Final report for LNC20-435
Project Information
Our research project will develop and deliver cultural post-harvest management tactics for fruit wastes utilized by the invasive pest, spotted wing Drosophila (Drosophila suzukii) (SWD). SWD is the number one insect pest of cherries in the NCR and is currently managed with repeated application of broad-spectrum insecticides. The waste fruit disposal methods developed will include composting and fruit crushing. Preliminary research conducted by our team demonstrates that SWD readily reproduces on waste fruits and that composting fruit wastes with animal manures, introduction of black soldier fly and/or crushing fruit wastes reduce SWD emergence by up to 100%. Project activities will evaluate these tactics at field scale, evaluate the potential of soluble nitrogen in place of animal manures, evaluate the feasibility of using BSF to compost field wastes, demonstrate the tactics to regional fruit growers and develop extension products highlighting these cultural control tactics. Our project meets all three desired outcomes for NCSARE research and education projects. Developing alternatives to costly insecticide applications and integrating cultural/sanitation pest management methods for SWD will improve farmer profitability, by reducing production costs and crop losses. Reduced reliance on broad spectrum insecticides will improve the environmental quality and natural resource base within cherry production systems by conserving biodiversity. Reduced reliance on broad spectrum insecticides and improved profitability will enhance the quality of life for farmers, farm workers and society at large by reducing insecticide exposure as well as by preventing the loss of historically important tart cherry orchards from NW Michigan.
1): Determine feasibility of fruit crushing/cultivation/composting as cultural control for SWD on fruit wastes.
2): Determine the impact of soluble nitrogen fertilizers and black soldier fly on SWD reproduction in fruit wastes.
3): Develop and demonstrate optimized practices utilizing combined tactics for managing SWD populations in fruit wastes.
4): Deliver optimized practices to grower clientele
Learning Outcome: Improved understanding of how post-harvest waste management impacts SWD populations and methodology for waste disposal using in field crushing or composting.
Action Outcome: Growers will adopt post-harvest management strategies to reduce local SWD populations, reducing the need for broad spectrum pesticide applications.
Michigan tart cherry growers are the immediate stakeholders served by our project team. However, the project will be applicable to soft fruit producers across the NCR and USA. The project team has a well-developed relationship with the fruit industry in MI and regional commodity groups funded the development of our preliminary data. While our research has strong regional support, it directly addresses the management of SWD, an insect that is an identified stakeholder priority of the North Eastern, Southern, North Central and Western IPM centers as outlined in numerous pest management strategic plans. Furthermore, all major fruit commodities that have published Pest Management Strategic Plans (PMSP) (sweet and tart cherries, blueberries, raspberries, strawberries and grapes) report the development of new pest management tactics to replace outgoing insecticides as an important priority.
Our proposal will develop cultural management tactics for Spotted wing Drosophila (Drosophila suzukii) (SWD) that can be integrated into existing IPM programs. We will provide farm scale proof of concept for these sanitation techniques as well as evaluate novel sanitation techniques. Michigan is the leading producer of tart cherries with 32,500 acres, and SWD has rapidly become the biggest economic challenge faced by this important NCR crop. Post-harvest management of fruit wastes is important because tart cherry growers are routinely required to set aside up to 40% of fruit due to marketing orders and fruit that is set aside serves as an important resource for SWD development. Post-harvest management will reduce local SWD populations within a growing season and the number of SWD entering the overwintering period. Ultimately, this will reduce applications of broad-spectrum insecticides to fruit just prior to harvest. Incorporating sanitation based cultural controls into SWD management programs will help growers meet Maximum Residue Limits (MRLs) and minimize negative impacts on beneficial organisms —i.e. bees, pollinators and natural enemies of key pests.
Our project will complement previous SARE projects in two areas: 1) sustainable control of SWD (15 funded projects) and 2) exploration of black soldier fly (BSF) as an on-farm waste management tool (7 funded projects). SARE funded SWD projects have ranged from evaluating protective culture, attract and kill, mass trapping and botanical insecticides. SARE funded work on BSF has focused on their use as animal feeds and composting farm wastes. Our project will complement these studies by providing farm scale proof of concept data on cultural sanitation approaches to post harvest management of SWD and explore the use of BSF larvae as interference biocontrol.
Need/Problem: Since 2008, SWD has become a global pest of berries and stone fruits and is found throughout the USA with annual damage and control costs in the HUNDREDS of millions of dollars (Bolda et al. 2010). The keys to this pest’s rapid rise to critical importance lie in its unique ovipositor, allowing it to insert eggs into fruit, rapid generation time (as short as 8 days) and broad host range (Lee et al. 2011). Perennial fruit crops threatened by SWD are important to the US economy, with a market value of $11.7 billion, plus they provide important nutrients and are valuable components of local food systems. In Michigan, the Tart Cherry and Blueberry industries have an annual farm gate value of $40 Million and $116 Million, respectively (USDA NASS 2017). Invasive pests notwithstanding, pest management is consistently listed as a prime concern for fruit growers, and insecticides alone can account for as much as 13% of yearly production expenses (Perez & Ali, 2009). Insect pest management in United States fruit production systems has faced unprecedented challenges in the last decade. These challenges include: the loss of insecticides through revised EPA regulations (http://www.epa.gov/opp00001/reregistration/azm/), rapid development of pest resistance to key insecticides (Gress and Zalom, 2018), increasing public concerns about negative impacts associated with insecticides (Damalas & Eleftherohorinos, 2011), and the promulgation of international MRLs (http://www.fas.usda.gov/maximum-residue-limits-mrl-database).
Post harvest management provides an opportunity for cultural control: The establishment of sustainable IPM programs for SWD demands that we develop non-pesticide based management tactics. Currently, the most successful non-pesticidal based management tactic has been protecting small fruit crops with insect mesh. While exclusion netting can provide significant reductions in SWD damage it is expensive (~$30,000 per acre) and not easily adapted to existing plantings (Leach et al. 2016; Rogers et al. 2016). Bal et al. (2017) demonstrated that SWD readily reproduces in unharvested fruit and fruit processing remnants, but little attention has been paid to post harvest management. Removal of fruit resources and sanitation can reduce SWD local populations. For example, Leach et al (2018) demonstrated that increased harvest frequency and removal of dropped or waste fruit reduces populations by up to 60%. Burial of wastes has also been evaluated but research suggests that shallow burial is not effective at farm scales with depths of at least 24 cm (10 inches) needed to achieve 95% emergence reductions (Hooper and Grieshop, 2020).
