Final report for LNE19-380
Project Information
Pennsylvania ranks second in the production of eggs in the US (USDA-NASS 2012) with 9,539-layer hen farms, including 586 farms that are organic/exempt. House flies are notorious for developing insecticide resistance. As a result, flies can’t be managed on most poultry farms using traditional insecticides. Biological control with biopesticides and parasitoids is a safe and environmentally friendly way to manage flies. Because of increasing insecticide resistance, additional tools to manage pest flies are needed on poultry facilities that are compliant with organic production and more environmentally sensitive. In addition, producers need to be educated on Integrated Pest Management options to comply with federal regulations. In this project, we evaluated new strains of the entomopathogenic fungi Beauveria bassiana for decreased kill times against house flies and safety if used along with parasitoid wasps that are often used in biological control. In addition, we developed an online course in poultry pest management for poultry producers. We successfully identified 5 new strains of B. bassiana. We found that they differed in virulence against house flies. We also found that Spalangia endius, a common parasitoid wasp, was more resistant to B. bassiana than two other commonly used species. This may help guide producers in parasitoid selection if B. bassiana is used for fly control. A 10-hour course in poultry fly management was produced through Penn State Extension. This course was video-based and also included Pennsylvania Pesticide Applicator credits. The course was developed so that each sub-section had embedded check your learning quizzes. Each section had a final quiz that was required before moving to the next section and a minimum passing grade. Participants were required to submit an IPM plan for review based on their facility as well. We had 38 producers take the course in poultry pest management and develop IPM plans for fly management. After this course, 41.7% of producers reported a reduction in chemical control use, and confidence in fly management increased by 33%.
Forty poultry producers, representing ~640,000 birds will adopt an IPM plan for fly control. Twenty will document decreases in fly numbers and 20 will save $200 by reducing chemical pesticide application for summer fly control.
Pennsylvania ranks second in the production of eggs in the US (USDA-NASS 2012) with 9,539-layer hen farms, including 586 farms that are organic/exempt. Despite its major role in the layer industry, it has been over 20 years since poultry arthropod control research has been conducted in this state. High populations of fly pests of poultry facilities present biosecurity risks of national concern. Muscoid flies are competent vectors of >100 pathogens including Salmonella, E. coli. (Mian et al. 2002), Coronavirus, New Castle disease (Malik et al. 2007) and potentially avian influenza virus (Wanaratana et al. 2013, Habibi et al. 2018). Of the more than 9 million foodborne illnesses in the United States, 22% are attributed to poultry (Painter et al. 2013). To offset these pathogen risks, the FDA egg safety rule requires the management and monitoring of flies as part of food safety regulations.
House flies are notorious for developing insecticide resistance. As a result, flies can’t be managed on most poultry farms using the traditional insecticides including organophosphates, carbamates, pyrethroids, diflubenzuron, and cyromazine (Boxler and Campbell 1983, Scott et al. 1999, Plapp 1990, Kaufman et al. 2001, Butler et al. 2007, Kozaki et al. 2009, Memmi 2010, see literature review for more detail). It has been estimated that the poultry industry spends about $22.5 million/annually on insecticides for fly control or $0.07/bird, exclusive of labor costs and other fly management efforts (FDA 2009). Losses due to house flies alone exceed $375 million/year (Geden and Hogsette, 2001). The country’s poultry producers face a pest control crisis with few options to protect animal welfare and human health.
Biological control with biopesticides and parasitoids is a safe and environmentally friendly way to manage flies. Fungi have been shown to have good efficacy, the only commercial mycoinsecticide available for poultry is “balEnceTM.” This product has produced disappointing results in both lab and field trials (Kaufman et al. 2005; Machtinger et al. 2016). A better fungal product is needed that will be as effective as conventional insecticides, and education is required on the use of biological control for fly control.
Poultry producers were surveyed in 2017 and 2018 via distribution through the membership of PennAg, integrators, and social media and responses were collected by mail and surveymonkey.com for a total of 215 responses. The survey was opportunistic and reached out to the poultry community, so a total response rate is not known but represented approximately 2,167,691 birds based on range responses.
Flies were listed by 77.6% (n=167) producers as a major issue. Over a quarter of respondents currently use biological control (26.0%, n=57), validating the need to test products for non-target arthropod safety, and 17.2% have at least tried biopesticides. Many respondents supported the development of new biopesticides (69.7% n=150); 22.7% (n=49) responded with “maybe,” depending on cost. Cumulatively, 75.3% of survey takers would use a new biopesticide. However, 14.8% stated that they maybe would use a new biopesticide but would require more education on safety and use to do so. Most respondents (71.2%; n=153), were willing to take a short course in poultry pest management if it was online. Many had time commitment concerns for in-person education.
To address this missed opportunity, the research proposed sought to develop a better biopesticide for fly control efficacy and application. The education portion developed a short course in poultry fly pest management using an IPM framework and focused on reducing insecticide applications by educating producers on available biological control options and effective use strategies.
