project Information
Cirsium arvense (L.) Scop. (Canada thistle) is an aggressive perennial weed which threatens sustainable crop production. Organic producers throughout Montana and across the Western region have expressed the need for the development of integrated Canada thistle management programs which include mechanical, cultural, and biological control tactics. The fungal pathogen, Puccinia punctiformis (thistle rust), is a highly selective Canada thistle parasite that has the potential to aid in the suppression of Canada thistle when integrated with other agronomic management tactics. This research and education project will (1) explore methods to integrate thistle rust into tillage practices and cropping rotations to reduce the spread and impact of Canada thistle in organic settings, and (2) convey the generated information to organic and conventional farmers to facilitate the development of integrated weed management practices.
In this study, we evaluate in certified organic farms and experimental plots: (1) the impact of conventional and reduced tillage practices on the establishment and spread of thistle rust in Canada thistle populations, and (2) Canada thistle growth and spread within competitive cropping rotations. Research results have been used to inform producers who wish to adopt tillage and rotational tactics which maximize disease establishment and minimize the impact of Canada thistle. The ecological principles developed in this study will be useful to both organic and conventional growers, and our findings have been shared with agricultural stakeholders through extension meetings, grower conferences, and virtual content. The ultimate contribution from this project is the sustainable suppression of Canada thistle infestations within agroecosystems.
The overall goal of this study is to assess the integration of the Canada thistle pathogen, Puccinia punctiformis (thistle rust) to manage Canada thistle in organic agroecosystems.
- Research Objective 1: Assess how thistle rust responds to conventional and reduced tillage practices.
- Research Objective 2: Compare the impacts of crop competition in rust infected Canada thistle and non-infected Canada thistle.
- Education Objective 1: Extend knowledge of thistle rust and its integration into organic weed management.
Cooperators
- - Technical Advisor - Producer
Research
Research Objective 1: Assess how thistle rust responds to conventional and reduced tillage practices.
An integration of thistle rust as a Canada thistle biocontrol in agricultural systems requires a clear understanding of the pathogen's response to management tactics. Thistle rust spores remain dormant within Canada thistle debris on the soil surface until they come in contact with a new host. Soil tillage has the potential to bury, transport, or destroy thistle rust spores which are responsible for transmission of the pathogen. This objective is designed to examine the potential for tillage to impact the spread and establishment of the thistle rust pathogen.
Cooperator Farm
During the summer of 2020, producer Ole Norgaard cooperated in the establishment of a field study on his organically certified farm near Shonkin, Montana. The farm has pre-existing Canada thistle infestations which contain varying degrees of naturally established thistle rust infection. The preliminary study explored tillage impacts on thistle rust establishment and spread. At Mr. Norgaard's farm, four discrete Canada thistle patches were identified, flagged and GPS mapped. Two of the patches contained varying degrees of symptomatic rust infected Canada thistle, while the other two patches showed no signs of infection. Tillage occurred in one patch containing thistle rust infection and one patch with no signs of infection, using the cooperator’s tillage method of choice. The remaining two patches were left untilled throughout the rest of the growing season. Prior to tillage, vegetation growth data was collected along 20-meter transects, placed through the center of each thistle patch. Ten 1-m2 sample frames were placed at even intervals along the transects. Vegetation density data was collected for symptomatically infected thistle stems and total thistle stems within each frame. Additionally, percent vegetation cover was estimated within frames, including % symptomatically infected Canada thistle, % asymptomatic Canada thistle, % crop, % other, and % bare ground.
Mr. Norgaard decided to plant alfalfa as a perennial forage crop in the fall of 2020. During the 2021 growing season, sampling methods were repeated in within the same four discrete thistle patches that were monitored in 2020. Mr. Norgaard did not till the soil or swathe the field because the alfalfa crop was in the early to mid-vegetative stage of growth. Low moisture conditions in the summer of 2021 led to poor establishment of Mr. Norgaard's alfalfa crop, and an expansion of the Canada thistle populations within the fields. As a result, Mr. Norgaard decided to remove the fields from organic certification in the fall of 2021. The fields which hosted this study were sprayed with 123.5 oz./hectare of glyphosate in the fall, followed by seeding of a forage mixture.
As a response to the new management, the methods for the 2022 sampling period were altered to best accommodate the project objectives. The new objectives at the Norgaard Farm focused on re-evaluation of the original four thistle patches within the crop fields, with a new evaluation of thistle patches in a grazed pasture that borders Mr. Norgaard's crop fields. The goal was to determine if there were any differences in thistle density and the density of thistle stems with rust symptoms between the two habitat types at the Norgaard Farm. The crop fields were revisited in June 2022 where all four experimental thistle patches were sampled under previously established protocols. Three additional thistle patches, located within grazed pastures along Mr. Norgaard's crop fields, were also sampled using the original protocols. Canada thistle stem density and density of thistle stems with rust symptoms data were analyzed in RStudio using a generalized linear model with the gaussian distribution. Comparisons were made between thistle growth and rust symptoms from 2022 in the crop field habitat and the pasture habitat, and analysis was conducted in RStudio. The margin of each thistle patch was also mapped with an Emlid Reach RS2 GNSS receiver on June of 2022, and point vectors were taken at the location of all observations of symptomatically infected Canada thistle within each patch. The data was analyzed in QGIS on the WGS 84 Pseudo-Mercator coordinate reference system. Patch areas from each patch polygon was derived in QGIS, and RStudio was used to evaluate the difference in mean patch areas between the crop field habitat and the pasture habitat.
Montana State University Fort Ellis Research Farm
This study took place at the Montana State University Ft. Ellis Research Farm from 2020 to 2023 (LatLon: 45.667137, -110.977948). Fort Ellis is approximately 6-km east of Bozeman, Montana where the mean annual precipitation during the study period was 53-cm and the mean annual temperature was 6°C (PRISM Climate Group, 2022). The site is not irrigated, and has a 1.5-m deep Blackmore silt loam soil profile (USDA NRCS, 2019). Experimental plots at Ft. Ellis were 5.5-m wide and approximately 15.2-m long. At the beginning of the study, each plot contained three to four discrete Canada thistle patches of varying sizes and with varying degrees of naturally established Canada thistle and thistle rust infected stems. Patches were considered discrete when there was at least 2 meters of distance between patch boundaries, where no Canada thistle stems could be observed. The plots at Ft. Ellis were USDA organically certified in 2015, where they hosted reduced-till livestock grazing studies until 2017 (Lehnhoff et al., 2017; Larson et al., 2021). The plots remained fallow in 2018, followed by one year of a small-plot crop rotation and tillage study in 2019. All plots were uniformly cultivated with a chisel plow in the fall of 2019.
A randomized complete block design was used to evaluate the effects of tillage on Canada thistle and thistle rust over three growing seasons. There were two levels of tillage that were randomly assigned to plots and replicated four times each: standard tillage and reduced tillage. The standard tillage treatment level was mechanically disturbed with a tandem disc harrow (25-cm depth) and with a chisel plow (25-cm depth). The reduced tillage treatment level was mechanically disturbed with a flail mower, with grazing sheep (10 individuals), and with light surface disturbance using a chisel plow (1.5-cm depth). Tillage treatments were performed one to two times per growing season. To help reduce weed pressure, all plots were uniformly drill-seeded into a three-year green manure sequence that included: 1) 90-kgs/hectare of a spring barley in 2020; 2) 91- kg/hectare of winter pea intercropped with 84- kg/hectare winter triticale in 2021; 3) 90- kg/hectare of spring wheat in 2022; and 4) 90-kg/hectare of spring wheat in 2023.
The 2020 crop was seeded in early April, and terminated with assigned tillage treatments prior to grain maturity in early July. The plots remained in fallow until mid-September, when the assigned tillage treatments were repeated to prepare seedbeds for the winter crop, which was immediately seeded after seedbed preparation. In July 2021, the crop was terminated using the respective tillage treatments. Plots remained in fallow for the rest of the year, with one additional tillage treatment in October, 2021. Seeding in 2022 was delayed until late May, due to heavy rains that limited access to experimental plots. Prior to seeding in the spring of 2022, the plots were prepared with assigned tillage treatments. Crops were terminated in late August 2022 with respective tillage treatments. Prior to seeding in the spring of 2023, plots were again prepared using the assigned treatments, and were terminated in early August 2023.