Preliminary Data: The project team has previously demonstrated that crushing and composting of fruit wastes significantly reduces SWD reproduction under laboratory and semi-field conditions. A series of 2016 experiments conducted by Dr. Rothwell and Ms. Pochubay evaluated mechanical, in-field crushing of post-harvest tart cherries, demonstrating reduced duration and overall level of SWD reproduction. Likewise, experiments conducted in Dr. Grieshop’s program have shown that composting apple pomace (apple cider leftovers) with relatively small volumes (<25%) of poultry manure can reduce SWD utilization of this common fruit waste by over 95%.
Fruit crushing was evaluated in 2016 by collecting ripe, unsprayed, Montmorency tart cherries into small windrows in the center of the sod row middles. A golf cart was used to smash windrows by driving over them twice and samples of smashed and intact cherries were collected 1, 3, 5, 7 and nine days afterwards and exposed to adult SWD in the lab. Crushing fruit in the orchard reduced the number of SWD larvae at all timings, with 100% reductions recorded for fruit by day 7 and 9.
KNOWLEDGE GAP 1: While preliminary data demonstrates that crushing fruit reduces SWD reproduction, this tactic has not been evaluated at a scale relevant to farming operations nor have we evaluated whether crushing reduces local populations of wild flies. We will address this Gap in objective 1. |
Composting fruit wastes with animal manures has also shown promising results in laboratory and field experiments. A laboratory experiment evaluated five mixtures of organic apple pomace (cider leftovers) with organic poultry chicken manure (100, 99, 95, 90, and 80% pomace). Poultry manure significantly affected SWD reproduction with 19.2%, 34.3%, and 81.8% reductions in the 95%, 90% and 80% apple pomace treatments, respectively (Hooper and Grieshop 2019). A field experiment with similar pomace/manure mixtures (100%, 90%, 75%, 50%, and 0% pomace) in 100 liter, caged experimental arenas had similar results. There was a significant effect for the quantity of apple pomace on SWD reproduction with emergence decreasing by 76.3%, 95.0%, 95.9%, and 99.4% for treatments containing 90%, 75%, 50%, and 0% apple pomace, respectively. These results further support the hypothesis that adding poultry manure to fruit wastes significantly reduces the reproductive quality of these wastes.
KNOWLEDGE GAP 2: Composting waste fruit with poultry manure reduces both laboratory and field SWD reproduction at small scales. This tactic has not been evaluated at the farm scale nor have we evaluated the impact of composting fruit wastes on local SWD populations. We will address this Gap in objective 1. |
Chicken manure is high in nitrogen, and high nitrogen substrates decrease oviposition and egg viability of SWD and D. melanogaster (Joshi et al., 1997; Belloni et al., 2018). Belloni et al. (2018) determined that high concentrations of dietary urea and ammonia reduced SWD oviposition by 70% and 60%, respectively. If nitrogen content is the causal mechanism behind the observed reductions in reproduction then growers could potentially use nitrate or ammonia based fertilizers in place of chicken manure.
KNOWLEDGE GAP 3: Nitrogen content may explain how the addition of manure reduces SWD reproduction. Soluble nitrogen sources are cheaper and easier to handle compared to manures and could be used to manage fruit wastes on planting floors or in canopies. Thus, determining the form and levels of N needed to reduce SWD reproduction in fruit wastes is the 1st step in developing this tactic. We will address this Gap in objective 2. |
Black soldier flies (Hermetia illucens) (Diptera: Stratiomyidae) (BSF) are a non-pestilent insect used for biological control of stable and house flies in manure management systems (Sheppard, 1983, Miranda et al. 2019). We have conducted lab experiments evaluating the impact of BSF composting of fruit wastes on SWD reproduction. 200 ml of apple pomace was exposed to SWD adults for 48 hours and BSF larvae added at day 0 (at the same time as SWD), 3 (1 day after SWD) and 7 (5 days after SWD) or not at all (control) after which SWD offspring were collected on a daily basis. The addition of BSF at day 0 completely eliminated SWD reproduction and provided a 10-fold and 3-fold reduction when BSF were added to wastes on day 3 and 7, respectively. BSF can be readily purchased from a variety of sources and may provide another attractive alternative for managing SWD populations in fruit wastes.
KNOWLEDGE GAP 4: How can BSF be best leveraged as a post-harvest control strategy for SWD and other insect pests that utilize fruit wastes? The first step of this process is to understand the biological mechanisms behind observed reductions. The next step is to develop a “dose-response” curve and the final step is to evaluate and demonstrate this tactic at field scale. We will address this Gap in objectives 2 and 3. |
Integration of pest management tactics and strategies is a core IPM principle and one that is desperately needed for the development of sustainable SWD management programs (Lee et al., 2011.; Sial et al. 2018). While the development of a fully integrated program is outside the scope of this project, the development of optimal integrated waste management strategies will provide a vital step forward to this ultimate goal. Furthermore, our grower collaborators have identified management of sap beetles (Coleoptera: Nitidulidae) as an additional post harvest concern.
KNOWLEDGE GAP 5: The effects and practicality of integrating multiple post-harvest fruit waste management tactics for reducing SWD and other pest insect reproduction are unknown. The combination of fruit crushing with either manure or soluble nitrogen applications may provide synergistic reductions in fly populations but the potential costs/benefits associated with integration of these processes is unknown. We will address this Gap in objective 3. |
Cooperators
Research
1) Crushing and manure applications will reduce SWD reproduction in waste cherries
2) Nitrogen fertilizers will reduce SWD reproduction in waste cherries
3) Black soldier fly will reduce SWD reproduction in waste cherries.