Cooperators
- (Researcher)
- (Educator)
Research
A virulent isolate of Beauveria bassiana can be developed from poultry-specific fungi isolated from house flies in Pennsylvania that will reduce fly kill times to <3 days. This isolate can be formulated as part of a bait and developed in an attract-and-kill/autodissemination device that will protect the fungi from environmental effects and will distribute the fungi in the environment, reducing cost and application time for the producer, while reducing pest fly numbers.
Objective 1: Identify new strains of B. bassiana collected from poultry facilities.
Objective 2: Screen isolates for high virulence against house flies and select for greater virulence in new isolates.
Objective 3: Test high-virulence strains for compatibility with beneficial insects
Objective 4: To develop a novel auto-dissemination method microbial control of house flies.
Objective 1: Identify new strains of B. bassiana collected from poultry facilities.
Site Description
Flies were collected from seven poultry facilities in 2018 and five facilities in 2019. Four poultry layer facilities were in central Pennsylvania; two cage-free facilities in Loganton (site 1; 41° 1'20.69"N 77°18'27.22"W and site 2; 41° 0'18.51"N 77°21'50.64"W), one cage-free facility in Williamsport (site 3; 41° 8'40.61"N 77° 7'41.97"W), and the Penn State Poultry Education and Research Center in State College (site 4, 40°48'52.6"N 77°52'14.4"W). In southeastern Pennsylvania, flies were collected from caged-layer facilities in Elizabethtown (site 5; 40°05'26.4"N 76°35'38.8"W), Marietta (site 6; 40°04'25.8"N 76°37'27.7"W), and Manheim (site 7; 40°10'12.3"N 76°21'34.7"W). In the summer of 2019, sites 1, 2 and 5 did not participate in the study, but two new caged-layer facilities, one near Landisburg (site 8; 40°17'25.9"N 77°17'55.5"W) and the other in Millersburg (site 9; 40°34'03.3"N 76°51'21.0"W), were added. Each poultry house except for sites 4 and 6 had traditional pit manure collection, with air circulation provided by fans placed along the pits for the length of the building. Site 6 had a conveyor belt which deposited the manure into sheds that were open on one side and had a wall of fans on the other. Site 4 had a similar system, but on a much smaller scale, with the conveyor belt just depositing the manure in an open area behind the house. Sites 1, 2, 3, 8 and 9 had one house with between 7,500 and 10,000 Hy-Line brown birds. Sites 5 and 6 had around 70,000 birds per house, all of which were White Leghorns. Site 7 had eleven houses of three types: Four houses of around 425,000 caged White leghorns, four houses of 195,000 caged Brown Leghorns, and three houses of 63,000 cage-free Brown Leghorns. Site 4 had less than a thousand birds per house, with mostly White Leghorns and some “show” variety birds housed in the same area.
Muscid Fly Collection
Live muscid flies were collected from June to August. Collections were made from manure pit areas weekly in 2018 and every two weeks in 2019 using a sweep net. Flies were taken from various areas along the length of the house until approximately 100 had been collected as determined visually. Flies were placed in 32.5 x 32.5 cm Bug Dorms (Megaview Science, Taiwan) and held in an environmental chamber set to 25°C. Each cage was provided with 16 oz of a 10% sucrose solution and 8 oz of dried egg powder ad libitum.
Bug dorms were checked every 3 days for fly mortality for a total of 14 days. Dead flies were identified to species and surface-sterilized by submerging in 70% ETOH (Holderman et al. 2017). Non-muscid flies were discarded. Flies were then placed on a 100 x 5mm Petri dish with a 90 mm circular filter paper wetted with 2 ml of deionized water to provide suitable humidity to encourage fungal growth on the fly cadaver. The edge of the Petri dish was wrapped in parafilm and then placed in the environmental chamber for 14 days. Fly cadavers with fungal growth were transferred to a 30-mm Petri dish lined with a new piece of filter paper and placed in a desiccator to dry for at last 7 days to dry out the spores and suspend the life cycle of the fungus and allow the samples to be stored and shipped.
Beauveria bassiana identification
Flies with fungal growth were dissected and samples of fungus placed in 100 μL of a 0.01% Capsil® (Aquatrols, Paulsboro, NJ) solution and pipetted onto Sabouraud dextrose agar plates with yeast (SDAY) containing gentamicin sulfate and chloramphenicol to limit the growth of other microbes (Goettel and Inglis 1997). To prepare the plates, 15 g of Sabourad dextrose broth, 5g yeast, 7.5 g of agar were added to 500 mL of de-ionized water and stirred on a stir plate until incorporated. The SDAY media was autoclaved and left to cool until it was 60℃. Once this temperature was reached 1.25 mL of gentamicin and 1 mL of chloramphenicol were added. Twenty mL of this solution were pipetted into 100 mm sterile Petri dishes in a biological fume hood, where they were left to solidify. An L-spreader was used to spread the solution into an even layer. Plates were held at 25°C in an environmental chamber for 7 to 9 days to sporulate.
The mycelium was then harvested and sequenced using the B-Locus region (Rehner and Buckley 2003) using a SNX forward primer. The PCR reactions consisted of 25 cycles of 95 °C denaturation for 30 s, then 60 °C annealing for 1 min, followed by 72 °C for 2 min, and finally by a 72 °C incubation for 30 min. Five isolates from field collection were identified as having around 900 base pairs overlapping B. bassiana species at 99 % when compared in BLAST.
