Progress report for GW21-228
Project Information
Nitrogen is a critical nutrient for fertilizing our crops that can be acquired non-synthetically via symbiotic nitrogen fixing bacteria associating with legumes. Legumes are widely used in crop rotations, and there is growing interest in using them in intercropping systems. Effective nitrogen fixation and nitrogen cycling depends on the microbial community, so it is important to understand how our agricultural practices impact these microbial communities. This project will determine how a mixed cropping system of pea and canola impacts microbial communities in the soil, rhizosphere, and roots in this system. Analysis of the diversity of the microbial communities will be done to determine the abundance of nitrogen fixing bacteria, how well they are performing, and how they are contributing to the growth and nitrogen content of pea and canola crops. Results from this study will be available to farmers and stakeholders via talks and posters at producer-focused workshops, conferences, field days, and more. The expected outcomes from this project are (1) to have a clearer understanding on how the way we grow our crops is impacting microbial communities, (2) to have suggestions for the farming community in Eastern Washington to improve the performance and abundance of nitrogen fixing bacteria in order for them to produce their crops more sustainably and efficiently, and (3) to help guide further research on nitrogen fixing bacteria as a means for nitrogen fertilization and to obtain a more sustainable and productive method of farming, while improving and maintaining soil health.
Our research objectives are:
- To determine if the peaola intercropping system that we are studying is producing similar results for yield and land equivalent ratio (LER) as other studies have found.
- To determine the biodiversity and abundance of the nitrogen fixing bacteria of the microbial populations present in soil, rhizosphere, and root samples collected from pea and canola in monoculture and intercropped, and with varying levels of nitrogen fertilizer application.
- To determine if there is a difference in the community structure and importance of nitrogen fixing bacteria in the microbial communities present in the different cropping systems, including the symbiotic ability of the pea-associated nitrogen-fixing bacteria.
- To determine the nitrogen content of the crops grown in monoculture and in the peaola intercropping system to determine whether symbiotic nitrogen fixation by pea can meet the nitrogen needs of intercropped canola.
Our primary education/outreach objective is:
To improve sustainability and productivity of the agricultural system across the inland Pacific Northwest by quantifying crop yield, changes to the soil and plant microbiome, and economic benefit when peaola is adopted as a rotational crop in a dryland wheat-based system.
Our education/outreach sub objectives are:
- To guide agricultural practices in the inland Pacific Northwest through sharing the results of this study with producers and agricultural stakeholders.
- To increase the awareness of the general public in the inland Pacific Northwest about the benefits of intercropping and peaola.
- To guide future research on intercropping and peaola in the inland Pacific Northwest.
For us to accomplish the various experiments and events we describe in this proposal, we will follow the below timeline. It should be noted that some of the steps for this project will happen and have happened before the start of this grant’s funding.
WSARE Grant Proposal Application: February 9th
First sample collection at Mark Greene’s farm: April-May 2021
First crop yield analysis: April-May 2021
First sample nitrogen tests: April-May 2021
First DNA extractions: May-June 2021
Initial sequencing and qPCR: June-July 2021
Data analysis of first samples: July-December 2021 (Could need an even longer of period to perform the data analysis due to the presented difficulty of data normalization)
Preparation of outreach materials based on first set of results: December 2021-April 2022
Second planting and growth of strip plots at Mark Greene's farm: September 2021-April 2022
Potential submission of an initial manuscript: December 2021-April 2022
Second sample collection at Mark Greene’s farm: April-May 2022
Second crop yield analysis: April-May 2022
Second nitrogen tests: April-May 2022
Second DNA extractions: May-June 2022
Second qPCR: June 2022-July 2022
Data analysis of the second samples: July-December 2022 (Same conditions as first round)
Tri-societies meeting: November 6th-9th, 2022
Regional soil health meeting: Winter 2022
Analysis of combined data: December 2022-January 2023 (May happen earlier depending on ease of data analysis)
Prepare final outreach materials: January 2023
Work on submitting more comprehensive manuscript: February 2023-August 2023
Attended and contribute to outreach events and platforms discussed in our education plan: February 2023-August 2023
With No-Cost Extension
Aug-Nov 2023: DNA extraction and 16S sequencing of roots + nifH SmartChip runs
Nov-Dec 2023: Tri-societies meeting and data analysis
Dec 2023-Jan 2024: Prepare final outreach materials
Winter 2024: Regional soil health meeting
Jan-August 2024: Manuscript preparation and submission + outreach activities
Cooperators
- (Researcher)
- - Producer
- (Researcher)
- (Researcher)
Research
The objectives of our project are:
- To determine if the peaola intercropping system that we are studying is producing similar results for yield and land equivalent ratio (LER) as other studies have found.
- To determine the biodiversity and abundance of the nitrogen fixing bacteria of the microbial populations present in soil, rhizosphere, and root samples collected from pea and canola in monoculture and intercropped, and with varying levels of nitrogen fertilizer application.