Data Collection
All data were collected within one week prior to crop termination during each study year, which was approximately timed with the observable development of thistle rust teliospores on infected Canada thistle stems. Canada thistle patch boundaries were mapped as polygons with an Emlid Reach RS2+ GNSS receiver. Mapping was completed on the WGS84 geographic coordinate system, and was post-processed in ArcGIS Pro using corrections from the Montana State University GPS base station. GPS polygon data from 2020 was used to relocate discrete patches throughout the study.
To determine the effects of tillage on Canada thistle infected stem density and the growth rate of infected stems through time, a total count of Canada thistle stems with signs of Canada thistle infection were collected from within each discrete patch, during each year of the study. To evaluate the interaction between Canada thistle and Canada thistle infection within tillage treatments, stem counts were collected by randomly hand throwing 1-m2 quadrats within each discrete patch. The number of quadrats per patch was scaled based on patch size, to account for spatial variability of discrete Canada thistle patches within plots. Sample sizes ranged from three quadrats in patches with a small spatial area to nine quadrats in patches with a large spatial area. Within each quadrat, stem counts were taken from asymptomatic and symptomatic Canada thistle.
Data Analysis
To assess the effects of tillage on thistle rust infected stem density over three years, the total count of Canada thistle stems with signs of thistle rust infection per patch was modelled using a generalized linear model with a Poisson distribution (“glmer” function in the R-Package “lme4”). The fixed effects were tillage treatment, year, and the interaction between tillage and year. To account for repeated observations within the same Canada thistle patch and replication, a unique patch identity was included as a random effect. The estimated marginal means and standard errors were calculated for the interaction between tillage treatment and year using the “emmeans” function in R-Package “emmeans” (Lenth et al., 2023). Model selection was completed by comparing all potential models with a χ2 drop in deviance test. Assumptions of homoscedasticity, normality, and influential observations were visually assessed (Ramsey and Schafer, 2012).
The relative growth rate (RGR) of thistle rust infected stem density over three years was evaluated between tillage treatments using a linear model (“lm” function in R; Ramsey and Schafer, 2012), using the total count of infected stems per patch. The relative growth rate was calculated by subtracting the natural log of infected stem density per patch in 2022 with the natural log of infected stem density per patch in 2020, and dividing by the difference in time between 2022 and 2020 (Hoffman and Porter, 2002; Gurevitch et al., 2016). All assumptions were assessed using the methods previously described.
Additionally, Canada thistle stem density data from the 1-m2 quadrats was aggregated into mean Canada thistle density estimates for each discrete patch for each year of the study. The change in Canada thistle stem density (stems/m2) over time was evaluated, using a negative binomial mixed-effects model. The fixed effects were tillage treatments, year, thistle rust infected stem density, and the interaction between tillage treatments and each study year. Pairwise contrasts between tillage treatments and year were calculated using estimated marginal means. The random effect was patch ID. Model selection and assumptions were checked using methods previously described.
Research Objective 2: Compare the impacts of cropping management between rust-infected Canada thistle and non-infected Canada thistle.
The experiments under this objective consist of a greenhouse study and a field study that assess the effects of thistle rust on Canada thistle competitiveness and spread. The greenhouse study is designed to look directly at the growth responses of thistle rust inoculated Canada thistle when it is under stress from crop competition, and within a controlled environment. The field study is designed to evaluate the performance of the biocontrol agent in a setting that more closely represents a "real world" cropping system.
Montana State University Greenhouse
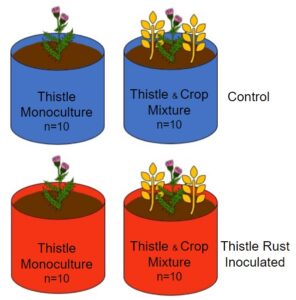
A greenhouse study with three independent trials was conducted at the Montana State University Plant Growth Center in Bozeman, Montana, between 2020 and 2022. A nested full factorial (2 x 2) design was used to assess the integration of thistle rust and crop competition. The primary treatment was thistle rust inoculation, with two levels: Canada thistle inoculated with thistle rust (n = 20) and non-inoculated Canada thistle grown as a control (n = 20). Nested within each level of the inoculation treatment was a competition treatment, split into two levels: Canada thistle grown in monoculture (n = 10) and Canada thistle grown in competition with a common crop species (n = 10; Figure 1).
The competition treatment was a four-phase crop sequence that incorporated common crops used by organic farmers in the dryland areas of the Northern Great Plains. The sequence included the following four phases, with seeding depths and seeding rates scaled for greenhouse pots: 1) Fallow: 1-gram Canada thistle rhizome planted at ~10 cm deep; 2) spring wheat: 100 kg/hectare planted at ~ 5 cm deep (18 plants/pot); 3) forage pea: 89 kg/hectare planted at ~ 5 cm deep (8 plants/pot); and 4) safflower: 33 kg/hectare planted at ~ 3 cm deep (2 plants/pot). Canada thistle rhizomes were planted in the approximate center of each pot during the first phase. Crops were planted in a manner that provided approximately equal space between individuals, with at least 5 cm of distance from pot edges.
Two separate greenhouse spaces were used to prevent movement of thistle rust spores between the thistle rust inoculated treatment and the non-inoculated (control) treatment. Internal greenhouse temperatures for both spaces were set at a range of 18°C to 26.5°C during the day, and 10°C to 24°C at night. To ensure consistent lighting, passive solar lighting with supplemental 1000-watt metal halide lamps, set to 12-hour intervals, were used throughout the course of the study.
Canada Thistle and Thistle Rust Establishment
Canada thistle rhizomes were acquired from naturally occurring populations in Gallatin County and Hill County, Montana during the summer of 2019. Rhizomes were maintained in greenhouse pots, and used as the source of rhizome transplants for the study. Pots (25.4 cm diameter x 20.3 cm deep) were sown with 1-gram cuttings of Canada thistle rhizome and randomly assigned to a treatment. Rhizomes were planted into a pasteurized soil mixture consisting of equal parts (by volume) of loam soil, washed sand, and Canadian sphagnum peat moss. Pots were watered every two days or as needed, for ten seconds per pot using the shower setting on a conventional garden hose wand. A soluble all-purpose fertilizer (20-20-20 NPK) was added to pots bi-weekly, by mixing 2.5 ml of fertilizer with 3.8 L of water in a watering can, and hand watering for ten seconds per pot. Canada thistle was grown for an average of 4.5 months during the first phase (fallow) in all treatments, which was approximately timed with the development of flower buds in all pots. In subsequent phases of each trial, Canada thistle was allowed to grow until harvest at the maturity stage of the crop within each crop phase.
Thistle rust inoculum was collected from naturally occurring populations of infected Canada thistle in Gallatin County, Montana and prepared as described by Berner et al. (2013). Systemically infected Canada thistle stems were harvested in the autumns of 2020 and 2021, and dried in paper bags in a dark room at ambient temperatures. From the dried stems, leaf tissue containing signs of teliospores were gathered, and ground into a coarse powder inoculum using a household blender. The ground teliospore-bearing inoculum was immediately used, or stored for future use in a -80°C freezer. Inoculation methodology followed Berner et al. (2013), where 5 ml of dry rust inoculum was dispersed evenly on the crowns of Canada thistle rosettes at the beginning of each phase, for a total of four inoculations per pot in each trial. The inoculated rosettes were misted with deionized water once a day for three days post inoculation to maintain humidity for spore germination. This method was repeated after the harvest of each phase and subsequent regrowth of Canada thistle, for a total of four inoculations per pot in each trial.
Data Collection
To address our first question, the density of Canada thistle stems with signs of systemic thistle rust infection was recorded from each pot at the termination of each crop phase. Canada thistle stems were identified as systemically infected when spore structures were observed on leaves and stems. To address our second and third questions, Canada thistle and crop stems were counted and cut at soil level at the termination of each crop phase. To obtain dry weight, the harvested biomass was oven dried for 72 hours at ~40.5°C and weighed to the nearest 0.01g. After each harvest, pots containing thistle rhizomes were left undisturbed and the next crop phase was seeded into pots assigned to the mixed competition treatment. After the aboveground harvest of final the crop phase (safflower) of each trial, Canada thistle rhizome biomass was removed from the soil of each pot, cleaned of soil and residue with cool water, dried for 72 hours at ~40.5°C, and weighed to the nearest 0.01g. Canada thistle pots assigned to the monoculture level of the competition treatment were harvested using the same methodology and at the same time as the mixed pots.