Approach and Methods: Activities for objectives 1 and 3 will involve extensive on farm, grower collaborative work. Objective 2 will involve largely laboratory proof of concept experiments used to inform objective 3. We will meet regularly throughout the project with our collaborating growers to provide updates and to co-create field methodology and extension outputs. A project timetable is presented in figure 7.
Objective 1. Evaluate and compare farm-scale feasibility of fruit crushing/cultivation and composting as cultural control tactics for reducing SWD and other drosophilid populations on aggregated post-harvest tart cherry wastes: Our farmer collaborators have expressed a need for “field scale” data on crushing and composting of fruit wastes before they will adopt these practices and have suggested that we monitor additional problem insects that build on waste cherries such as sap beetles. To address these needs we will evaluate the two tactics on waste tart cherries at the MSU Northwest Michigan Horticultural Research Center (NWMHRC) and three collaborating farm/fruit processing locations in years 1 and 2. Shoreline Fruit Growers, Garthe Farms, Cherry Bay Orchards, and Smeltzer Orchard Company will provide field locations, waste cherries and farm labor/equipment to establish experiments. The project team is requesting $30,000 over the course of the project to offset costs to our grower collaborators.
Experiment 1.1 We will demonstrate and evaluate fruit crushing and composting at farm scale at our four field sites. The experiment will consist of four treatments; an untreated control, 15% and 30% poultry manure composting treatments (by volume) and mechanical crushing of fruit with a tractor mounted roller or flail mower with four replicates per site. Experimental units will consist of 100-300 liter (0.1-0.3 cubic meter) quantities of waste cherries. Fruit wastes will be mixed with poultry manure with a front loader or similar tractor-based implement. SWD and other insect development will be monitored using a combination of emergence cages, laboratory assessment of emergence from sub-samples, and a trapping grid. Waste piles will be separated by at least 20 m and placed in an open field adjacent to fruit production or processing sites.
We will begin experiments in late July or early August and terminate them after 10 weeks. Adult SWD and other insect emergence will be monitored using emergence cages and weekly fruit samples. A 60 cm x 60 cm x 60 cm mesh emergence cage (Fig. 8) will be placed on the center of each waste pile and adult fruit flies and other insects collected on a weekly basis. Five, 200 ml fruit samples will be collected from each pile and adult fruit flies reared out in laboratory emergence arenas and checked daily for 5 weeks or until no emergence is detected for 7 days. To assess impacts on SWD density within the treated area, three 946 ml deli cup SWD traps baited with a commercial lure will be situated around each pile with one trap directly over fruit wastes and two traps placed within 5 m of each waste pile. We will record the number of male and female SWD, number of Drosophila melanogaster and other drosophilids and sap beetles at the family level. Data will be analyzed with repeated measures MANOVA with treatment as a fixed factor and block and time as random factors.
Objective 2. Determine the impact of soluble nitrogen fertilizers and black soldier fly on SWD reproduction in fruit wastes.: When presented with preliminary data on management of SWD on post harvest wastes our grower collaborators requested that we evaluate soluble fertilizers as these products are less expensive, more readily available and require less machinery for handling. Our grower collaborators also expressed interest in evaluation of BSF composting. However, before these approaches are ready for field testing, proof of concept work needs to be conducted.
Experiment 2.1 will determine the effect of soluble synthetic nitrogen on SWD reproduction on waste cherries and Experiment 2.2 will determine if soluble nitrogen on fresh tart cherries will reduce SWD oviposition. Experiment 2.3 will determine the optimal “dose” of black soldier fly (BSF) to use per liter of waste cherries to minimize SWD emergence. Waste cherries will be collected and stored in a walk in freezer located on the MSU campus. Fresh cherries will be purchased as needed from local produce suppliers. Laboratory trials will be conducted in growth chambers located at the MSU insectary and Department of Entomology during the fall, winter and spring months.
Experiment 2.1: In fall 2021 we will evaluate five rates of urea, ammonium, nitrate and calcium nitrate, an untreated control and a positive control containing 20% (by volume) organic chicken manure treatments with at least ten replicates. Nitrogen treatments will be assigned based on the estimated percentage nitrogen and density of chicken manure at 25% moisture –5.415% and 1.08 g/ml, respectively. We will evaluate the three fertilizers based on the mass of Nitrogen estimated to be contained in 10%, 25%, 50%, 100%, and 150% chicken manure/fruit blends. For example, for a 46% Urea fertilizer the resulting mass of fertilizer added to 200 ml of fruit waste would be: 1.18 g, 2.95 g, 5.9 g, 11.8 g, and 17.7 g, respectively. Experiments will use emergence arenas described in Experiment 1.1 (Fig. 9). Ten, 7 d old female adult SWD will be placed in each arena and allowed to oviposit for 48 h after which they will be removed with an aspirator. Arenas will be held in a growth chamber (25˚C, 70% RH, 16:8 L:D), and checked daily for emergence and flies counted and removed for five weeks or after 7 days without emergence. Data will be analyzed utilizing a repeated measures ANOVA with nitrogen source (fertilizer) and rate as fixed factors and experimental run and collection date as random factors.
Experiment 2.2: We will determine the impact of soluble nitrogen applications to fresh cherries on SWD oviposition and development. We will evaluate at least five fertilizer types/rates (identified in Experiment 2.1) tested in choice bioassays. The bioassays will be conducted in a 25 cm (H) x 25 cm (D) x 75 cm (W) arena containing untreated and treated fruit. Fertilizers will be applied by dipping five cherries into solution. The five treated fruits and a second set of water dipped fruit (control) will be placed at opposite ends of the arena and 10 7 d old, mated female SWD released into the arenas. Flies will be allowed to interact with fruit for 24 hours and presence on fruit recorded throughout the experiment. Fruits will be collected, oviposition scars counted using a stereomicroscope and offspring from the fruits reared out and counted. Fly count data will be analyzed utilizing a repeated measures ANOVA with nitrogen source (fertilizer rate) and rate (% chicken manure equivalent) treatment as fixed factors and experimental run and observation time as random factors. Oviposition scar and offspring data will be analyzed utilizing an ANOVA with nitrogen source (fertilizer) and rate treatment as fixed factors.