- To determine if there is a difference in the community structure and importance of nitrogen fixing bacteria in the microbial communities present in the different cropping systems, including the symbiotic ability of the pea-associated nitrogen-fixing bacteria.
- To determine the nitrogen content of the crops grown in monoculture and in the peaola intercropping system to determine whether symbiotic nitrogen fixation by pea can meet the nitrogen needs of intercropped canola.
Land Equivalent Ratio and Yield Analysis
For our first objective, peaola plot trials were planted across three different years (2020, 2021, and 2022). Peaola planted in 2020 was located at a farm near Colfax, WA where the plots were 11 m by 61 m. These plots were planted and sampled prior to the project start date, but are being included due to their relevance to the overall project and final datasets. In 2021 and 2022 peaola was planted at a WSU research farm near Davenport, WA where the plots were 2 m by 8 m and at Mark Greene's farm where the plots were 12 m by 152 m. At the WSU research farm, the 2021 and 2022 plots were seeded into no-till winter wheat chemical fallow using a Fabro double disk no-till drill. The winter pea variety Goldenwood (ProGene Plant Research, LLC) and the canola variety Plurax (Rubisco Seeds) were used in both the monoculture and intercrop as both varieties have been successfully grown in the Palouse. In the intercrop, peas and canola were planted in the same row at the same time. Planting occurred in late August as a compromise between the pea and canola ideal planting periods. The whole plot yield was sampled, using a M-2B clipper mill from A. T. Ferrell & Company Bluffton, Indiana which could separate the peas and canola. The plots near Colfax, WA were planted on April 9th, 2020, into stubble from the previous year's winter wheat crop using a no-till Cross Slot drill. Again, the winter pea variety used was Goldenwood (ProGene Plant Research, LLC) and a Clearfield spring-canola variety (DynaGro 200 CL) was used. Strips were harvested on September 14th, 2020 and weighed using a weigh wagon. A 1 kg subsample was collected from the grain stream which then had peas and canola separated using an M-2B clipper mill from A.T. Ferrell & Company Bluffton, Indiana. Peas and canola were weighed separately and used to calculate the relative pea and canola yield. At the Greene Farm in 2021 and 2022, 12 m by 152 m large strip trials were seeded into chemical fallow winter wheat stubble on April 20th, 2021, and May 17th, 2022. Banner variety spring pea purchased from ProGene Plant Research, LLC and canola NCC101s (Photosyntech) were used. A horsch hoe style winged opener was used to seed the plots, with the peas being sent down the dry fertilizer shoot in the mid-row and canola being placed an inch deep by the winged openers.
Land equivalence ratio (LER) was calculated using Equation 1, where ICp and ICc represent the yields of intercropped pea and canola, respectively, and Mp and Mc represent the yields of monoculture pea and canola, respectively. Mp and Mc were calculated through the mean of all four replicates for peas and canola, respectively. The LER for each individual peaola plot was calculated using the same Mp and Mc for the corresponding year.
Equation 1: LER = (ICp/Mp) + (ICc/MCc)
Microbial Community Analysis
To accomplish our second objective, we have collected samples from small strip plots (2 m x 8 m) at a WSU research farm near Davenport, WA and large strip trials on Mark Greene’s farm (12 m x 152 m) near Cloverland, WA and at farm located near Colfax, WA (11 m x 61 m). The large strip trials at the Greene farm consisted of 1 peaola plot in 2021 and 2 peaola plots in 2022 to accommodate for the needs of the producer. Large strip trials located near Colfax, WA consisted of 4 replicates of peaola (33 kg N ha-1), 4 replicates of pea (0 kg N ha-1), and 4 replicates of canola (67 kg N ha-1). The small plot trials near Davenport, WA in 2021 were comprised of peaola at three N rates (4 replicates at 0 kg N ha-1, 4 replicates at 33 kg N ha-1, and 8 replicates at 67 kg N ha-1), 4 replicates of pea (0 kg N ha-1), and 4 replicates of canola (67 kg N ha-1). In 2022 the small plot trials near Davenport, WA consisted of 36 replicates of peaola, 4 replicates of pea, and 4 replicates of canola. Seeding rates were varied amongst peaola (Table 1). N application rates were originally given as percentages in the proposal due to uncertainty in the needed application rate at the time the proposal was written. Therefore, the values 0%, 50%, and 100% N refer to 0, 33, and 67 kg N ha-1 respectively as the percentages represented steps in the application rate. The number of replicates varies from what was indicated in the proposal due to changes in the needs of the producer, Mark Greene, and the agronomist, Dr. Isaac Madsen. These changes were needed by Mark Greene due to limited availability of space for growing crops. Dr. Isaac Madsen needed to change the experimental design at the Davenport, WA location due to the results of prior experiments which showed a need to investigate the impacts of seeding rates in 2022. For each plot, we collected soil and root samples at 3 different points. Soil was stored at -20C. Rhizosphere was collected from all root samples into rhizosphere buffer and both samples were stored at -80C.
Table 1. Peaola seeding rates for the 2022 trials at the Davenport, WA location.