Data Analysis
The probability of observing systemic thistle rust infection in pots was calculated at each phase in the crop sequence, and was modeled using a generalized linear mixed effects model with a binomial distribution (“glmer” function in the R-Package “lmerTest”; Kuznetsova et al., 2022). The fixed effect in this model was crop phase, and pot ID was included as a random effect to account for repeated observations within each pot over the three trials. Model selection followed a backwards selection from a full model containing all potential explanatory variables using a ‘Drop in Deviance’ test (Ramsey & Schafer, 2012). Model over dispersion was checked by calculating the sum of squared Pearson residuals and comparing it to the residual degrees of freedom, and assumptions homoscedasticity, normality, or influential observations were visually assessed (Ramsey & Schafer, 2012).
The percentage of Canada thistle stems with signs of systemic thistle rust infection within the inoculated treatment was calculated out of the total density of Canada thistle stems per pot, and was modeled using a linear mixed effects model (“lmer” function in the R-Package “lmerTest”; Kuznetsova et al., 2022). The fixed effects and random effects in this model were the same as previously described. Explanatory variables were backwards selected from a full model containing all potential explanatory variables (“step” function in the R-Package “lmerTest”; Ramsey & Schafer, 2012). Model assumptions of homoscedasticity, normality, and influential observations were visually assessed (Ramsey & Schafer, 2012).
Differences in Canada thistle above- and belowground biomass was evaluated using separate linear mixed effects models. In the model for aboveground biomass, the fixed effects were inoculation treatment, competition treatment, and crop phase, with pot ID as a random effect. In the model for belowground biomass, the fixed effects were inoculation treatment and competition treatment, with trial as a random effect to account for repeated observations within each trial. In both models, explanatory terms were selected and assumptions were checked using methods described previously.
To assess the competitive ability of Canada thistle, a relative competition intensity (RCI; Weigelt & Jolliffe, 2003) was used to evaluate the impacts of competition between the thistle rust inoculated and non-inoculated (control) treatments was calculated as:
RCI = ((Monoculture - Mixed)/(Monoculture)) x 100
Where “monoculture” was the aboveground biomass of Canada thistle from the non-inoculated (control) monoculture treatment, and “mixed” was the aboveground biomass of the mixed pots for either the thistle rust inoculated or non-inoculated (control) treatment. RCIcontrol was calculated using aboveground biomass from the control monoculture and mixed pots that were not inoculated with thistle rust. RCIinoculated was calculated using aboveground biomass from the non-inoculated (control) monoculture and the aboveground biomass from the mixed pots in the thistle rust inoculated treatment. An RCI value ≤ 0 indicates that Canada thistle grown in mixed pots produced as much or more aboveground biomass compared to Canada thistle grown in a monoculture. In contrast, RCI > 0 indicates that aboveground biomass of Canada thistle was reduced when grown in mixed pots, and RCI = 100 indicates that no aboveground Canada thistle biomass was produced in the mixed treatment.
The relationship between RCIcontrol and RCIinoculated was evaluated using a linear mixed effects model, with fixed effects of inoculation treatment and crop phase, and pot ID included as a random effect. Model selection was completed by comparing all potential models with an Extra Sums of Squares F-Test. All model assumptions were visually assessed.
Montana State University Post Agronomy Farm:
The impacts of the thistle rust biocontrol on Canada thistle growth was evaluated at the MSU Post Agronomy Farm in Bozeman, Montana. The research fields at the site are each 0.02 hectares with a mean annual precipitation of 48 cm and a mean annual temperature of 7°C (USDA NRCS, 2019). This experiment combines biological, mechanical, and cultural controls for Canada thistle infestations within agroecosystems. During the spring of 2020, 1-gram cuttings of thistle rhizome were planted in two separate fallow fields. Each field contained 10 thistle rust inoculated and 10 non-inoculated Canada thistle. To reduce potential for unintentional thistle rust inoculation of the non-inoculated control treatment, the controls were intentionally planted in the predominant upwind direction, 12 meters away from the inoculated treatments. During the summer of 2020, both fields were maintained in fallow so that the planted rhizome could establish. Thistle growth data was collected in the fall within 1-m2 sample frames centered over the original rhizome planting locations. Data collection included thistle stem density, density of thistle stems showing rust symptoms, % cover asymptomatic thistle, % cover symptomatic thistle, % cover crop, % cover bare ground, and % cover other. Fall inoculations were performed during an optimal climate range, where temperatures were forecasted between 8 and 25°C. A maximum of five thistle rosettes were inoculated within 1-m2 sample frames centered over the original rhizome planting locations. Each inoculation consisted of a 5-mL application of thistle rust spores, applied directly to thistle rosettes. The spores were sourced from symptomatically infected thistle stems in Gallatin County, and prepared by grinding symptomatic thistle leaves into a dry powder. Thistle was allowed to senesce naturally in each field, which were left no-till to avoid disturbance of patch development.
In the spring of 2021, 90-kg/hectare of forage barley was drill seeded into each no-till field with an in-furrow application of 13-36-0 fertilizer at a rate of 112-kg/hectare. Thistle was allowed to freely grow through the summer within the barley crop. Data collection occurred at the ripening stage of the barley kernels. An Emlid Reach RS2 GNSS receiver was used to map the spread of thistle patch boundaries, and collect total patch area data for each treatment. Sampling of three additional transects was conducted, west and east from the original planting locations, to account for the spread of thistle beyond the original planting locations. Each transect was 20-m long, with ten 1-m2 samples taken at every 0.5-m interval (Figure 2). Data collection followed the same protocols that were established in 2020. Each field was swathed with a gas-powered trimmer, hand raked, and cleared of above-ground biomass following data collection. The fields were left untilled, and thistle rhizomes were allowed to re-establish through the fall. Once fall climate conditions reached an optimal range, a maximum of five thistle rosettes were inoculated within all 1-m2 sample frames along the four transects in the inoculated treatment. Inoculations were only applied within sample frames containing live thistle rosettes, following protocols established in 2020. In the spring of 2022, spring wheat was drill seeded in the fields at a rate of 95-kg/hectare. The sampling protocols that were established in 2021 were repeated at the harvest-ready stage of wheat in 2022, followed by removal of all aboveground biomass. The fields were left untilled through the fall and winter. In the spring of 2023, alfalfa was drill seeded in the fields of 17-kg/hectare. All data was collected, using previously established methods, at the Canada thistle flower bud development stage. The fields were flail mowed, with the mower set at a 12-inch height, to remove all Canada thistle biomass while conserving alfalfa stand establishment.
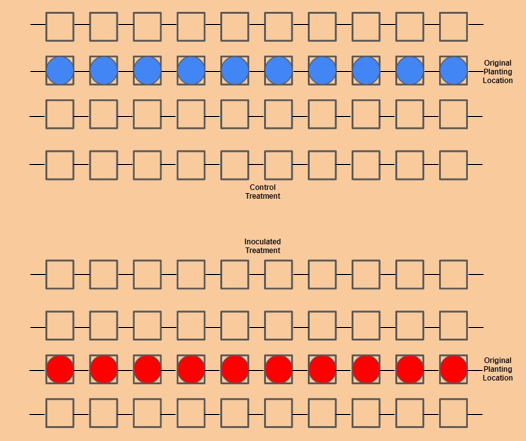
Works Cited
- Berner, D., Smallwood, E., Cavin, C., Lagopodi, A., Kashefi, J., Kolomiets, T., et al. (2013). Successful establishment of epiphytotics of Puccinia punctiformis for biological control of Cirsium arvense. Biological Control 67, 350–360. doi: 1016/j.biocontrol.2013.09.010.
- Gurevitch, J., Scheiner, S., and Fox, G. (2016). The Ecology of Plants. 2nd ed. Sinauer Associates, Inc.
- Hoffman, W. A., and Poorter, H. (2002). Avoiding Bias in Calculations of Relative Growth Rate. Annals of Botany 90, 37–42. doi: 1093/aob/mcf140.
- Kuznetsova, A., Brockhoff, P. B., and Christensen, R. H. B. (2017). lmerTest Package: Tests in Linear Mixed Effects Models. Stat. Soft. 82. doi: 10.18637/jss.v082.i13.