Objective 2: Screen isolates for high virulence against house flies and select for greater virulence in new isolates.
Sources, propagation and preparation of B. bassiana for testing
From previous field collections, five strains of B. bassiana fungus were isolated and named PSU 1,2,3,4, and 5. Preliminary tests showed that PSU 1, 4, and 5 would be the most appropriate to use in this study, given their more consistent viability. We compared these three strains as well as strains GHA, a strain isolated from a chrysomelid beetle that is registered for use and available in three commercial products, and L90, a strain isolated from house flies at a dairy farm in New York, as positive controls. The negative control was a sterile 0.1 % Tween 80 (polysorbate 80, polyoxyethylene sorbitan monooleate) (Sigma-Aldrich, Steinheim, Germany) solution. Each strain of fungus was cultured over 9 days in advance on Sabaourad Dextrose Agar Yeast (SDAY) plates (15 g of Sabourad dextrose broth, 5 g yeast, 7.5 g of agar to 500 ml of de-ionized water) to allow for full sporulation. The exception to this was GHA, which was obtained as pure spore powder (Lot number 16-06-2A, LAM International, Montana, USA). Strains were “wet harvested” which involved pipetting 10 mL of 0.1% tween onto the plate and then gently scraping the fungal bodies and liquid into an Erlenmeyer flask. The flask was then covered with parafilm and vortexed for two one-minute increments, with an interval in between to gently swirl the glass and redistribute the spore powder back into the Tween 80 solution. The liquid was poured into a glass media bottle through a glass funnel lined with a thin sheet of glass wool that had been moistened with de-ionized water. The spores still caught in the glass wool were gently rinsed with 0.1% Tween 80 to push them through to the solution in the bottle.
Each spore suspension was then counted using a hemocytometer and tested for viability. Spores were counted in the method described by Moore 2018. For the viability test, 10 μL of spore solution was placed on an SDY plate. The plate was then left in an environmental chamber for 24 h at 25 °C and then the spores were checked under a microscope for germination. Any spores that produced a germ tube that was at least half as long as the diameter of the spore were considered viable and a percentage was calculated. The results of these tests were used to determine the dilution needed to achieve a suspension containing a 109 concentration of viable spores in 0.1 % Tween 80 solution. Once the correct concentration was achieved, 1.5 mL would then be pipetted onto a piece of 100 mm filter paper (Whatman grade 4, Sigma-Aldrich, Steinheim, Germany), and left to dry for 30 minutes in a corresponding petri dish (except for the first trial, which was left to dry for approximately half an hour rather than exactly). Even coverage of the treatment onto the filter paper was achieved by affixing the paper to a pottery wheel and then pipetting the treatment onto it from the center to the outside as it spun, forming a spiral pattern.
Sources and rearing methods for flies
For each replicate, ten females between 3-to-5 days old were used. A colony established from the previous collection season was used. House flies were kept in bug dorms and fed on a diet of sugar and milk powder, with water provided. The colony was continued by collecting eggs from oviposition cups, a cup containing a piece of black fabric wrapped around a substrate, like manure. These eggs were then rinsed into a large container full of rearing media, a mixture of two parts wheat bran to one-part calf manna (MannaPro, Chesterfield, Missouri) and one-part water. After developing for one week, the resulting pupae were separated from the media by placing the entire mix into water and straining out the pupae that naturally float to the top. These pupae were dried and then placed in a new cage.
Three to five days after eclosion was observed in the new cage, the flies were removed with a modified handheld vacuum with a removable compartment inside. This compartment was removed with the recovered flies and placed in a chamber filled with CO2 until the house flies were knocked down (no more than two min). They were then placed in a container, placed within a larger container filled with ice, to slow them down without overly chilling them. They were separated into males and females with the females being placed into 2.5 mL cups with lids, with ten in each. CO2 was applied as needed during the sorting process.
Bioassay methods
The house fly females from the previous section were knocked down again within the cups they were stored in and then 10 each introduced to the treatment plate. The house flies were made to remain in contact with the treatment during the testing period by coating the entire area of the lid in Fluon® (Insect-A-Slip™, Bioquip products, Rancho Dominguez, California) and allowing it to dry prior to the trial. The lid to the 150 mm Petri dish containing the filter paper and water would be placed over the setup to maintain humidity. After the testing period was over, the flies were removed with the use of CO2 through a small hole that had been drilled into the lid of each Petri dish, allowing CO2 to enter but preventing the flies from escaping. Flies were then placed in a new container for observation. The new container was a 473 mL plastic container (Choice, Lancaster, Pennsylvania, U.S.) with a muslin cloth lid placed over the opening and held tight with the container's accompanying lid, which had the middle cut out to about 2 cm from the edge. A 2.5 mL cup of both milk powder and a 10 % sugar water (sucrose) was provided inside. The flies were then observed daily for 14 days for mortality. Any cadavers were removed daily with soft forceps through a cut in the cloth lid and placed on a piece of 35 mm filter paper (Whatman grade 1, Sigma-Aldrich, Steinheim, Germany) in an accompanying Petri dish and dried for 24-48 hrs. The cut in the lid was small enough (2.5cm) that by putting a hand over the opening and feeding the forceps between two fingers, flies could be removed without additional anesthetization while preventing any accidental escapes. A piece of tape was smoothed over the cut when not in use, placed carefully so that the sticky side was not exposed to the flies. After the house flies were placed in the 35 mm Petri dishes for 24 hrs, 0.2 ml of sterile de-ionized water was added to the 35 mm filter paper. The cadavers were observed for another week to confirm sporulation and therefore death from B. bassiana infection.