Experiment 2.3: We will determine an optimal dose of BSF larvae (g of larvae/l of waste) for disrupting SWD reproduction in waste cherries. Laboratory experiments will be conducted in arenas containing 2 l of cherry waste with 5 rates of BSF evaluated (0.1, 0.3, 1, 3 and 10 g per l of waste) and an untreated control and replicated 5 times. Arenas will consist of aluminum baking pans placed within a mesh cage. Wastes will be infested with SWD by introducing 100 SWD mated females to the arenas 48 hours in advance of introducing BSF as 3rd instar maggots. Arenas will be sampled and data analyzed as outlined in Experiment 2.1. This experiment will be carried out between September 2021 and April 2022 and used to inform Experiment 3.3.
Objective 3. Develop and demonstrate optimized practices utilizing combined crushing, manure and/or nutrient inputs for managing SWD populations in post-harvest fruit wastes in field or orchard plantings: The ultimate goal of our project is to provide NCR tart cherry growers with an integrated set of tactics for post harvest management to reduce SWD use of these resources. In the final field season of the project we will address this in a series of three on farm experiments conducted with grower collaborators at the NWMHRC and 3 grower locations.
Experiment 3.1: We will evaluate combinations of crushing, composting and soluble nitrogen applications on SWD, sap beetle and other insect development at our 4 field sites. Waste fruit piles will be established as described in Experiment 1.1 and treatments replicated at least four times per site. We will evaluate at least 5 treatments consisting of crushing fruit with a turf roller or flail mower, mixing fruit with manure at the optimal rate identified in Experiment 2.1, mixing fruit with the optimal rate of the best soluble nitrogen fertilizer as identified in Experiment 2.1, a combination treatment of crushing and manure, and a combination treatment of crushing and soluble nitrogen application. Data will be collected and analyzed as described in Experiment 1.1.
Experiment 3.2: We will determine the impact of soluble nitrogen applications to the canopy and floor of tart cherry orchards on SWD reproduction, in an experiment consisting of five treatments replicated four times in a randomized complete block design at our four field sites. Treatments will consist of an untreated control and three application rates of a single soluble nitrogen source that will be determined based on Experiment 2.2. Nitrogen will be applied utilizing an optimized radial air blast sprayer using a spray volume of 468 l/ha (50 gallons per acre) to 1/10 ha experimental blocks once per week over a four week period. Data will be collected utilizing three yeast-sugar baited 946 ml deli cup traps per experimental plot and fruit samples (see Experiment 1.1) . Three 200 ml samples per plot will be collected from the canopy and floor for five weeks or until fruit is no longer available. Samples will be reared out in growth chambers using deli cup arenas described in objective 1.1 (Fig. 10). Data will be analyzed utilizing a repeated measures MANOVA for each location with treatment as a fixed factor and block and time as random factors.
Experiment 3.3: We will evaluate the impact of BSF on SWD and other insect development in waste cherry piles at our four field sites. This experiment will consist of three to four treatments based on the outcomes of Experiment 2.3 and be carried out at the NWMHRC and at 3 grower locations. Waste fruit piles will be established as described in Experiment 1.1 and treatments replicated at least four times per site/fruit. 3rd Instar BSF will be placed within the piles at 2-3 rates plus an untreated control. SWD and pest insect data will be collected and analyzed as described in Experiment 1.1.
Objective 4. Extend project information to growers and pest management industry: Outreach and extension will be conducted throughout the project through: biannual meetings with our grower collaborators, the development of bulletins and publications, delivery of materials at regional grower meetings and at project specific field days and webinars. Activities for this objective are documented in the “Outreach” section.
Pitfalls : Our research has two expected pitfalls: crop availability and interdependence between our lab work and field work. The first issue faces all research in fruit crops in the NCR region, because of perennial crop vulnerability to climate change induced crop failures. If we experience regional crop failures, we will seek out alternative fruit and vegetable wastes to work with. The second issue applies to Experiments 2.1-2.3. If laboratory work does not provide satisfactory results, we will work with our growers to develop replacement field trials/demonstrations on the proven management techniques of crushing and composting.
Note that Entomology Masters degree student Charlotte Schuttler was supported via this project, and results and discussion for Objectives 1 and 2 have been published in her thesis which is available here: https://d.lib.msu.edu/etd/51544. Detailed methods, results, and discussion are available there.
Experiment 1.1: Experiment 1.1 was conducted at seven locations across five farms in 2021 and five locations across three farms in 2022.
2021 Methods: Treatments were adjusted to include (1) crushing, (2) incorporation of 25% manure by volume; and (3) incorporation of 15% manure by volume compared to (4) an untreated control. Granulated organic chicken manure obtained from Herbruck’s Poultry Ranch, Inc. (Saranac, MI) was used for manure containing treatments. At each location, cherries were measured by volume with 5-gal plastic buckets and dumped into four piles. Each pile consisted of 95 L of cherries (~73.5kg). Piles were spaced at least 100 m apart. For crushing treatments, cherries were crushed with tractor tires until 90% of fruit was macerated then fruit was raked back into a pile. For manure treatments poultry manure was measured and applied to waste piles. Treatments with 15% manure had 13.2 L of water added, and treatments with 25% manure had 26.5 L of water added. All other treatments had 13.2 L of water added to them. All waste piles were mixed for approximately 4 min with hand tools after treatments were applied to either incorporate manure or to maintain conditions comparable to manure incorporation.