- Larson, C. D., Menalled, F. D., Lehnhoff, E. A., and Seipel, T. (2021). Plant community responses to integrating livestock into a reduced-till organic cropping system. Ecosphere 12, e03412. doi: 1002/ecs2.3412.
- Lehnhoff, E., Miller, Z., Miller, P., Johnson, S., Scott, T., Hatfield, P., et al. (2017). Organic Agriculture and the Quest for the Holy Grail in Water-Limited Ecosystems: Managing Weeds and Reducing Tillage Intensity. Agriculture doi: 10.3390/agriculture7040033.
- PRISM Climate Group, Oregon State University, https://prism.oregonstate.edu, data created 2014, accessed [ 04/22/2023].
- Ramsey, F., and Schafer, D. (2012). The Statistical Sleuth: A Course in Methods of Data Analysis. 3rd ed. Richard Stratton Available at: https://www.tandfonline.com/doi/full/10.1080/00224065.1998.11979882 [Accessed February 1, 2023].
- Soil Survey Staff, Natural Resources Conservation Service, United States Department of Agriculture. Web Soil Survey. Available online. Accessed [10/03/23].
- Weigelt, A., and Jolliffe, P. (2003). Indices of Plant Competition. Journal of Ecology 91, 707–720.
Research Objective 1: Assess how thistle rust responds to conventional and reduced tillage practices.
Cooperator Farm
The mean Canada thistle density per 1-m2 was 38.9 thistle stems in the crop field, and 34.8 thistle stems in the pasture. There is no evidence for a difference in mean thistle stem density per 1-m2 between habitat types at the Norgaard Farm in 2022 (generalized linear model, gaussian distribution, p-value= 0.770). The mean density of Canada thistle stems with rust symptoms per 1-m2 was 0.6 thistle stems with rust symptoms in the crop field and 1.5 thistle stems with rust symptoms in the pasture. There was no evidence for a significant difference in the mean density of Canada thistle stems with rust symptoms per 1-m2 between habitat types (generalized linear model, gaussian distribution, p-value= 0.134). There is strong evidence for a difference in mean patch area (m2) between the habitats at the Norgaard Farm in 2022 (generalized linear model, gaussian distribution, p-value<0.0001). The average patch area in the herbicide treated crop field was 3989.9- m2, and 331.0- m2 in the grazed pasture (Figure 3).
Habitat Type |
Management Type |
Average Thistle Patch Area (m2) |
Average Thistle Stem Density (1-m2) |
Average Thistle Stems with Rust Symptoms (1-m2) |
Average Thistle Stems with Rust Symptoms (Patch) |
Crop Field |
Herbicide |
3989.8 |
38.9 |
0.6 |
61.8 |
Pasture |
Grazing |
331.0 |
34.8 |
1.5 |
16.6 |
Mean Canada thistle stem densities (generalized linear model, p-value= 0.406) and stem densities with rust symptoms (generalized linear model, p-value= 0.12) were not impacted by the habitat type or management type at the Norgaard Farm. Mr. Norgaard's Fall 2021 application of glyphosate did not appear to have any significant impact on the overall thistle performance indicating that these thistle patches were resilient to a singular application of herbicide, likely attributed to well established below-ground energy stores. The differences in patch area between habitats may be attributed to past tillage events. Tillage within the crop field can change the soil profile by reducing compaction, leading to less obstruction to thistle rhizome spread. The pasture area consisted of perennial grasses and forbs that are rotationally grazed by 4 heads of cattle. The combination of vegetative competition, soil stability and minimized grazing has appeared to prevent large spatial spread of thistle within the pasture habitat. Thistle rust symptoms were generally consolidated to dense infection areas within patches, but showed no overall difference in densities per 1-m2 between habitat or management types. The observed grouping of symptoms within patches could mean that the pathogen is not effectively spread by grazers or by a combined legacy of tillage and herbicide application.
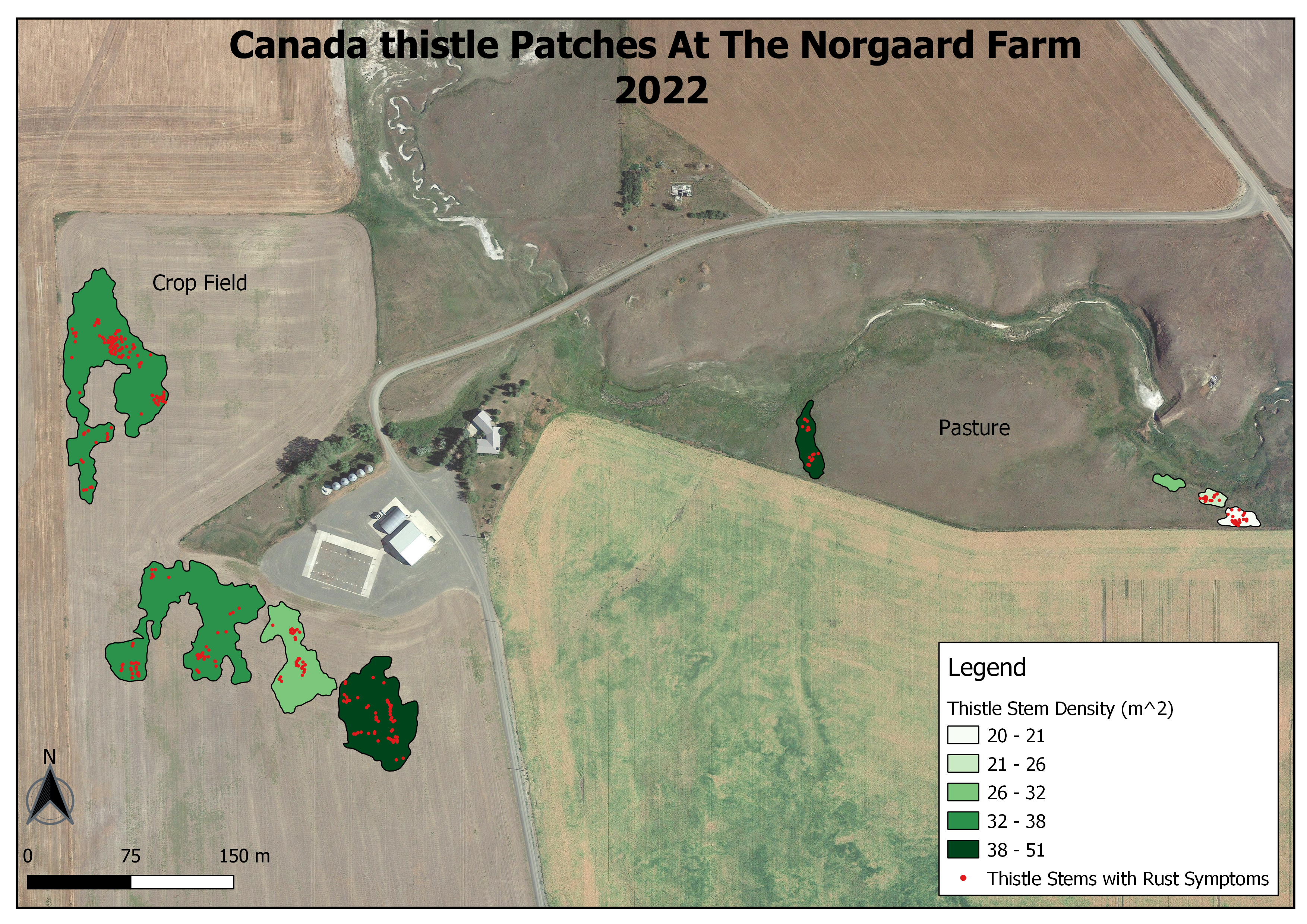
Montana State University (MSU) Fort Ellis Research Farm
Thistle rust infected stem density and growth rate
There was no evidence for a difference in thistle rust infected stem density (stems/patch) between tillage treatments alone (ANOVA χ2 =2.1616, p = 0.1415). However, infected stem density was different between study years (ANOVA χ2 =180.9016, p < 0.001), with an interaction between tillage treatments and years (ANOVA χ2 =127.8345, p < 0.001). During 2020 and 2021, there was no difference in infected stem density between tillage treatments (2020: z-ratio = 0.425, p = .6711; 2021: -0.173, p = 0.8624). There was a difference in infected stem density between tillage treatments in 2022 and 2023, where infected stem density was highest in the reduced tillage treatment during both years (2022: z-ratio = -2.123, p = 0.0330; 2023: -3.226, p = 0.0013). By the final year of the study (2023), the estimated mean infected stem densities were (±SE) 2.56 ± 1.06 stems in the standard tillage treatment and (±SE) 15.12 ± 5.49 in the reduced tillage treatment (Figure 4).