Objective 3: Test high-virulence strains for compatibility with beneficial insects (** Concurrent with Objective 2, so only beneficial insect methods are reported here as the remaining methods are the same as above).
Sources and rearing methods for parasitoids
For each replicate, ten females between 3-to-5 days old were used. A colony established from the previous collection season was used. The species of parasitoids used were Spalangia cameroni, Spalangia endius, and Muscidifurax raptor. These were taken from established colonies available at Penn State and were originally from Minnesota (S. cameroni), California (S. endius), and Florida (M. raptor). Trichomalopsis spp. were not tested in this bioassay even though they were collected frequently in Pennsylvania due to the lack of an established colony. Muscidifurax raptor was tested because of previous reports in other states that this species may be common in poultry facilities, and it is often sold as a biological control agent.
To separate males from females and get the correct age range, the “feeding” style of the parasitoids was taken advantage of. Parasitoids were kept in bug dorms (Bioquip products, Rancho Dominguez, California) and given fresh fly pupae twice a week. Each time a new cup of pupae was added, the old one would be taken out and covered, and left for either three weeks (for Spalangia) or two weeks (for Muscidifurax). At the end of this time period the cups would be placed back into the cages to release the next generation of parasitoids that had been deposited in the pupae the last time they were in the cage.
To get the correct age range, a sample from the pupae containing the next generation was separated and placed in separate bug dorms. Because males emerge first, for the first three days, wasps were removed from these isolated cages by covering the pupal cup in a screened lid and shaking it back out into the original cage. The pupae were left in cages for 3-5 d to get the correct age range for females. Parasitoids were removed from the cage through an aspirator. The females were identified (taking care to not mix species) on a cold plate through a microscope. Each species was then placed in 2.5 ml cups with lids, divided into groups of ten each to be transferred for the bioassay.
Objective 4: To develop a novel auto-dissemination method microbial control of house flies.
House flies have been shown to be susceptible to sugar baits formulated with fungal conidia (Machtinger et al. 2016). Using fungal baits could provide an alternative to chemical control, slow insecticide resistance, and reduce control costs. The use of Beauveria-laced baits would minimize the risks to beneficial arthropods as well (Vega et al. 1995). However, sugar baits provide a nutrition source for fly pests. The use of artificial sweeteners in B. bassiana baits has recently been demonstrated to provide excellent fly control under lab conditions without giving flies a life-extending dose of sugar (Burgess et al. 2018). Therefore, Beauveria baits with isolates selected for fast kill times should be developed with non-nutritive sugars as part of a fly control program for flies in poultry facilities.
Materials and methods
Bait preparation
A non-nutritive sugar (erythritol) was evaluated laced with B. bassiana conidia. The sweetener was 100% pure erythritol (Now Foods, Bloomingdale, IL) and was tested with 100% Sucrose (Crystal sugar, United Sugars Corp., Minneapolis, MN). One mg of spores were tested for efficacy in concert with fungal isolates at 4 ×108 and 10 mg at ca. 4 × 109 conidia. Spore viability was tested during each experiment. Xylitol was proposed to be tested, but because of avian toxicity concerns, this was excluded from the assays.
Adult house fly consumption of sweeteners with B. bassiana isolates
Ten male and female house flies were placed in 17.5- × 17.5- × 17.5-cm test cages (Bugdorm; Megaview Co., Taichung, Taiwan) with water ad libitum. Five grams of each sugar combined with B. bassiana, and sugars without B. bassiana were weighed and tested for consumption over a 72-hr period. Baits were weighed daily. Flies were held at 27 °C with sucrose, dried milk and egg powder, and water ad libitum. Dead flies were removed every 2-3 days and processed for sporulation.
Adult fly mortality after exposure to B. bassiana baits
Groups of ten male and female flies were exposed to each sugar combined with B. bassiana for 12 h, 24 h, and 48 h to determine mortality after short- and long-term exposure. Choice assays (all sugars) and no-choice assays were conducted. After each exposure time, flies were held at 27 °C with sucrose, dried milk and egg powder, and water ad libitum. Dead flies were removed every 2-3 days and processed for sporulation.
Objective 1: Identify new strains of B. bassiana collected from poultry facilities.
During the first collection season (2018), 5,366 filth flies were collected. Of these, 4,009 were house flies, 1,192 were black dump flies (Hydrotea aenescens, Diptera: Muscidae,Wiedemann 1830), and 165 were little house flies (Fannia canicularis, Diptera: Muscidae, Linneaus 1761). The remainder were other species that were not identified (Table 1). From these collections, 662 fungal candidates were chosen for further testing and five isolates were confirmed to be B. bassiana. This gave an overall infection rate of 0.09%. Of these five isolates, four were house flies from a June 8 collection in Marietta, PA (0.01 % infection in house flies) and one was a black dump fly from a collection in Manheim, PA (0.08% infection in black dump flies) on the same day. Most specimens that displayed Beauveria-like mycoses and all of the confirmed isolates were from collection sites in Southeastern Pennsylvania (Table 2).