To capture insects emerging from fruit waste, each pile was capped with an emergence cage (Bugdorm, Talchung, Taiwan) measuring 60 cm L by 60 cm W by 60 cm H, secured at four points with ground stakes. The whole experiment took place between July 15th and August 24th, 2021, with all sampling starting on July 22nd, 2021. The inside of each emergence cage was vacuumed twice weekly using a handheld aspirator (BioQuip Products, Inc., Rancho Dominguez, CA). Aspirated samples were frozen for at least 24 h, after which the number of male D. suzukii, female D. suzukii, non-suzukii drosophila, and sap beetles (Coleoptera: Nitidulidae) were counted under a stereomicroscope. To monitor insects on and around fruit waste piles, we used three yeast-baited cup traps. All traps were suspected from 0.91m stakes and placed either directly next to each fruit waste pile (trap E) or 10 m from the pile in opposite directions (traps N & S). Yeast trap contents were collected weekly, filtered, and D. suzukii, non-suzukii drosophila, and sap beetles were sorted and counted.
2021 Results: Analyzed means of D. suzukii collected in vacuum samples during 2021 (Figure 1.1A) showed significant effects of treatment (F3,138=4.29, p=0.0063), week (F5,138=4.79, p=0.0005) and their interaction (F15,138=2.03, p=0.0170). Capture was not significantly different between treatments from week 1 to week 3. From week 3 to week 6, significantly more D. suzukii were collected from the control treatment than in crushed, 15% manure, or 25% manure treatments. Highest overall capture occurred in week 4 of the experiment and an average of 143 ± 96, 2 ± 0.2, 3 ± 0.8, and 3 ± 1.4 adult D. suzukii were collected from the control, crushed, 15% manure, and 25% manure treatments, respectively.
The most D. suzukii per week were captured in cup traps associated with 15% manure (26.4 ± 0.2) treated piles as compared to crushed cherries (14.4 ± 0.2) or control (15.1 ± 0.2) treatments(treatment: F2,234=3.90, p=0.0095). The number of D. suzukii caught in 25% manure (18.7 ± 0.2) and 15% manure (26.4 ± 0.2) treatments were not significantly different from each other. Drosophila suzukii capture increased from week 1 to week 3, declined in week 4, and increased again in week 5 (week: F4,234=57.62, p<0.0001). There were no interactions between experimental week and treatment (F12,234=0.62, p=0.8240). In 2021, nearly twice as many D. suzukii were captured in cup traps placed on piles (10,532) than in traps placed 10m away (5896) from piles (F1,234=4.14, p=0.0429) with no interaction between treatment and trap placement (F3,234=1.80, p = 0.1486).
2022 Methods: In addition to the treatments compared in 2021, the following treatments were added during 2022: (5) A D. suzukii seeded control and (6) a urea fertilizer treatment. Urea was added with the intent to isolate the effect of nitrogen addition on D. suzukii infestation. The 2022 experiment took place between July 22-August 24th, 2022. Sampling began on July 25th, 2022. Yeast cup captures were used to assess insects near fruit piles following the same methods as in 2021. To measure insects emerging from piles within cages, vacuum samples were collected as in 2021, and in addition, a single yellow sticky card was hung inside each cage and was replaced weekly. Sticky cards were stored at -20°C after collection until the number of D. suzukii, non-suzukii drosophila, and sap beetles could be enumerated.
2022 Results: Vacuum captures of D. suzukii in 2022 did not differ between treatments (F5,111=1.42, p=0.2226), week (F4,111=0.87, p=0.4852), and there was no interaction between week and treatment (F20,111=0.68, p=0.8417). Sticky traps were added within emergence cages in 2022 as an additional form of data collection. Numerically, more D. suzukii were caught in sticky traps (2256) than in vacuum samples in 2022 (63). Based on catch from vacuum samples in 2021 (1685), it is likely that the addition of the sticky card inside the tent interacted with vacuum capture in 2022. Analyzed sticky trap capture of D. suzukii in 2022, showed that treatment (F5,88=14.64, p<0.0001), week (F3,88=14.56, p<0.0001), and their interaction (F15,88=2.66, p=0.0023) were significant. Similar to vacuum capture in 2021, for the first 2 weeks of the experiment there was no significant differences in capture between treatments, but in week 3 there were more D. suzukii caught in the control treatment than in all other treatments. In week 3, an average of 98 ± 40, 37.3 ± 11.3, 15.7 ± 7.9, 21.5 ± 7.5, 5 ± 1.9, and 3.1 ± 0.9, adult SWD were collected from the control, seed control, crushed, urea, 15% manure, and 25% manure treatments, respectively.
A similar pattern was observed in 2022 cup traps where treatments containing manure had the highest D. suzukii captures (15%: 65.8 ± 19.3 ; 25%: 65.3± 21.6). (treatment: F5,180=16.23, p<0.0001). There was no significant difference between control (5.2 ± 0.9), seeded control (15.0 ± 3.8), crushed cherry (10.4 ± 2.4), and urea treatments (4.6 ± 1.1). D. suzukii capture increased from week 1 to week 3 and declined in week 4 (week: F3,180=31.83, p<0.0001), but there was no interaction between week and treatment (F15,180=0.87, p=0.6036). There was a significant interaction between trap placement and treatment in 2022 (location*treatment: F5,180=3.70, p = 0.0032), but this only affected the 15% manure treatment in that more flies were captured in traps located on the pile. Traps placed on and off piles captured similar numbers of flies for all other treatments.
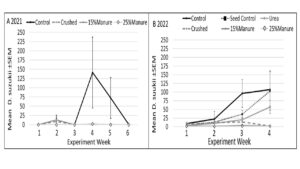
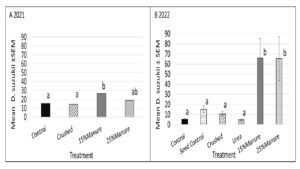
Experiment 1.1 Discussion: This research supports previous studies suggesting incorporation of poultry manure or crushing as treatments to decrease D. suzukii emergence from post-harvest waste cherries. Crushing cherries was found to be as effective at reducing Drosophila suzukii and non-suzukii drosophila species (data not included here but available in Schuttler thesis) as incorporating poultry manure at 15% or 25% by volume. Drosophila emergence from manure treatments was low throughout our experiments, but it took 2 to 3 weeks for crushed treatments to separate from untreated fruit. This delayed effect may be due to the time it took crushed cherries to break down to the point at which they were no longer able to support Drosophila spp. larvae.