Peaola Seeding Treatment | Canola Seeding Rates (seeds/ha) | Pea Seeding Rates (kg/ha) |
1 | 247,000 | 89.41 |
2 | 247,000 | 111.76 |
3 | 247,000 | 134.12 |
4 | 494,000 | 89.41 |
5 | 494,000 | 111.76 |
6 | 494,000 | 134.12 |
7 | 741,000 | 89.41 |
8 | 741,000 | 111.76 |
9 | 741,000 | 134.12 |
10 | 0 | 111.76 |
11 | 494,000 | 0 |
12 | Null = Leftover seeds | Null = Leftover seeds |
DNA extractions were performed on the collected soil and rhizosphere samples using a Kingfisher DNA extraction machine following the Earth Microbiome Project’s protocol for the QIAGEN® MagAttract® PowerSoil® DNA KF Kit. 8 roots were freeze dried and crushed to a powder before performing DNA extracting using CTAB (a cationic detergent that can extract and purify DNA from bacteria) in order to pilot this method of sample handling. Extracted DNA was sent to Michigan State University’s Research Technology Support Facility for Illumina Amplicon Sequencing of the 16S V4 region on the MiSeq v2 Standard platform, resulting in 250 bp paired end reads. We will be performing qPCR for the nifH gene, which is a functional gene associated with nitrogen fixation and is often used as a marker for nitrogen fixing bacteria. This will be performed using the SmartChip Real-Time PCR System. Through doing qPCR of the nifH gene, we will be able to determine approximately how many bacteria in the population can perform nitrogen fixation.
Sequences were analyzed using QIIME2 version 2021.8 on WSU’s Kamiak High Performance Computing Cluster. Alpha-rarefaction plots showed that our sampling depth was sufficient for analysis of the microbial community. Bacteria were classified using “qiime feature-classifier classify-sklearn” with the Silva 138 99% OTUs from 515F/806R classifier found on the QIIME2 data resources page. After removing sequences representing chloroplasts and mitochondria, the bacterial core microbiome was found using “qiime feature-table core-features”. For nifH, we will be looking at the difference in the abundance of the nifH gene between the samples. This will allow us to determine if there are more nitrogen fixing bacteria present in a particular treatment. Analysis of this data will be done using R version 4.2.1 in RStudio version 2022.07.0+548.
Network Analysis
We performed network analysis on the microbial count data used in objective 2 from Illumina amplicon sequencing of 16S rDNA for our third objective using SPIEC-EASI version 1.1.0 in R version 4.1.0 on WSU’s Kamiak High Performance Computing Cluster. SPIEC-EASI was chosen due to it being acknowledged as an appropriate method to produce a co-occurrence network from microbial abundance data. We used Cytoscape version 3.9.1 to analyze the networks, where bacterial RSVs are represented by individual nodes. Networks were produced for peaola, pea, and canola individually to allow for comparisons of the network structure across the three cropping systems. We will also be generating networks using the above methods, but having each node represent the microbial community at the Phylum level.
Stable Isotope Analysis
To accomplish our fourth objective, we were able to determine how the microbial communities impact the levels of nitrogen present in the plant samples through performing stable isotope analysis, which relates to our fourth objective. To do this, plant shoots were collected at each sampling point across the different trials except for in 2021 due to a communication error. Shoot samples were dried, leaf tissue was collected, and crushed to a fine powder. Stable isotope analysis was then performed at the WSU Stable Isotope Core providing us with C and N percentages for each sample.
Results
Land Equivalent Ratio and Yield Analysis
For our first objective, we found that the cropping system had a significant impact on the yield of pea and canola, and on the LER. Across the trials grown at the WSU research farm in 2021 and the trials grown near Colfax, WA in 2020, it was found that the average LER was 1.63 for peaola compared to the normalized value of 1 for the monocultures. The application of N fertilizers had no significant impact on the yield of peas and canola, or the LER for both of these locations. For the strip trials grown in Colfax, WA, the LER for peaola, at 1.37, was not significantly different from the monocultures. It was found that the yield of canola was not significantly different from when it was grown in monoculture, but the yield of pea was significantly reduced compared to when it was grown in monoculture at the Colfax, WA location. At the WSU research farm there was a significant increase in the LER of peaola from monoculture. However, there was a significant reduction in pea and canola yields from monoculture. At the Greene farm in 2021, no yield data was collected due to poor performance of the peas, so it was considered a loss. LERs were not calculated for the crops planted in 2022 at the WSU research farm and at the Greene farm as crops at the WSU research farm location were damaged significantly by deer and yield data was not collected at the Greene farm due to Dr. Isaac Madsen's move to Africa. The LERs that were recorded do match the range that has been recorded for peaola in the literature at low N application rates (Dowling et al., 2021).