Samples from 2019 were held in the same environmental chamber as the previous year. Unfortunately, a separate part of this overall project was added shortly after the season started, which involved rearing large numbers of house fly immatures in this chamber. There is a good amount of ammonia produced by the combination of house fly larvae and the media they are reared in and placing them in a warm enclosed space caused the ammonia levels to become very high. I suspect that these high ammonia levels prevented any fungus from sporulating, as no cadavers were salvageable, and no data was recorded for the summer of 2019.
Table 1: Total number of Musca domestica and Hydrotea aenescens caught at layer facilities in Central and Southeastern Pennsylvania in the summer of 2018. June had the highest number of collections for both groups, with August having the least. This may be partly because one of the locations was no longer accessible due to a new flock.
June |
July |
August |
||||
Site |
Musca domestica |
Hydrotea aenescens |
Musca domestica |
Hydrotea aenescens |
Musca domestica |
Hydrotea aenescens |
1 |
89 |
555 |
29 |
99 |
84 |
59 |
2 |
310 |
3 |
196 |
5 |
- |
- |
3 |
546 |
8 |
347 |
0 |
16 |
2 |
4 |
210 |
0 |
109 |
0 |
130 |
0 |
5 |
242 |
0 |
391 |
0 |
364 |
0 |
6 |
210 |
1 |
261 |
3 |
247 |
0 |
7 |
22 |
220 |
55 |
115 |
151 |
122 |
Table 2: Total number of flies from all species caught at layer facilities in Central and Southeastern Pennsylvania in the summer of 2018, with accompanying infection rates by Beauveria bassiana. Of the 5,366 samples recovered, only five were confirmed to be infected with B. bassiana1. Of those five, all of them were caught on the same day, June 8th, and four were even from the same location.
June |
July |
August |
||||
Site |
Flies (N) |
Flies Infected (N/%) |
Flies (N) |
Flies Infected (N/%) |
Flies (N) |
Flies Infected (N/%) |
1 |
653 |
0 |
261 |
0 |
143 |
0 |
2 |
313 |
0 |
210 |
0 |
- |
- |
3 |
562 |
0 |
355 |
0 |
16 |
0 |
4 |
210 |
0 |
109 |
0 |
130 |
0 |
5 |
242 |
0 |
391 |
0 |
364 |
0 |
6 |
211 |
4/1.8% |
264 |
0 |
247 |
0 |
7 |
242 |
1/0.4% |
170 |
0 |
273 |
0 |
[1] House flies were exposed to the isolates collected, and any that demonstrated the ability to cause mortality in house flies were selected for further PCR testing. This is how the isolates were confirmed to be B. bassiana.
Discussion
Biological control using entomopathogenic fungi such as B. bassiana could become a valuable part of pest fly IPM. This potential could be further improved by using strains that are isolated from the target pest itself (Maurer et al. 1997). The discovery of naturally occurring strains of B. bassiana on house flies at layer poultry facilities in Pennsylvania is a promising step for developing more effective biological control strategies using this pathogen.
While B. bassiana has been reported to occur in house fly populations (Steinkraus et al. 1990) rates of natural infection appear to be very low (Siri et al. 2005). Only house flies that displayed outward signs of infection, visible fungal growth on the cadaver, were sent for further screening so it is likely that some cases of B. bassiana infection were missed due to lack of sporulation. The infection rate in the current study, 0.09%, was in line with other studies (Steinkraus et al. 1990, Steenberg et al. 2001, Skovgård and Steenberg 2002, Siri et al. 2005), but those studies have a wide range, from as low as 0.06%,(Steinkraus et al. 1990) or as high as 1.45% (Siri et al. 2005). The percentage was calculated from the 5 strains confirmed to be able to kill flies in preliminary testing and then confirmed by PCR. The reason for this typically low infection rate may be certain environmental factors or differences between host populations. Given the low natural infection rates demonstrated in the literature, paired with the difficulties associated with applying the fungi to poultry facilities, it is encouraging to have recovered as much as we did to demonstrate that natural strains can and do occur in these environments.
Considering the low infection rates among house flies presented here and in accompanying literature, questions arise as to the reservoir for the pathogen. With such low numbers in house flies, it may be possible that they may not wholly be the source for the spread of B. bassiana amongst themselves. If the case is that the infection is not being brought in by house flies from the outside, and the rest of the arthropods present in the manure do not have a great range, it is possible that the fungus is continuing its life cycle in the poultry house in another way. In the future it may be interesting to look at beetles in poultry houses for the presence of B. bassiana, with both Carcinops pumilio and Alphitobius diaperinus as possible vectors. Since B. bassiana can be produced in large amounts commercially on substances like corn or other grains (Karanja et al. 2004, Latifian et al. 2014), the viability of spores grown on chicken feed (pre- and post-digestion) should be researched.