Our different sampling methods provided different types of information with respect to all our focal insects. Cup traps were placed directly on waste fruit piles and around piles. These trap captures provide information on insects attracted both to fruit waste and to the traps themselves. Cup trap captures of D. suzukii were higher in 15% and 25% manure treatments than in the untreated control, while vacuum samples and sticky traps captures of insects within emergence cages were lower for these treatments. This may suggest that insects are more attracted to the trap lure than the manure treated fruit waste when in close proximity. Commercial cup trap lures which produce similar volatiles to the yeast baited traps used here were shown to attract D. suzukii within 93m. Therefore, it is also possible that our cup traps were capturing D. suzukii to the general area. With respect to monitoring insects emerging from waste piles within cages, sticky cards provided similar information to vacuum samples and were easier to use and assess.
We hypothesized that increased nitrogen in poultry manure was responsible for the reduction of D. suzukii. To test this hypothesis, we used granulated soluble urea as a synthetic nitrogen source at an amount equivalent to the nitrogen provided by 20% poultry manure by volume during our 2022 experiment. However, incorporation of urea fertilizer did not result in lower D. suzukii, other drosophila species, or sap beetles in waste fruit when compared to manure treatments or crushing. Future research should investigate other nitrogen-based fertilizers as a synthetic alternative for manure composting as well as seek to quantify microbial communities throughout fruit decomposition in treated and untreated fruit.
None of the treatments we investigated reduced sap beetle populations (data not shown but available in Schuttler thesis).
Experiment 2.1: Pomace from organically grown apples was obtained from a certified organic orchard and cidery in Flushing, MI. Prior to experiments, apple pomace was frozen at -20ºC for a minimum of one week prior to use to eliminate any previous arthropod infestation. Apple pomace was thawed to room temperature prior to use in experiments. Apple pomace mixed with poultry manure at 20% by volume in previous experiments had 1.5g N/ 250ml pomace, which is used as the ‘100%’ value in this experiment. To assess effects of nitrogen added to infested fruit waste, five soluble nitrogen fertilizers were each dissolved in 10ml deionized water at five different rates: 6%,12.5%,25%,50%, or 100% by volume. Treatment were replicated 5 times. Ten gravid female D. suzukii were allowed to lay eggs in each replicate for 48 hrs.
Arenas were placed in a growth chamber set to the same parameters used for the D. suzukii colony and checked daily for fly emergence. Emerging flies were removed using an aspirator, enumerated, and sexed. The experiment concluded when no D. suzukii emerged from any container for seven consecutive days.
Experiment 2.1 Treatments. Fertilizer sources, analysis, and dilution in 10ml water to achieve 1.5g N (100%).
Fertilizer |
Manufacturer |
Nitrogen (mg/L) |
Phosphorus (mg/L) |
Potassium (mg/L) |
6.25% (g) |
12.5% (g) |
25% (g) |
50% (g) |
100 % (g) |
Urea |
Easy Peasy Plants (Alvin, IL) |
46 |
0 |
0 |
0.20 |
0.41 |
0.82 |
1.63 |
3.26 |
Calcium Nitrate |
Envy (Chicago, IL) |
15.5 |
0 |
0 |
0.60 |
1.21 |
2.42 |
4.84 |
9.67 |
Potassium Nitrate |
Fireworks Cookbook LLC (Kathleen, GA) |
13 |
0 |
44 |
0.45 |
0.89 |
1.79 |
3.57 |
7.14 |
Magnesium Nitrate |
Greenway Biotech, Inc. (Santa Fe Springs, CA) |
11 |
0 |
0 |
0.85 |
1.70 |
3.41 |
6.82 |
13.63 |
Ammonium Sulfate |
Pure Original Ingredients (Lindon, UT) |
21 |
0 |
0 |
0.72 |
1.44 |
2.88 |
5.77 |
11.54 |
Poultry Manure |
Herbruck’s Poultry Ranch, Inc. (Saranac, MI) |
4 |
3 |
2 |
-- |
-- |
-- |
-- |
4.93* |
*Manure is measured as 20% by volume of total media (250ml)
Experiment 2.1 Results:
There was a significant effect of treatment (F25,100=9.73, p<0.0001) on the number of emerging D. suzukii. No D. suzukii emerged from treatments containing calcium nitrate, potassium nitrate, ammonium sulfate, or poultry manure. Very small numbers of D. suzukii emerged from treatments containing magnesium nitrate which was significantly lower than emergence in the untreated control. Urea treatments, except for the 50% rate, had similar D. suzukii emergence to the untreated control. The highest urea concentration did not significantly differ from the untreated control.
Mean emergence of D. suzukii (±SEM) from fertilizer treatments at 6.5%,12.5%, 25%, 50%, or 100%; control; or 20% poultry manure by pomace volume. Values indicated by the same letter are not significantly different, α=0.05.
Treatment |
Mean D. suzukii ± SEM |
||||
Control |
8.8 ± 2.3 a |
||||
Poultry Manure |
0.0 ± 0.0 c |
||||
|
6.5% |
12.5% |
25% |
50% |
100% |
Ammonium Sulfate |
0.0 ± 0.0 c |
0.0 ± 0.0 c |
0.0 ± 0.0 c |
0.0 ± 0.0 c |
0.0 ± 0.0 c |
Calcium Nitrate |
0.0 ± 0.0 c |
0.0 ± 0.0 c |
0.0 ± 0.0 c |
0.0 ± 0.0 c |
0.0 ± 0.0 c |
Magnesium Nitrate |
0.2 ± 0.2 bc |
0.0 ± 0.0 c |
0.0 ± 0.0 c |
0.0 ± 0.0 c |
0.0 ± 0.0 c |
Potassium Nitrate |
0.0 ± 0.0 c |
0.0 ± 0.0 c |
0.0 ± 0.0 c |
0.0 ± 0.0 c |
0.0 ± 0.0 c |
Urea |
6.8 ± 5.1 ab |
9.0 ± 4.2 a |
8.4 ± 4.2 a |
0.4 ± 0.4 bc |
4.8 ± 1.2 ab |
Experiment 2.2: Blueberries were utilized instead of cherries for this experiment due to fruit availability.