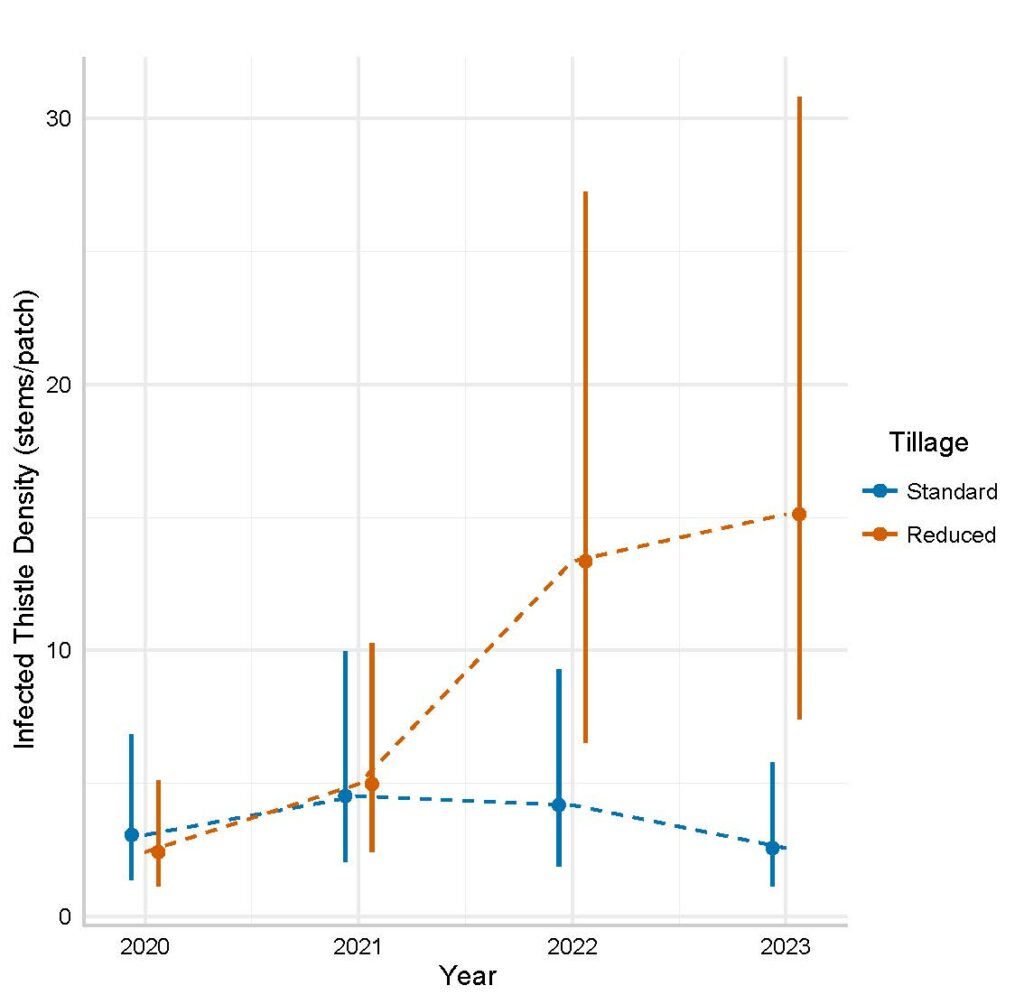
There was little-to-no evidence that the mean relative growth rate of thistle rust infected stems (stems/patch) was different between the reduced and standard tillage treatments (ANOVA F =2.4334, p = 0.1319). The estimated mean relative growth rates were (±SE) 0.261 ± .253 thistle rust infected stems per year in the standard tillage treatment and (±SE) 0.828 ± 0.234 thistle rust infected stems per year in the reduced tillage treatment (Figure 5).
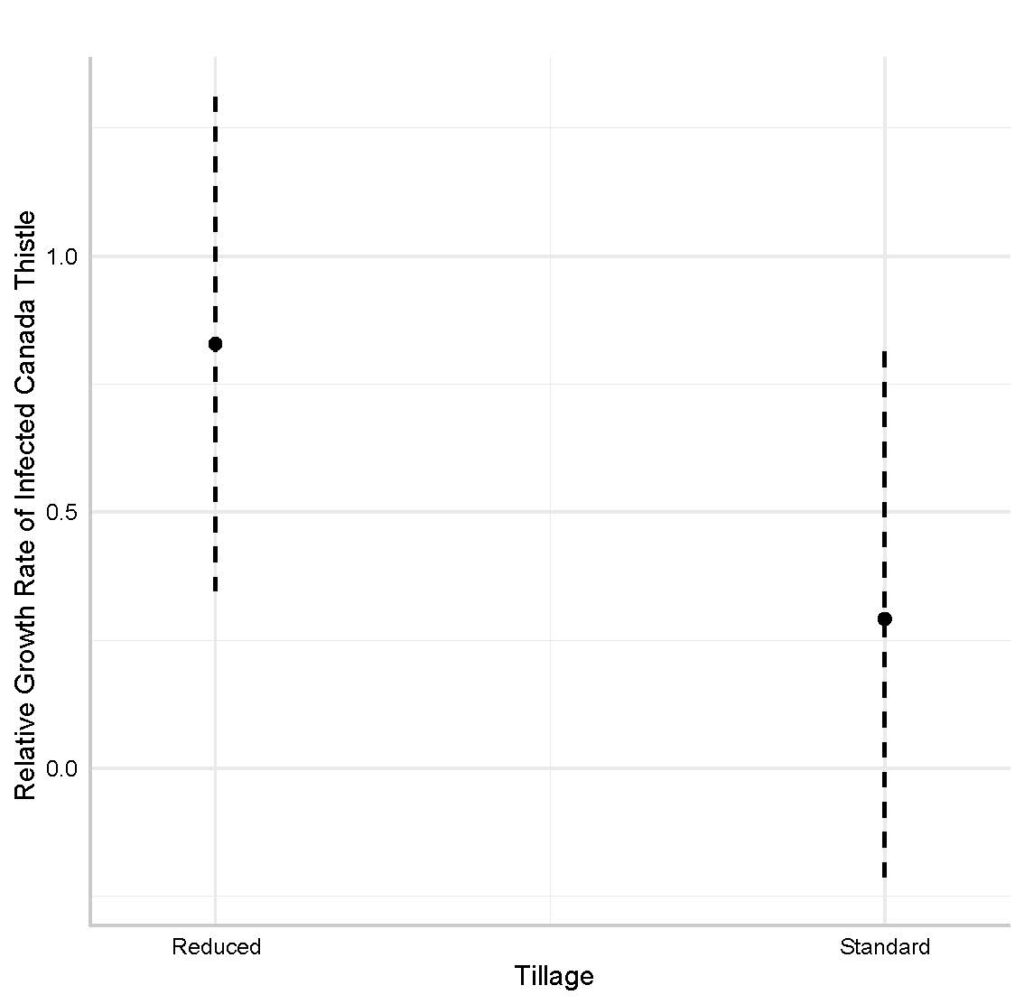
There was evidence that asymptomatic Canada thistle stem density (stems/m2) was lowest in the reduced tillage treatment (ANOVA χ2 =3.1580, p = 0.0756). Over all four years, the estimated mean thistle stem density per m2 was (±SE) 53.0 ± 3.9 in the reduced tillage treatment and (±SE) 64.6 ± 5.2 in the standard tillage treatment. There was an interaction between tillage treatments and years (ANOVA χ2 =10.9162, p = 0.0122). At the onset of the study, asymptomatic thistle density was not different between tillage treatments (z-ration = 0.222 , p-value = 0.8241). By 2023, asymptomatic thistle density had decreased in the reduced tillage treatment (z-ratio = 4.307, p-value = 0.0004) and did not change over time in the standard tillage treatment (z-ratio = -0.386, p-value = 0.999 , Figure 6).