Some of the difficulties associated with B. bassiana growth in poultry houses are related to the high ammonia levels produced by the accumulated manure (Acharya, Rajotte, et al. 2015b). Ultraviolet light can also kill B. bassiana conidia (Daoust and Pereirn 1986, Inglis et al. 1995), which may be an issue in smaller, open-sided poultry houses. Perhaps the main deterrent for B. bassiana efficiency in poultry houses is the specific level of humidity required for it to function most efficiently (Walstad et al. 1970, Gillespie and Crawford 1986). Traditionally, manure pits are kept as dry as possible to prevent house fly development. The commercial product balEnce™ recommends applying large amounts of aqueous spray as part of its application. Not only is this a much higher demand on time than a traditional pesticide application, but the increased moisture in the manure leads to higher fly populations. In addition to the issues it has remaining viable in the environment it is applied to, balEnce™ takes 6 days to kill fly pests, giving the flies enough time to breed and start another generation. This suggests that the strain used in balEnce™ is not virulent enough to be used in this context. Future research will have to focus on delivering the fungus in a way that shelters it from the deleterious effects of the manure while also allowing for normal business operations, and on finding an isolate with increased virulence.
Looking at the recovery rate from individual farms, there is an interesting trend that may indicate the degree to which house flies are responsible for transmitting the fungus between each other. As B. bassiana is associated with soil (Hajek, 1999; Keller & Zimmerman,1989; Meyling & Eilenberg, 2007) and can be further spread through mechanisms like wind and rain, more open systems might be expected to have a higher incidence of B. bassiana infections. However, the majority of B. bassiana isolates recovered were from a caged-layer facility with deep manure pits that had solid walls except for the exhaust fans embedded in them (site 6). Conversely, at three of the other collection sites (1, 2, and 3) the ends and sometimes the sides of the manure pit walls had screens in place, which allowed air and rainwater into the manure pit, but none of the confirmed B. bassiana samples came from these locations. Perhaps the increase in ultraviolet light from natural sunlight, or the use of lime at these facilities prevented B. bassiana occurrence. Additional research is needed to see how the different environmental conditions and designs of layer facilities could affect entomopathogenic fungi. Another factor that may explain this is that these three locations had noticeably lower house fly populations. This would support the idea that the house flies are mainly picking up these infections through contact with other house flies rather than contact with the pathogen in the environment (García-Munguía et al. 2015)
Fungi are diverse in the ecosystem and many species other than the target B. bassiana or M. anisopliae may be recovered. Site 5 had the second highest number of flies captured and of cadavers showing fungal growth yet did not yield any confirmed B. bassiana cadavers. This is especially interesting as Site 6 which was the source of nearly all B. bassiana strains recovered was less than 4 km from site 5. A possible explanation for the lack of B. bassiana could be the high prevalence of Entomophthora muscae at this location. Flies observed on location at site 5 and those brought back to the lab during the late summer months exhibited behavioral symptoms and the mass mortality associated with E. muscae infection (Watson et al. 1993). It’s possible that the presence of this fungus affects the house fly behavior in a way that makes them less likely to encounter B. bassiana, or that infection with E. muscae prevents further infection with B. bassiana. Future studies could look into how co-infection with this common entomopathogenic fungus affects the lethality of B. bassiana.
While the current B. bassiana product labeled for use on poultry has had disappointing returns (Weeks et al. 2017), other strains such as GHA have proven more efficient at killing house flies in comparison (Machtinger et al. 2016; Weeks et al. 2017). At high enough concentrations, GHA has been reported to have a mean survival time of 5-6 d (Anderson et al. 2011; Acharya, Rajotte, et al. 2015a; Weeks et al. 2017) which is faster than the 6-8 d survival range of HF23. If a more virulent strain can be developed it may lead to more effective fly control on poultry facilities using entomopathogenic fungi. These strains could also be tested for other characteristics such as high spore production, performance at higher temperatures, ammonia tolerance, and other factors which would make them well suited for development as a biological control tool. Efficacy of the recovered B. bassiana strains from this project will be evaluated in future studies
Objective 2: Screen isolates for high virulence against house flies and select for greater virulence in new isolates and Objective 3: Test high-virulence strains for compatibility with beneficial insects (**Because objectives 2 and 3 were screened together, results are reported together herein).
Results
Due to differences in mycoses between strains, and between species, overall mortality was used as a metric instead of visible mycoses on cadavers. All B. bassiana treated house flies had a significantly lower mean survival time than the negative control, which had a mean survival time of 13.05 days (Table 3).
Table 3: Mean survival time for house flies over a 14-day period after exposure to treatments. All strains were significantly different than the control, with GHA being the least efficient and L90 being the most efficient in killing house flies.
Treatment |
Mean survival time (14 days)* |
Std. Error |
Lower Bound |
Upper Bound |
Control |
13.05 a |
0.18 |
12.69 |
13.41 |
GHA |
8.28 b |
0.28 |
7.73 |
8.84 |
PSU1 |
7.76 b |
0.23 |
7.31 |
8.21 |
PSU4 |
7.04 b,c |
0.24 |
6.57 |
7.50 |
PSU5 |
6.22 c,d |
0.21 |
5.82 |
6.62 |
L90 |
5.67 d |
0.20 |
5.28 |
6.06 |
*Mean survival time with the same letters in did not differ significantly at the α of p <0.001. Significant value in pairwise comparison between PSU4 and PSU5 is p= 0. 0013, close to significant.