Experiment 2.2 Treatments. Fertilizer grams per solution percentage.
Fertilizer |
Amount of fertilizer (g) per 250 ml, 1.5 g N |
Amount of fertilizer (g) per 250 ml, 3.0 g N |
Urea |
3.26 |
6.52 |
Calcium Nitrate |
9.67 |
19.34 |
Magnesium Nitrate |
13.63 |
27.26 |
Ten blueberries per treatment were placed in a 15cm petri dish (VWR International LLC, Radnor, PA), and a dish containing fruit with each treatment was placed on the floor of an emergence cage held within a grow tent (2.7mx1.2mx2m, Vivosun, Ontario, CA) at 21˚C 70%RH, 16:8 L:D. Treatment placement was randomized in each cage to avoid position effects. Three-day old female (100) and male (100) D. suzukii from mixed sex colony vials were placed within a 9 cm petri dish (VWR International LLC, Radnor, PA) with dampened filter paper (No. 1, qualitative filter paper, GE Healthcare Bio-Sciences, Pittsburgh, PA) and released within the center of the cage. This choice experiment was replicated five times. After 48 hours blueberries were removed and dissected using a stereomicroscope to count eggs and larvae.
A second round of comparisons in which N rates for three fertilizers were doubled to 3 g per 250 ml were conducted following initial results (Table 2.2). Blueberries were treated as in the previous experiment, and treatments for choice assays were arranged in cages following the same methods. Female (60) and male (60) 3-day old D. suzukii from mixed sex colony vials were placed in cages using the same methods as for the prior experiment. The experiment was replicated across five cages, which were held under the same conditions as the previous experiment. After 48 h, blueberries were removed and stored in a fridge at 4˚C before dissection under a stereo microscope to count eggs.
Experiment 2.2 Results:
A similar number of D. suzukii eggs were laid across all treatments (F3,9=1.25, p= 0.3486). Doubling nitrogen rate did not change oviposition in treated fruit compared to untreated fruit (F3,12=0.6, p=0.625). Similar to observations in Experiment 2, the number of surviving adult D. suzukii did not differ between treatments dipped in different fertilizer solutions (F3,9=1.58, p=0.2607)
Experiment 2.2 Discussion: Our laboratory experiments suggest that nitrogen containing fertilizers may be a useful tool for controlling D. suzukii when incorporated in post-harvest waste, but that the fertilizers evaluated do not reduce egg laying or survival of subsequent larvae when applied to whole fruit preharvest.
When certain nitrogen-based fertilizers (calcium nitrate, potassium nitrate, ammonium sulfate, and magnesium nitrate) were incorporated into apple pomace, there was a notable reduction in D. suzukii survival. However, when urea was added to apple pomace, there was no reduction of D. suzukii compared to the control at almost all levels of nitrogen evaluated. All fertilizers were added to pomace at rates scaled to nitrogen levels in organic poultry manure. It was expected that if nitrogen is the main component affecting D. suzukii reduction in manure treated waste fruit, nitrogen-based fertilizers incorporated with waste fruit should yield similar results. Because all fertilizers were added based on similar rates of nitrogen, but results differed between fertilizers, it can be assumed that nitrogen is not the sole component specifically causing D. suzukii reduction within treated fruit waste.
Experiment 2.3: We investigated D. suzukii survival in substrates containing black soldier fly across multiple factors: infestation timing and infestation rate.
Infestation timing Experiment 2.3.1: Five infestation timing treatments were compared: simultaneous D. suzukii and H. illucens infestation, D. suzukii infestation 3 d after H. illucens infestation, D. suzukii infestation 7 d after H. illucens infestation, and D. suzukii alone. Each infestation timing was compared in both 250 ml (170g) organic apple pomace, or 90% organic apple pomace mixed with 10% organic poultry manure (Herbruck's Poultry Ranch, Inc., Saranac, MI, USA) by volume for a total of ten treatments. Treatments were replicated three times.
Infestation timing Experiment 2.3.2: Drosophila suzukii infested apple pomace was generated by placing ten seven-day-old presumed mated females from mixed sex colony vials in cages with the substrate for 48 h, after which all adult D. suzukii were removed. Three different H. illucens infestation timing treatments were applied to 250 ml of D. suzukii infested pomace (Figure 3.2). Three grams of approximately 15- day old (second instar) H. illucens larvae (five insects) were added on the same day as D. suzukii infestation, three days after D. suzukii infestation, or seven days after D. suzukii infestation. Two controls consisting of just H. illucens or just D. suzukii added on the first day of the experiment were also compared for a total of five treatments. All treatments were replicated five times.
Infestation rate Experiment 2.3.3: This experiment was conducted to compare D. suzukii egg and larvae survival when exposed to different rates of H. illucens infestation. To do this, we provisioned three different volumes of pomace, 170g (250ml), 340g (500ml), 680g (750ml), into 946 ml plastic deli containers (Deli-Serve, Chattanooga, TN) and then infested with 15, 30, or 45 pre-mated female D. suzukii respectively for 48 h. Each container was capped with a corresponding lid with two 2 cm holes, one hole covered with 150-micron polyester mesh (The Cary Company, Addison, IL) to allow for ventilation, and one hole covered with Parafilm (Bemis Company, Inc., Neenah, WI) to allow for aspirator insertion.
Five approximately 8-day old H. illucens larvae were added to each experimental arena. Positive controls consisted of each volume of pomace infested with only D. suzukii. Negative controls consisted of each volume of pomace with only 5 H. illucens added, for a total of nine treatments. Treatments were replicated four times, and there were thirty-six experimental arenas in total. Arenas were placed in a grow tent (2.7mx1.2mx2m Vivosun, Ontario, CA) at 21̊C, 70 RH with a photoperiod of 16:8 L:D for a period of three weeks. Following the start of D. suzukii emergence, all treatments were checked every other day, and D. suzukii were collected via aspiration every two days, frozen, sexed, and counted. The experiment concluded when all H. illucens larvae had pupated. Hermetia illucens pupae were then removed from all arenas and held to assess survival.