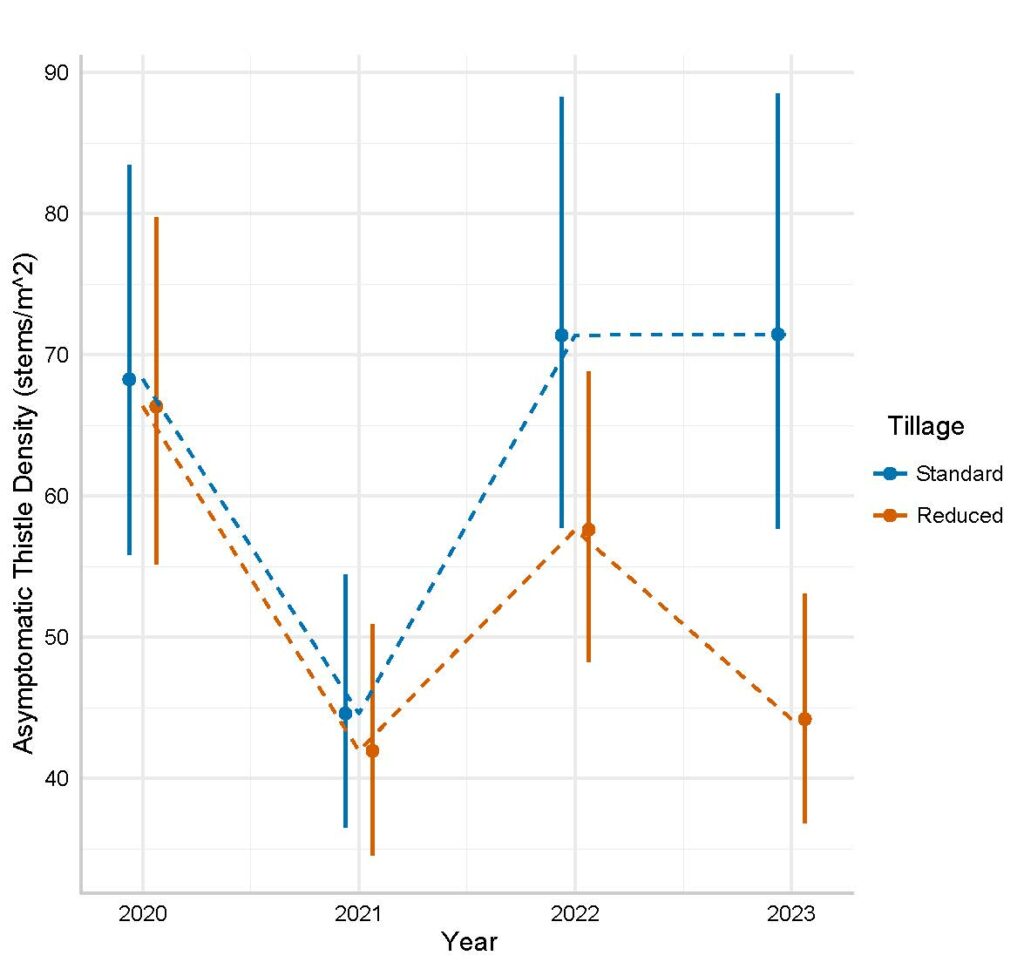
Research Objective 2: Compare the impacts of cropping management between rust infected Canada thistle and non-infected Canada thistle.
Montana State University Greenhouse
Thistle rust establishment
The overall percentage of thistle rust inoculated pots with systemically infected Canada thistle stems over the three trials was 52% with no infection observed in the non-inoculated control treatment. Systemically infected Canada thistle stems were observed in 15% of pots in the fallow phase, 65% of pots in the wheat phase, 60% of pots in the pea phase, and 67% of pots in the safflower phase (F = 14.159; p <0.001; Figure 7). The percentage of thistle rust infected stems in the inoculated treatment, out of all Canada thistle stems produced per pot, increased as the crop sequence progressed, with the largest increase occurring after the fallow phase (F = 8.58; p <0.001). The overall mean percentage of thistle rust infected stems per pot was 12%. Out of all stems produced per pot, 4% were systemically infected in the fallow phase, 14% were systemically infected in the wheat phase, 16% were systemically infected in the pea phase, and 14% were systemically infected in the safflower phase (Figure 8).
Canada thistle above- and belowground biomass
Canada thistle that was inoculated with thistle rust had 1.6 grams/pot (± 0.52) less aboveground biomass compared to non-inoculated (control) Canada thistle (F = 9.965; p = 0.0020). Canada thistle grown with crop competition produced (±SE) 3.1 ± 0.52 grams/pot less aboveground biomass than Canada thistle grown in monoculture (F = 36.396; p < 0.0001). Canada thistle biomass in the integrated thistle rust inoculated and crop competition treatment was (±SE) 4.8 ± 0.74 grams/pot less than Canada thistle biomass in the monoculture, non-inoculated treatment (t = 6.506; p < 0.0001; Figure 9).
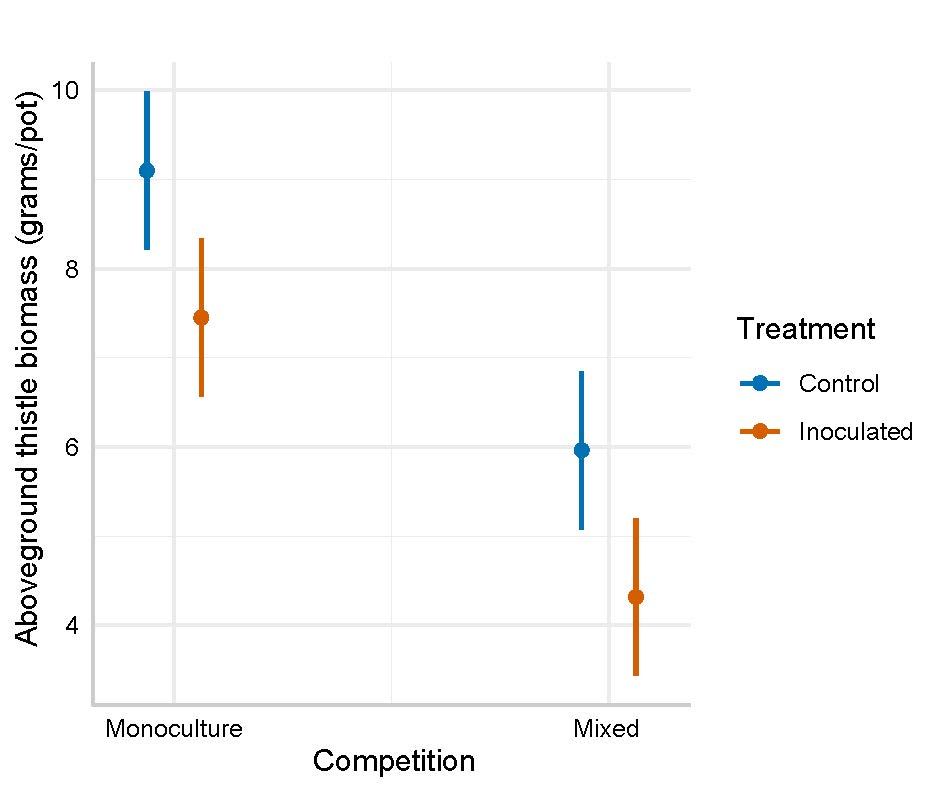
After starting each trial at the same weight, Canada thistle rhizome biomass was 6.9 grams/pot in the thistle rust inoculated treatment and 12.5 grams/pot in the non-inoculated (control) treatment, after an average of 12.9 months of growth. Rhizome biomass in the thistle rust inoculated treatment was less than rhizome biomass in the non-inoculated control treatment (F = 25.791; p < 0.0001). The estimated biomass of Canada thistle rhizome in the inoculated treatment was (±SE) 5.6 ± 1.1 grams/pot less than in the control treatment. Canada thistle grown with crop competition produced (±SE) 2.7 ± 1.1 grams/pot less rhizome biomass than Canada thistle grown in monoculture (F = 6.211; p-value = 0.0141). Rhizome biomass in the integrated thistle rust inoculated and crop competition treatment was (±SE) 8.3 ± 1.6 grams/pot less than rhizome biomass in the monoculture, non-inoculated (control) treatment (t = 5.353; p < 0.0001; Figure 10).