The next highest mean survival time was GHA at 8.28 days, and the lowest was L90 at 5.67 days. The survival curve for all strains is shown in Figure 1. There were three parameters compared with the log-rank test. The first of these was the strains vs the control, to confirm that an effect was taking place. This measure was significant (χ2=428.67; DF=5; P=<0.001). The next grouping that was compared was all the treatment strains against each other, to see if there was a difference in the efficacy of strains isolated from muscoid flies to GHA, which was not isolated from a muscoid fly. These results were also significant (χ2=76.85; DF=4; P=<0.001). The last comparison was between all the fungi isolated from house flies, which also demonstrated a significant difference (χ2=47, DF=3, P=<0.001). Looking at the efficacy overall, the ranking was L90> PSU5>PSU4>PSU1>GHA.
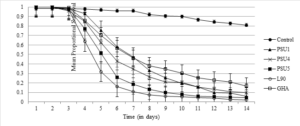
Among the 3 parasitoid species, all strains of B. bassiana had the most effect on S. cameroni (χ2=55.93 ; DF =5 P=<0.001 )followed by M. raptor( χ2=38.39 ; DF =5, P=<0.001 ), and S. endius (χ2=11.95 ; DF =5 P=<0.001 ) (Table 4).
Table 4: Comparisons of the significant values of the test isolates on house flies and three species of parasitoid. Comparisons of different groups were pooled over strata with a log-rank test. Measurements were taken until a significant value was no longer reached, suggesting any difference between the strains compared. There was a significant difference in house flies between the treatments and the control, between the treatments, and between the treatments isolated specifically from house flies. For S. cameroni and M. raptor, there were only significant differences between the control and the treatments. For S. endius, there was no difference.
Comparisons |
Χ2, p-value |
|||
M. domestica |
S. cameroni |
S. endius |
M. raptor |
|
Treatment vs. control |
428.67, <0.0001 |
55.393, <0.0001 |
16.46, 0.006 |
38.39, <0.0001 |
GHA vs House fly isolates
|
76.85, <0.0001 |
2.15, 0.708 |
- |
4.62, 0.327 |
House fly isolate vs. House fly isolate |
47.00, <0.0001 |
- |
- |
- |
PSU1 has the lowest mean survival time among all parasitoid species (Tables 5,6,7). However, highest mean survival time was variable between the three species ranging from PSU5 for S. cameroni, L90 for S. endius, and PSU 4 for M. raptor. When compared again with the log rank test to compare the differences between just the isolate treatments, no significant difference was found for any of the species (S. cameroni , χ2 =2.15; DF =4; P=<0.001 ) (M. raptor , χ2 =4.63 ; DF =4; P=<0.001 ) meaning that there was little difference in effect between B. bassiana isolates in parasitoids. In considering these results, data taken from over 14d may not be an appropriate measure for these parasitoids as the end of their natural life cycle falls within the treatment time range.
Table 5: Mean survival time for Spalangia cameroni over a 14-day period after exposure to treatments. Pairs of means with the same letters did not differ significantly at the α of p <0.001. All strains were significantly different than the control, with PSU1 being the least efficient and PSU5 being the most efficient in killing S. cameroni, but the difference between these strains was not significant.
Treatment |
Mean survival time (14 days) |
Std. Error |
Lower Bound |
Upper Bound |
Control |
8.57a |
0.32 |
7.94 |
9.21 |
GHA |
6.09b |
0.25 |
5.62 |
6.57 |
PSU1 |
5.63b |
0.23 |
5.18 |
6.08 |
PSU4 |
5.88b |
0.27 |
5.34 |
6.41 |
PSU5 |
6.13b |
0.29 |
5.56 |
6.70 |
L90 |
5.73b |
0.31 |
5.13 |
6.33 |
Table 6: Mean survival time for Spalangia endius over a 14-day period after exposure to treatments. Pairs of means with the same letters did not differ significantly at the α of p <0.001. The only strain that was significantly different from the control at an α of p<0.001, was PSU1, with p= 0.0075 in a pairwise test against the control. The rest of the strains did not differ significantly from each other.
Treatment |
Mean survival time (14 days) |
Std. Error |
Lower Bound |
Upper Bound |
Control |
7.479a |
0.299 |
6.893 |
8.066 |
GHA |
6.403a b |
0.279 |
5.857 |
6.949 |
PSU4 |
6.151a b |
0.269 |
5.624 |
6.678 |
PSU5 |
6.456 a b |
0.311 |
5.847 |
7.065 |
L90 |
6.911a b |
0.339 |
6.247 |
7.575 |
PSU1 |
6.036 b |
0.238 |
5.570 |
6.503 |
Table 7: Mean survival time for Muscidifurax raptor over a 14-day period after exposure to treatments. Pairs of means with the same letters did not differ significantly at the α of p <0.001. All isolates were significantly different from the control, but not from each other.