Microbial Community Analysis
To accomplish our second, third, and fourth objectives, we have collected 264 soil samples, 459 root and rhizosphere samples, and 333 plant shoots across 3 different locations from 2020 to 2022. Substantial delays were experienced in regard to accomplishing our second and third objectives due to there being initial issues with our DNA extraction and quality control pipeline. As a result, we are slightly behind schedule in regard to our sequencing timeline, but we are quickly getting back on schedule. To date, we have extracted DNA from all of the soil and rhizosphere samples we collected in 2020, 2021, and 2022. Extractions have begun on the root samples DNA with sequencing expected to happen by August. Sequencing has been done on all of the soil and rhizosphere samples collected in 2020, 2021, and 2022. Some of these samples are still actively being sequenced by the Michigan State University Genomics Core with results expected to be back by late August. Due to delays in our optimization of our lab's protocol of the SmartChip Real-Time PCR system, we have not performed qPCR for the nifH gene on all of the samples. Currently, work is being done to test a small batch of samples within the system. Once this test has been completed and is successful, all extracted samples will be put through the protocol. Network analysis has been successfully done on the sequences for our 2020 samples. Stable isotope analysis for C and N percentages has been performed on the leaf tissue of the shoot samples collected in 2020 and 2022. Analysis of these results has been completed for both years. All results can be found bellow.
Data analysis has been done on the 2020, 2021, and 2022 soil and rhizosphere sequences. Through the inclusion of a microbial community standard, we determined we were able to detect bacteria at the appropriate abundance down to a relative abundance of 0.089%. When analyzing the 2020 soil using Shannon diversity index, Observed Features, and Evenness (measures of α-diversity) we did not find any significant differences (P < 0.05) or trends (0.05 ≤ P ≤ 0.1) between pea monoculture, canola monoculture, and peaola. We did find a trend towards the monoculture pea soil being richer than the monoculture canola soil using Faith's Phylogenetic Diversity index (Kruskal–Wallis Test, n1 = 12, n2 = 12, H = 5.60, p = 0.0537) (Figure 1). No other significant differences or trends were found using Faith's Phylogenetic Diversity index. For the 2020 rhizosphere, we found that the bacterial community in pea is significantly more even than canola (P < 0.05) and that there was a trend towards it being more even than intercropped canola (0.05 ≤ P ≤ 0.1) (Figure 2).
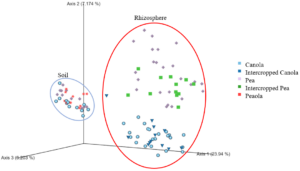
When looking at the β-diversity of the 2020 soil using Jaccard distance, Bray-Curtis distance, and unweighted UniFrac distance, we did not find any significant differences (P < 0.05) or trends (0.05 ≤ P ≤ 0.1) between pea monoculture, canola monoculture, and peaola. Under weighted UniFrac distance, we found that between pea and canola monoculture soils, and between peaola and canola monoculture soils, there was a trend toward there being a difference in the community composition (PERMANOVA, F = 2.06, P = 0.0705; PERMANOVA, F = 1.72, P = 0.0705) (Figure 3). This was not observed between pea monoculture and peaola soils. When looking at the 2020 rhizosphere, we found a significant difference in the β-diversity between canola and pea rhizosphere regardless of cropping system (PERMANOVA, P<0.05), and trends between like intercropped and monoculture crops (PERMANOVA, 0.05≤P≤0.1) based on Bray-Curtis distance (Figure 3). Similar observations were found using other measurements of β-diversity.
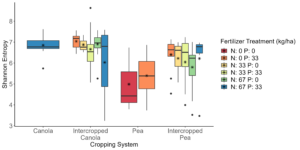
Analysis of the 2021 soil samples showed that there are no significant differences across all measure of α-diversity and β-diversity between the different cropping systems and treatment groups (Figure 5). For the 2021 rhizosphere samples, it was found that pea with a fertilizer treatment of 0 kg N ha-1 and 33 kg P ha-1 is significantly (P < 0.05) more diverse than intercropped canola with the same fertilizer treatment and a fertilizer treatment of 67 kg N ha-1 and 0 kg P ha-1 (Figure 4). Pea with a fertilizer treatment of 0 kg N ha-1 and 33 kg P ha-1 trended (0.05 ≤ P ≤ 0.1) towards being more diverse than canola with a fertilizer treatment of 67 kg N ha-1 and 33 kg P ha-1 (Figure 4). The same pattern was seen for intercropped pea with a fertilizer treatment of 67 kg N ha-1 and 0 kg P ha-1, but it was significantly (P < 0.05) more diverse than canola with a fertilizer treatment of 67 kg N ha-1 and 33 kg P ha-1 (Figure 4).