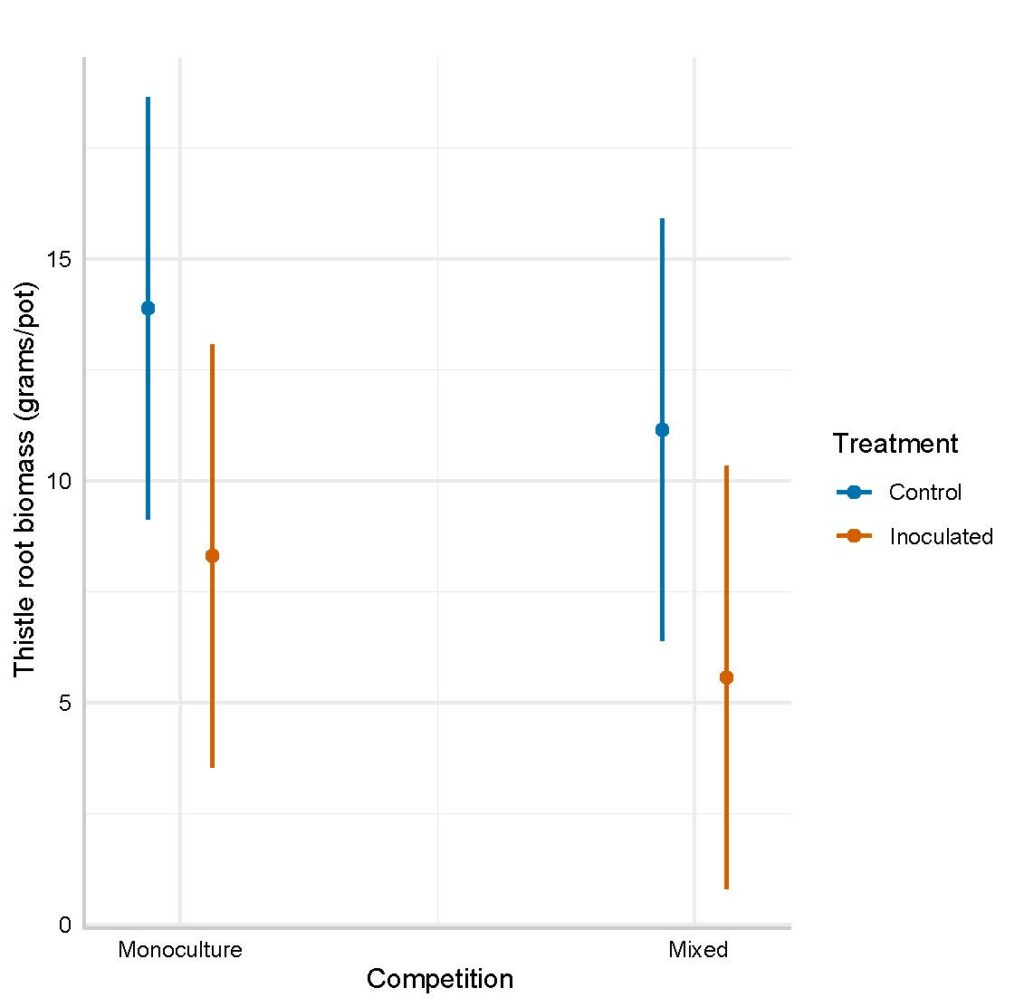
Crop competition reduced aboveground biomass, with (±SE) 49.2% ± 5.9 biomass loss in the inoculated treatment, and (±SE) 39.2% ± 5.9 biomass loss in the non-inoculated (control) treatment, when compared against the monoculture index for growth in the non-inoculated (control) treatment. There was little evidence for a difference in RCI between the inoculated treatment and the non-inoculated (control) (F = 2.816, p-value = 0.0987). The relative competition of Canada thistle varied between crop phases (wheat, pea, and safflower) in both the inoculated and control treatments (F = 63.669; p < 0.001). Crop competition reduced aboveground biomass by (±SE) 48% ± 5.9 in the wheat phase, (±SE) 71% ± 5.9 in the pea phase, and (±SE) 14% ± 5.9 in the safflower phase, when compared against the monoculture index for growth in the non-inoculated (control) treatment. Additionally, there was an interaction between the inoculation treatments and crop phases (F = 3.329; p = 0.0393). The RCI between the inoculation treatments increasingly separated as the crop sequence progressed, where the inoculated treatment lost (±SE) 24% ± 8.3 more biomass than the non-inoculated (control) treatment by the final safflower phase in the crop sequence (Figure 11).
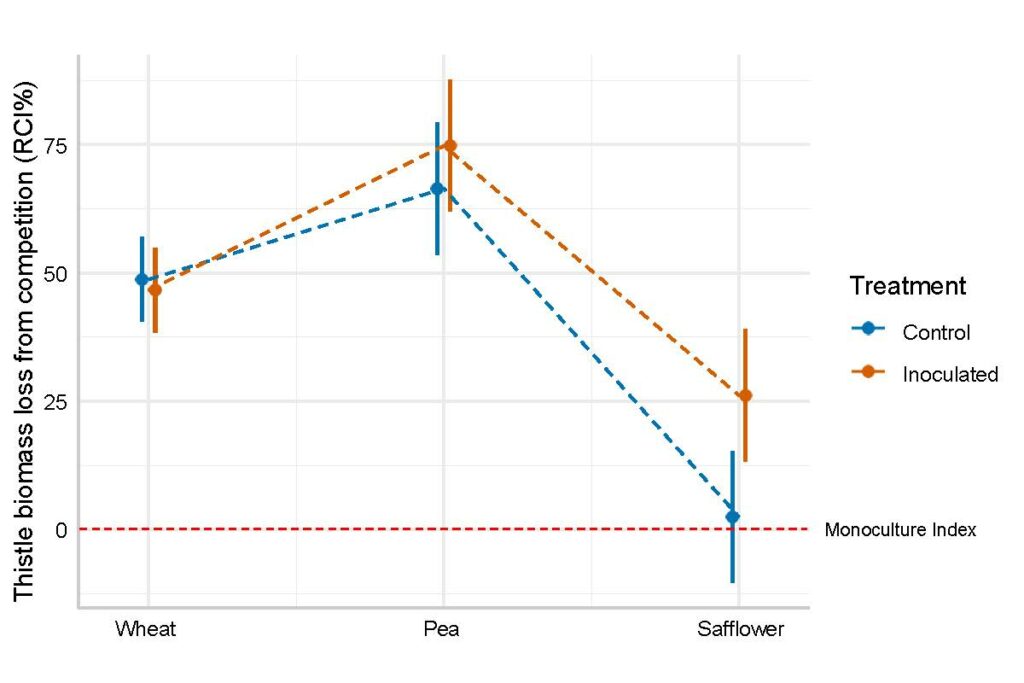
Montana State University Post Agronomy Farm:
There is very strong evidence that thistle density in the inoculated treatment was less than thistle density in the non-inoculated (control) (ANOVA χ2 =25.752, p < 0.001), with an interaction between treatments and study years (ANOVA χ2 =25.898, p < 0.001). There was initially no difference in thistle density between the treatments in 2020 and 2021 (2020: p = 0.7678; 2021: p = 0.8360). By 2022, the difference in thistle density between the treatments began to increase (p = 0.228), where the estimated mean thistle stem densities (stems/m^2) were (±SE) 12.2 ± 3.6 stems in the non-inoculated (control) treatment and (±SE) 8.91 ± 3.6 stems in the inoculated treatment. The trend continued in 2023 (p < 0.001), where the estimated mean thistle densities were (±SE) 28.52 ± 3.6 stems in the non-inoculated (control) treatment and (±SE) 17.22 ± 53.6 stems in the inoculated treatment (Figure 12).