Experiment 2.3 Results:
Experiment 2.3.1: Treatments were significantly different (X2 = 22.8, p = 0.0018, df = 7; data not shown), with D. suzukii emerging only from the control treatment which did not contain H. illucens.
Experiment 2.3.2: There were significant differences between treatments (F2,12 = 45.14, p = < 0.0001). No D. suzukii adults emerged in treatments where H. illucens larvae were infested simultaneously with D. suzukii. When H. illucens were added day 7, 4 times more D. suzukii (39.4 ± 11.3) emerged than when H. illucens were added day 3 (9.8 ± 2.0). In treatments where H. illucens were added on day 3, D. suzukii were observed to pupate on the lids of arenas. Of the total 29 D. suzukii pupae collected from lids across all treatments, 5 survived to adulthood.
Experiment 2.3.3: In contrast with previous experiments, H. illucens infestation did not significantly reduce D. suzukii emergence regardless of volume of pomace in this experiment. More D. suzukii emerged as pomace volume increased (F2,17=6.29, p=0.009). Treatments with 250 ml pomace had an average of 222 ± 42.5 adult D. suzukii emerge per replicate, 500 ml had an average of 335 ± 42.5 D. suzukii, and 750ml had an average of 435 ± 42.5 D. suzukii per replicate. The lowest volume of pomace (250ml) had significantly less D. suzukii than the highest volume of pomace (750ml).
Experiment 2.3 Discussion: Adding black soldier fly larvae to apple pomace can reduce infestation by D. suzukii. However, the effectiveness of this method at controlling D. suzukii numbers depends on the timing of black soldier fly addition relative to D. suzukii infestation. When D. suzukii adults were added to infest substrate containing H. illucens larvae, D. suzukii offspring only survived to adulthood in control treatments where no H. illucens larvae were present. When D. suzukii were allowed to infest apple pomace for different time periods before H. illucens larvae addition, D. suzukii only emerged in treatments where H. illucens larvae were added following D. suzukii infestation.
Experiment 3: Due to limited labor following the departure of PI Grieshop from MSU, Experiment 3 was conducted at a single location during the 2023 growing season, the Northwest Michigan Horticultural Research Center. The following 5 combination treatments were applied piles of cherries consisting of 55L each with treatments replicated 4 times each and arranged in a randomized complete block design:
Treatment |
Spotted-wing drosophila (SWD) per 55 L |
Black soldier fly (BSF) larvae per 55 L |
Manure per 55 L |
1. Untreated control |
None |
None |
None |
2. SWD seeded control |
400 |
None |
None |
3. BSF + SWD |
400 |
1100 |
None |
4. Manure + SWD |
400 |
None |
11L |
5. Manure + BSF + SWD |
400 |
1100 |
11L |
Treatments were applied on 20 July 2023, and each pile of cherries was capped with an emergence tent. Insect activity was assessed via a yellow sticky trap placed inside the emergence tent and changed weekly. The experiment ran for a total of 8 weeks.
Experiment 3 Results: There were no significant differences in SWD within emergence cages between treatments. Only 1 black solider fly was recovered within emergence tents, so it is possible that they did not effectively establish under field conditions. A total 140 yellow soldier flies, a related species native to Michigan which were not added to our treatments, were recovered inside our emergence tents. These would have had to naturally colonize our experiment. Interestingly, yellow solider fly numbers were highest in our untreated control treatment (Treatment 1) and appeared roughly four times higher than Treatment 5 which contained a combination of chicken manure and black soldier flies.
Experiment 3 Discussion: Significant additional work is necessary to determine the best field methods for the use of black solider fly in post harvest pest control for fruit in Michigan.
The results of this experiment demonstrate that chicken manure fertilizers can reduce spotted-wing drosophila (SWD) emergence in the laboratory and under field conditions but do not appear to inhibit egg laying in whole fruit by flies. Black solider flies can effective limit SWD survival in fruit waste in the laboratory when introduced prior to or simultaneous with SWD egg laying, but translating this potential suppression to the field will require additional optimization.
Crushing fruit was just as effective over the course of an entire experiment in reducing SWD emergence in the field as adding chicken manure to fruit waste. The former may be a more cost effective and easily implemented strategy as compared to purchasing and incorporating fertilizer on unmarketable fruit.
The results of these experiments have been shared in 2021 and 2022 with growers attended programming at the Northwest Michigan Horticultural Research Center.
Education
We have developed a handouts and presented research at the 2021 and 2022 field days at the North West Michigan Horticultural Research Center presented at the 2021 Great Lakes Fruit and Vegetable Expo, and presented a poster and a talk at the 2022 Entomological Society of America Annual Meeting. No educational or outreach activities were held in 2023. A Master's student working on this project wrote and defended her thesis which can be downloaded from the MSU library website.
Project Activities
Educational & Outreach Activities
Participation Summary:
This presentation on project preliminary results was presented at the 2020 Great Lakes Fruit and Vegetable EXPO in Grand Rapids MI on December 9, 2020. PDF Slideshow.
A graduate student, Charlotte Shutter gave a seminar presentation at MSU that was open to the public and can be attended either in-person or online. This seminar served as her defense for her Master's thesis that researched the objectives of this grant.
Learning Outcomes
- Cultural sanitation techniques for reducing spotted wing Drosophila in post harvest tart cherries
Project Outcomes
Crushing fruit wastes to eliminate spotted-wing drosophila
Composting fruit waste with poultry manure to eliminate spotted-wing drosophila
Information Products
- Post Harvest SWD Management: Crushing and Composting to Manage SWD in Fruit Waste
- Soluble nitrogen fertilizers for spotted-wing Drosophila management on fruit and fruit waste
- Spotted-wing Drosophila Management on Post-harvest Fruit Waste
- Post Harvest SWD Management: Crushing and Composting to Manage SWD in Waste Fruit