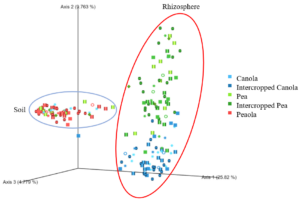
Using Bray-Curtis distance, it was found that there was a significant difference (P < 0.05) between most pea and canola samples regardless of treatment or cropping system (Figure 5). However, intercropped canola with a fertilizer treatment of 33 kg N ha-1 and 0 kg P ha-1 is not significantly different (P > 0.05) from pea with no fertilizer and intercropped pea with fertilizer treatments of 33 kg N ha-1 and 0 kg P ha-1 and 67 kg N ha-1 and 33 kg P ha-1 (Figure 5). There was also a trend (0.05 ≤ P ≤ 0.1) towards it being significantly different from intercropped pea with a fertilizer treatment of 33 kg N ha-1 and 33 kg P ha-1 and pea with a fertilizer treatment of 0 kg N ha-1 and 33 kg P ha-1 (Figure 5). Intercropped canola with a fertilizer treatment of 67 kg N ha-1 and 33 kg P ha-1 was also found to not be significantly (P > 0.05) different from pea with no fertilizer and intercropped pea with a fertilizer treatment of 67 kg N ha-1 and 33 kg P ha-1 (Figure 5). Some significant differences (P > 0.05) were also observed between like crops. These were seen between intercropped canola with a fertilizer treatment of 67 kg N ha-1 and 33 kg P ha-1 and canola with the same fertilizer treatment and intercropped canola with the fertilizer treatments of 0 kg N ha-1 and 33 kg P ha-1 and 67 kg N ha-1 and 0 kg P ha-1 (Figure 5). A trend (0.05 ≤ P ≤ 0.1) towards intercropped pea with a fertilizer treatment of 0 kg N ha-1 and 33 kg P ha-1 and 67 kg N ha-1 and 0 kg P ha-1 was found (Figure 5).
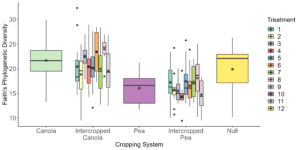
Similar to the results we saw in 2021, we saw no significant differences in the measures of α-diversity and β-diversity for the 2022 soil samples across the different cropping systems and treatments (Figure 7). When looking at the results for α-diversity in the 2022 rhizosphere samples there were significant differences (P < 0.05) and trends (0.05 ≤ P ≤ 0.1) across different cropping systems and seeding treatments using Faith's Phylogenetic Diversity index (Table 2 and Figure 6). Significant differences (P < 0.05) also existed between canola 2 and 8 and canola 8 and null Figure 6. Trends (0.05 ≤ P ≤ 0.1) existed between canola treatments 1 and 8, canola 2 and 3, canola 2 and 6, canola 4 and 8, canola 5 and 8, and pea 1 and 4 (Figure 6).
Table 2. Results for Faith's Phylogenetic Diversity index, with yes indicating a significant difference (P < 0.05) and trend indicating a trend (0.05 ≤ P ≤ 0.1)
Crop and Treatment | Pea 1 | Pea 2 | Pea 3 | Pea 4 | Pea 5 | Pea 6 | Pea 7 | Pea 8 | Pea 9 | Pea 10 |
Canola 1 | Yes | Yes | Yes | Trend | Yes | Trend | ||||
Canola 2 | Trend | Yes | Trend | |||||||
Canola 3 | Yes | Yes | Yes | Yes | Yes | Yes | Yes | Yes | Yes | Yes |
Canola 4 | Yes | Yes | Yes | Trend | Yes | |||||
Canola 5 | Trend | Yes | Yes | Yes | Trend | |||||
Canola 6 | Yes | Yes | Yes | Yes | Yes | Yes | Yes | Trend | Yes | Yes |
Canola 7 | Trend | Yes | Yes | |||||||
Canola 8 | Yes | Yes | Yes | Yes | Yes | Yes | Yes | Yes | Yes | Yes |
Canola 9 | Yes | Trend | ||||||||
Canola 11 | Trend | Yes | Yes | Yes | Trend | Yes | Trend | Yes | Yes | |
Null 12 | Trend | Yes | Yes | Trend | Yes | Trend |
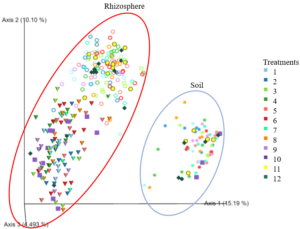
The results for β-diversity for the 2022 rhizosphere samples using Bray-Curtis distance showed a similar pattern as was observed for the previous 2 years of data. Significant differences (P < 0.05) existed between pea and canola samples across treatments groups (Figure 7). Interesting, significant differences (P < 0.05) were observed between canola 6 and canola 1 and canola 6 and canola 4 (Figure 7). Trends (0.05 ≤ P ≤ 0.1) were observed between canola 6 and canola 9, canola 7 and null, canola 11 and canola 1, canola 1 and canola 3, canola 5 and canola 6, canola 3 and null, canola 1 and canola 4, canola 2 and canola 6, canola 4 and null, and pea 1 and 5 (Figure 7).