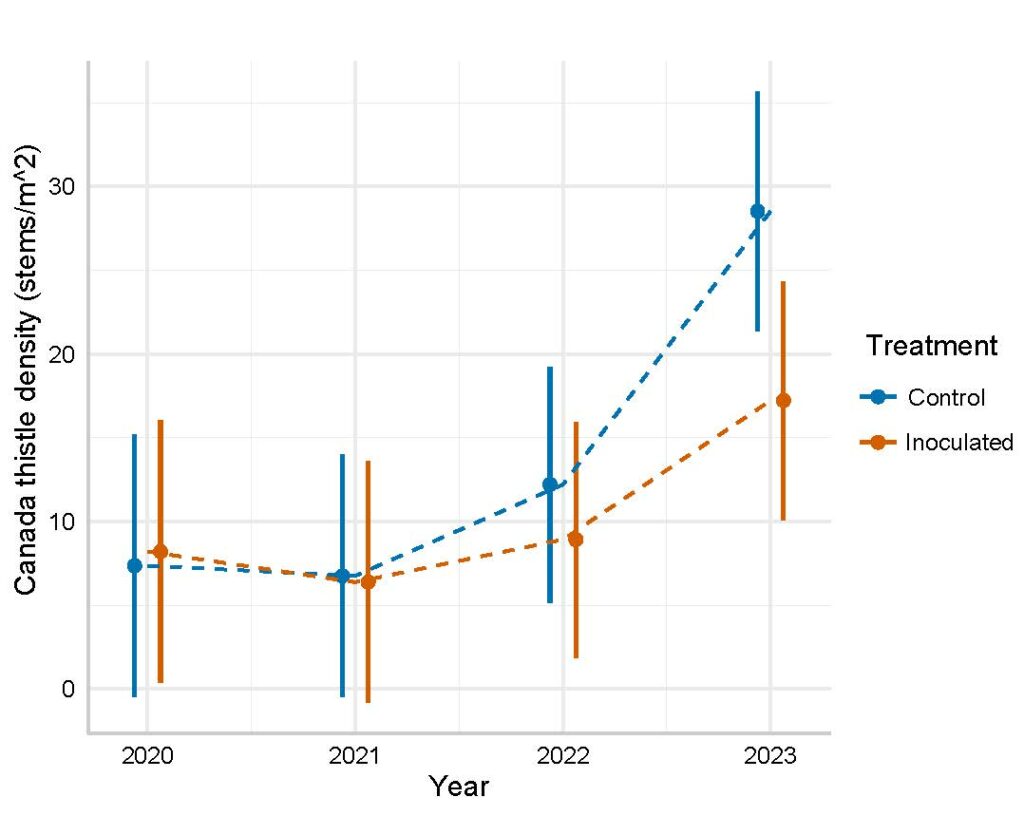
There is strong evidence that Canada thistle patch area was lowest in the thistle rust inoculated treatment compared to the non-inoculated (control) treatment (ANOVA F =82.977, p < 0.001; Figure 14), with an interaction between treatments and study years (ANOVA F =17.962, p < 0.001). Patch area was not different in 2021 (p = 0.2320), after one year of thistle spread. By 2022, the difference in patch area began to increase between the treatments (p<0.001), where the estimated mean patch areas were 179.7 - m2 in the non-inoculated (control) treatment and 151.8- m2 in the thistle rust inoculated treatment. Patch areas between the treatments separated further in 2023 (p < 0.001), with an estimated mean patch area of 323.3 - m2 in the non-inoculated (control) treatment and 251.1 - m2 in the thistle rust inoculated treatment (Figure 13).
Research Outcomes
Our findings suggest that thistle rust can effectively impact Canada thistle when integrated with cultural management, but may be most effective in reduced tillage systems where disturbance is minimal. There is potential to build upon this research by exploring the interaction of thistle rust with competitive crop sequences that include multiple years of a perennial forage. The use of perennial forages for Canada thistle management is advantageous because they can produce competitive root systems and dense canopies, they can aide soil fertility, and can be harvested multiple times per year with minimal soil disturbance, resulting in a reduction of Canada thistle carbon stores and a suppression of seed production when mowed (Hodgson, 1958; Carr et al., 2012; Jarvis et al., 2017; Favreliere et al., 2020). Furthermore, thistle rust may be successful in a multi-year perennial forage system, as the multi-year forage would facilitate slow development of the pathogen, the low disturbance regime would promote spore accumulation on the soil surface, and mechanical spore dispersal from mowing could increase the distribution range of transmissible spores.
Although our results show promising potential in favor of integrating thistle rust as a Canada thistle biocontrol in organic crop systems, there are many research steps that must be taken prior to a widespread adoption of the pathogen. For example, the obligate biology of thistle rust limits the ability to artificially culture transmissible spores (Kentjen et al., 2023), making it difficult to utilize the biocontrol agent in a scalable manner. Research efforts are constrained by the availability of thistle rust inoculum, which is currently sourced from wild populations. There is a need for new development of thistle rust production techniques that yield a reliable supply of inoculum with low economic costs. One possible way to address the shortage of inoculum is to intentionally cultivate Canada thistle in a controlled environment, where thistle rust spores could be produced for harvest and processing. Another option would involve a development of culture mediums that encourage the fungal life-cycle by simulating specific pathogen-host interactions that drive teliospore development.
To improve the efficacy of thistle rust as a biocontrol agent, there is a need for future research that explores pathogen-host interactions and the genetic factors that determine pathogen virulence. Puccinia pathogens are known to be genetically diverse (Kolmer et al., 2012). Molecular techniques have been used to identify virulence factors in species that impact crops (Wan and Chen, 2011), and more recently, identify genetic characteristics and diversity between thistle rust populations (Berner et al., 2015; Henderson et al., 2018). There is a need to build upon previous work with a concerted effort to identify and isolate virulent strains of thistle rust that are fit for field applications. If virulent mechanisms and thistle rust strains can be identified, then there is potential to incorporate breeding or engineering techniques that produce offspring with desirable characteristics, ultimately improving its efficacy in applied settings.
If thistle rust is to become a publicly available biocontrol product in the United States, it will be necessary to meet the requirements of the USDA Animal and Plant Health Inspection Service (APHIS). These requirements are meant to regulate the importation, interstate movement, and environmental release of plant pests or noxious weeds, and provide permitting for release of non-indigenous weed biocontrol agents. Additionally, the biocontrol would need to meet individual state requirements for labeling and use as an organically certified product. Completion of the federal regulatory processes would expand access to the biocontrol for future research and application opportunities, effectively increasing thistle rust's potential as a Canada thistle management tool.
Works Cited
- Berner, D. K., Smallwood, E. L., Cavin, C. A., McMahon, M. B., Thomas, K. M., Luster, D. G., et al. (2015). Asymptomatic systemic disease of Canada thistle (Cirsium arvense) caused by Puccinia punctiformis and changes in shoot density following inoculation. Biological Control 86, 28–35. doi: 1016/j.biocontrol.2015.02.006.
- Carr, P. M., Anderson, R. L., Lawley, Y. E., Miller, P. R., and Zwinger, S. F. (2012). Organic zero-till in the northern US Great Plains Region: Opportunities and obstacles. Renewable Agriculture and Food Systems 27, 12–20.
- Favrelière, E., Ronceux, A., Pernel, J., and Meynard, J.-M. (2020). Nonchemical control of a perennial weed, Cirsium arvense, in arable cropping systems. A review. Agronomy for Sustainable Development 40, 31. doi: 1007/s13593-020-00635-2.
- Henderson, C., Cripps, M., and Casonato, S. (2019). Distribution of Puccinia punctiformis in above-ground tissue of Cirsium arvense (Californian thistle). New Zealand Plant Protection Society 72, 265–270.
- Hodgson, J. M. (1958). Canada thistle (Cirsium arvense Scop.) control with cultivation, cropping, and chemical sprays. Weeds 6, 1–11.
- Jarvis, N., Forkman, J., Koestel, J., Kätterer, T., Larsbo, M., and Taylor, A. (2017). Long-term effects of grass-clover leys on the structure of a silt loam soil in a cold climate. Agriculture, Ecosystems & Environment 247, 319–328.
- Kentjens, W., Casonato, S., and Kaiser, C. (n.d.). Californian thistle (Cirsium arvense): endophytes and Puccinia punctiformis. Pest Management Science n/a. doi: https://doi.org/10.1002/ps.7387.
- Kolmer, J. A., Hanzalova, A., Goyeau, H., Bayles, R., and Morgounov, A. (2013). Genetic differentiation of the wheat leaf rust fungus Puccinia triticina in Europe. Plant Pathology 62, 21–31. doi: 1111/j.1365-3059.2012.02626.x
- Wan, A., and Chen, X. (2012). Virulence, Frequency, and Distribution of Races of Puccinia striiformis f. sp. tritici and P. striiformis f. sp. hordei Identified in the United States in 2008 and 2009. Plant Disease 96, 67–74. doi: 1094/PDIS-02-11-0119.