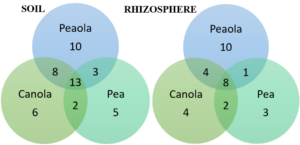
Analyses of the bacterial core microbiomes of our 2020 soil and rhizosphere samples were performed. Analysis of the 2021 and 2022 samples will be done. For soil, we analyzed the strict bacterial core microbiome, consisting of bacteria found in 100% of our samples. We found that the peaola core microbiome consisted of 34 members, the pea core microbiome consisted of 23 members, and the canola core microbiome consisted of 29 members. When comparing those microbiomes, we found that 13 bacteria were shared across them. From the canola core microbiome, 8 bacteria were shared with peaola. Out of the pea core microbiome, 3 bacteria were shared with peaola. Despite the peaola microbiome having shared members with monoculture pea and canola, it does have 10 core members that were unique to its own microbiome (Figure 8). When analyzing the rhizosphere, we did not analyze the strict core microbiome because it was not found across all 3 cropping systems. Instead, we analyzed core microbiomes consisting of bacteria found across 90% of our samples since a core microbiome was found across all 3 cropping systems at this level. At this level, we found that the peaola core microbiome consisted of 23 members, the pea core microbiome consisted of 14 members, and the canola core microbiome consisted of 18 members. When comparing those microbiomes, we found that 8 bacteria were shared across them. From the canola core microbiome, 4 were shared with peaola, and from the pea core microbiome, 1 was shared with peaola. Again, we found that despite peaola having shared core members with the microbiomes of pea and canola monoculture, we did find 10 unique members of the peaola core microbiome (Figure 8).
Network Analysis
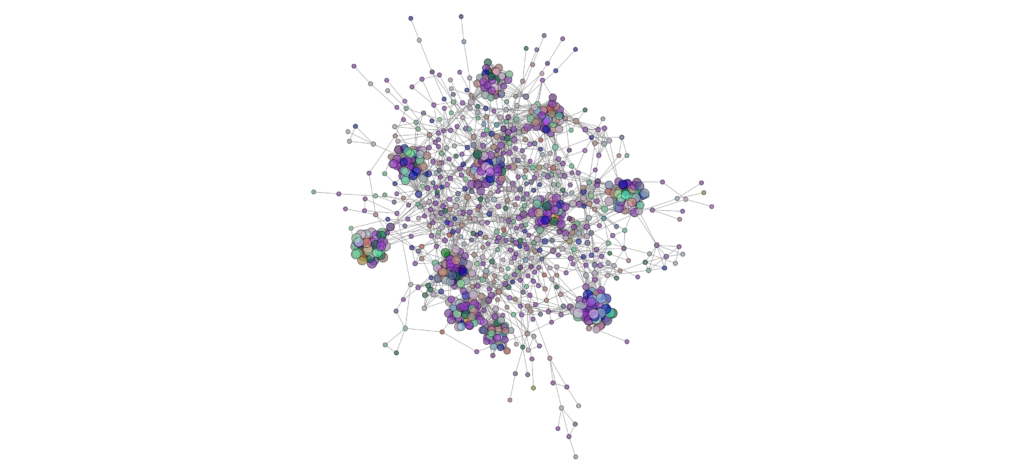
Network analysis using SPIEC-EASI has been performed successfully at the genus level on the 2020 soil samples. It should be noted, however, that identification to the genus level is rare when performing analyses on Illumina amplicon sequences due to the small size of the sequences. The peaola co-occurence network consists of 1016 nodes and 10,058 edges (Figure 9). The pea monoculture network consisted of 984 nodes and 8674 edges and the canola monoculture network consists of 965 nodes and 8330 edges (Figures 10 and 11).
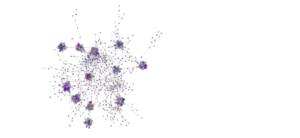
This shows that there is a change in the structure of the microbial community from monoculture pea and canola to peaola, which can also be seen visually (Figures 9, 10, and 11). Although there are nodes that are more highly connected than others, there does not appear to be any one node that is more highly connected than the others. Instead, there are clusters of highly connected nodes found throughout all 3 networks.
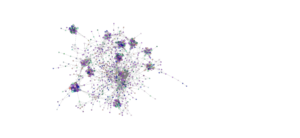
Stable Isotope Analysis
After performing statistical analyses on the nitrogen percentages for the 2020 samples collected at the Davenport location, we determined that there were no significant differences (P < 0.05) or trends (0.05 ≤ P ≤ 0.1) between the intercropped and monoculture pea and canola, and between pea and canola regardless of cropping system (Figure 12). For the 2022 samples, we found monoculture pea had significant more (P < 0.05) nitrogen in its leaf tissue than canola monoculture. Additionally, we found that pea in peaola treatments 1, 6, and 8 had significantly more (P < 0.05) nitrogen in their leaf tissue than monoculture canola. For canola in peaola treatments 1 and 6 we found that it had significantly less (P < 0.05) nitrogen in its leaf tissue than monoculture pea. For the samples collected from the Greene Farm, we also found no significant differences (P < 005) or trends (0.05 ≤ P ≤ 0.1) between the pea and canola in the intercropping system (Figure 13). Comparisons between intercropped and monoculture pea and canola were not able to be made at the Greene Farm as monoculture trials were not planted for both crops.
Discussion
Land Equivalent Ratio and Yield Analysis
It is not surprising that the peaola system did not appear to benefit from increasing the rates of synthetic N fertilizer, as previous work done on legume-oilseed intercropping has found similar results (Porter et al., 2020). Despite these results, more work needs to be done to determine if the peaola cropping system can be grown independently of N fertilizer application. Largely, this is due to there being a potential that N was not a limiting factor in the crops grown in this study. Therefore, future research will be addressing if N is being transferred from peas to canola via plant-plant-microbe interactions. This research will help us determine if these interactions are responsible for the increased LER with decreased synthetic N input of peaola. The relative yield of peas to canola, calculated based on monoculture pea and canola, showed that winter peaola did not strongly favor either pea or canola. Although some studies have found that pea is favored over canola in overyielding peaola, our data align with the majority of studies on peaola with an overall LER of 1.63 with neither pea nor canola being strongly favored (Fletcher et al., 2016). The crop grown in 2021 at the Greene farm likely experienced failure of its pea crop due to extreme weather conditions around the time of flowering. This is because heat is known to decrease yield in both pea and canola, especially around flowering (Mohapatra et al., 2020).
Microbial Community Analysis
Through our analyses of the α-diversity and β-diversity of the microbial communities in the soil and rhizosphere, we did not find that intercropping pea and canola had a large impact on the diversity of the microbial community alone. Despite this finding, we did find that peaola did have a unique core microbiome compared to pea and canola when grown in monoculture. Taken with our findings for α-diversity and β-diversity, it does show why we did not see a significant difference here as in the core microbiome there does appear to be a combining of the pea and canola microbiomes despite peaola's unique members. Therefore, it would make sense that the diversity would not be significantly different from pea and canola if there were not many unique members added to the peaola microbiome overall. Despite this, our findings that peaola does have unique core members does suggest to us that it is possible that peaola is creating a unique soil environment from pea and canola, potentially due to changes it is making to the soil chemistry. Further work will need to be done in order to confirm this. In addition, since changes were observed in the core microbiome, it is possible that some members of the microbial community are impacted by peaola in terms of their overall abundance. Therefore, we will be performing differential abundance analysis on our sequencing data in order to determine if there are any bacteria that are changing significantly in their overall abundance between cropping systems. This will be done in R version 4.2.1 in RStudio version 2022.07.0+548. Additionally, data on the abundance of the nifH gene across cropping systems from qPCR will also be insightful into how the microbial community is changing across cropping systems, as it will show us if there is a change in the abundance of bacteria with the ability to fix nitrogen.
The results from the sample taken in 2021 and 2022 do show that crop management practices do have a significant impact on microbial communities, especially when different seeding rates were used in peaola. From looking at the 2022 data, it appears that treatments 3, 6, and 8 had the most significant impact on the canola bacterial communities overall, as these communities were significantly richer than almost all of the pea bacterial communities across treatments. Interestingly, treatments 3 and 6 had the higher pea seeding rate tested. Treatment 8 had the mid-range pea seeding treatment, but the highest canola seeding rate. Analysis of the core microbiome will be helpful in determining what is happening in these systems, as it is possible that bacteria from the pea core microbiome could be entering the canola core microbiome in these treatments. Differential abundance analysis will also be useful here in determining which bacterial taxa are shifting. Knowing which taxa are shifting in the microbiome will allow us to determine if these bacteria are playing a role in nutrient cycling and therefore are potentially contributing to overyielding in peaola.
Network Analysis
Based on the changes in the number of nodes and edges found in the networks from pea and canola to peaola, it is clear that there is a change in the structure of the microbial community. There is also the potential that this is demonstrating the combining of the pea and canola microbial communities in peaola as we saw through our microbial community analysis. Although there is useful information that can be extracted from these networks, we have decided to remake our networks with bacteria at the phylum level instead. This will allow patterns to become clearer in the networks, as this reduces the number of nodes. Further investigation will then be done into phyla that are highly connected in the network to determine the microbial members present in each phylum and their predicted ecological role.
Stable Isotope Analysis
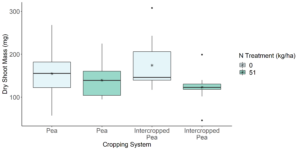
From our analyses of our stable isotope data, it appears that the seeding rate of pea and canola in peaola does have a significant impact on the N content of pea and canola. Although no difference was seen between pea and canola N content in 2020, in 2022 pea was found to have significantly more N than canola. In peaola, this trend was no longer observed. This suggests that there could be an exchange of nutrients between pea and canola, and in some treatment groups there could be better exchange of N from pea to canola. For example, in seeding treatments 1 and 6 canola was found to have significantly less N content than monoculture pea, but pea was found to have significantly more N than monoculture P. In the other seeding treatments, canola and pea N content was not significantly different from monoculture pea in either direction. With that said, it is possible that in seeding treatments 1 and 6 that pea could be withhold more N for itself and even competing with canola, which is why we see an increase in N content from monoculture pea, but a maintained pattern of canola having significantly less N content than monoculture pea. With that said, greenhouse experiments will be necessary to determine if there is transfer of N from pea to canola. Preliminary results from a greenhouse experiment we performed measuring the dry shoot mass of pea and canola across cropping systems suggests that pea is providing N to canola while experiencing no negative consequences to its own success.
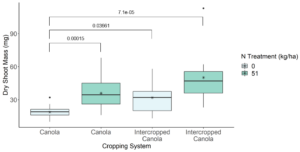
This is because we saw that there was no significant difference in the dry shoot mass of pea across cropping systems both with and without a N treatment (Figure 10). When looking at canola, we did see that the dry shoot mass of canola when grown with pea was significantly greater than canola grown in monoculture with no N treatment (Kruskal–Wallis Test, n1 = 11, n2 = 20, P = 0.0366), but was not significantly different from canola grown in monoculture with a N treatment (Kruskal–Wallis Test, n1 = 11, n2 = 20, P = 0.445) (Figure 11). Therefore, further investigation will need to be done in the greenhouse to determine how pea and canola are interacting in order to confirm these results were the results of N nutrient transfer between plants.
Sources
Dowling A, Victor SO, Penny R, Ashlea D, Yi Z, Matthew DD. Legume-oilseed intercropping in mechanised broadacre agriculture – a review. Field Crops Research. (2021) 260:107980. doi: https://doi.org/10.1016/j.fcr.2020.107980
Fletcher AL, Kirkegaard JA, Peoples MB, Robertson MJ, Whish J, Swan AD. Prospects to utilise intercrops and crop variety mixtures in mechanised, rain-fed, temperate cropping systems. In: Crop and Pasture Science, Vol. 67. CSIRO (2016). p. 1252–67. doi: 10.1071/CP16211
Mohapatra C, Chand R, Tiwari JK, Singh AK. Effect of heat stress during flowering and pod formation in pea (Pisum sativum physiology L). Mol Biol Plants. (2020) 26:1119–25. doi: 10.1007/s12298-020-00803-4
Porter MJ, Pan WL, Schillinger WF, Madsen IJ, Sowers KE, Tao H. Winter canola response to soil and fertilizer nitrogen in semiarid mediterranean conditions. Agron J. (2020) 112:801–14. doi: 10.1002/agj2.20119
Research Outcomes
Education and Outreach
Participation Summary:
A journal article was published on June 30th in Frontiers in Soil Science that detailed the findings of the microbial community analyses along with data collected by Dr. Isaac Madsen on crop yield, land equivalent ratio, and N response. This article can be found at the link and has the DOI: 10.3389/fsoil.2022.818862. Two separate conferences were also attended. One was WA SoilCon, where a recorded lightning talk was given on the findings for the microbial community analysis
In the Fall of 2022, a virtual talk was given at the WSU Farmer’s Network Soil Health Coffee Hour presenting the results that we had at the time of the talk. The Western SARE survey was distributed to attendees, but none were returned. So far in 2023, a poster presentation was given at the WSU Plant Science Retreat in Pullman, WA that showed results for our analysis of the core microbiome MPS_Retreat_Poster. This poster also included data from greenhouse experiments done to determine if N is being transferred from pea to canola. A review article covering the role of plant-plant-microbe interactions on intercropping success was just submitted to Plant and Science for publication in the special issue they are putting together in intercropping. In this review the manuscript published in Frontiers in Soil Science DOI: 10.3389/fsoil.2022.818862 in 2022 was cited. This grant was cited in that review as a source of funding. An article was wrote about Janice Parks by her undergraduate institution where some of the findings from this project, along with her recent publication, were discussed (Alumna Janice Parks '20 Builds On Pacific Research Foundation | Pacific University). This article was sent to all subscribers of Pacific University's magazine/newsletter and was shared via Pacific University's LinkedIn page.
Currently, there are plans to do public outreach events such as distributing informative brochures to local businesses in the Pullman-Moscow area. There are also plans to publish a 'Timely Topic" with the WSU Wheat and Small Grains program on their website, to do a live interview on the “Wheat Beat” podcast hosted by Dr. Drew Lyon, to give a talk or poster at the Pacific Northwest Direct Seed Association annual conference and at a WSU Farmers Network or WOCS events, to publish an abstract to the WSU Dryland Field-day Abstract reference guide, to publish a reviewed WSU extension fact sheet and an article into Crops and Soils magazine, to work with the “Visualizing Microbial Agroecology” Western SARE PDP to create animations of the functional roles of the microbiome in peaola, to give two 40-minute webinar videos hosted on the famersnetwork.wsu.edu and broadcast via the css.wsu.edu/oilseeds, to present at the tri-societies, regional soil health and/or a like meeting, and publish manuscripts to journals such as Plant and Soil, Applied and Environmental Microbiology, and/or other like journals.