Treatment |
Mean survival time (14 days) |
Std. Error |
Lower Bound |
Upper Bound |
Control |
7.872a |
0.334 |
7.217 |
8.528 |
GHA |
5.355b |
0.329 |
4.710 |
6.001 |
L90 |
5.476b |
0.324 |
4.842 |
6.110 |
PSU4 |
5.706b |
0.318 |
5.083 |
6.330 |
PSU5 |
5.335b |
0.330 |
4.687 |
5.983 |
PSU1 |
5.198b |
0.261 |
4.687 |
5.710 |
Discussion
Of all the strains of B. bassiana tested, many were shown to have different traits that would be attractive for use as biological control and these traits varied between strains. For example, while PSU 1 had the second highest mean survival time, overall it killed more flies than PSU 4, a strain with a lower mean survival time. Kill rate of PSU 1 was comparable to the more efficient PSU5 and L90. All strains that were originally isolated from muscid flies performed better than GHA, a strain that was isolated from a Chrysomelid beetle. This is interesting, as GHA has been used for over a decade as a commercial application, and has been found to be effective for other insect orders (Ludwig and Oetting 2002, Liu and Bauer 2008). This supports the suggestion that certain strains of B. bassiana show a degree of host specificity (Fargues and Remaudiere 1977, Steinkraus et al. 1990, Viaud et al. 1996, Maurer et al. 1997, Vestergaard et al. 2003).
In house flies, the mortality rate for strains was lower than expected, especially for L90 (Geden et al. 1995, Watson et al. 1995, Weeks et al. 2017, Burgess et al. 2018b, Johnson et al. 2019) and GHA (Anderson et al. 2011) which has yielded high mortality rates in previous studies. The reason for this may have been the delivery method which the test used to expose the house flies to the spores. In theory, only the tarsi of the fly were in contact with the treatment the entire time, with the mouthparts occasionally coming into contact through feeding and grooming behavior. Previous studies suggest that many of the spores present would be removed through grooming or not be effective after germination due to an immune response (Butt et al. 1988, Gillespie et al. 2000). Other attachment points such as the eyes, abdomen, or the base of setae are more effective in initiating infection (Hasaballah et al. 2017). Other studies have exposed the entire surface of the insect to a fungal suspension to determine efficacy (Mwamburi et al. (2010), however, the methods herein were in line with what a fly would encounter in the field.
Another possible explanation for the lower mean mortality and longer survival times than reported in previous studies may have been due to the formulation used to apply the treatment. Both Weeks et al. 2017 and Anderson 2011 exposed their flies to filter papers in a similar design to this experiment, except that they used oil formulations instead of water and a non-ionic surfactant. Other studies involving house flies have also yielded higher mortality at a faster rate, as well as longer reports of viability when using an oil-based formulation (Acharya, Rajotte, et al. 2015a, 2015b, Acharya, Seliga, et al. 2015). Non-ionic surfactants, while often helpful in improving the stability of the spore suspension, may have detrimental effects on the fungus, while the introduction of oil may stimulate germination (Luz and Batagin 2005). In addition to this, the introduction of oil to a formulation may improve the spore’s adherence to the insect cuticle. Another important factor in B. bassiana virulence is the dose that the insect is exposed to (Watson et al. 1995, Anderson et al. 2011). All the experiments that yielded higher mean mortality and shorter survival times used a dose of 1x109 spores/ml, the same as this bioassay, or lower even. But if the oil formulations allow for more spores to remain in contact with the insect cuticle than just Tween80 and water, then the effective dose for these experiments is much higher.
In terms of parasitoids, the effective dose of these treatments may also explain why the isolates had less of an effect on them than on the house flies. Male house flies have been shown to be more resistant to B. bassiana exposure than females (Acharya, Rajotte, et al. 2015a). While this may be due to different immune responses between males and females, it may also relate to the fact that females are slightly larger than males. A larger body size means a larger surface area that can pick up more spores. Parasitoid wasps are many times smaller than house flies so they may be getting a reduced dose of spores. Other experiments have also demonstrated reduced effects on parasitoids when directly exposed to B. bassiana (Geden et al. 1995, Lecuona et al. 2007), with one of them only achieving higher mortality when exposing the parasitoids a second time, increasing the effective dose.
Efficacy differences among B. bassiana strains from previous research may have been a result of house fly susceptibility differences by population, perhaps related to immune response. The flies used in this study were caught in poultry houses the year prior, and therefore would better reflect the population that would be found in a field setting than a colony that has been lab reared for many years (Bryant and Meffert 1998). House flies do exhibit an immune response when infected with B. bassiana (Mishra et al. 2015). With possible repeated exposure in the field, these “wild” populations may be better equipped to handle a B. bassiana infection than a colony that has no history of exposure (Dubovskiy et al. 2013). This immune response could be carried in future generations, even without exposure (Trauer and Hilker 2013), which could explain the lower rates of infection from this study. Considering how insular the poultry layer facility ecosystem is, this effect would also have serious considerations for biological control. Certain strains may perform better in certain populations, and those populations could differ from region to region or farm to farm.
While results for the house flies showed considerable variation, the results for all three species of parasitoids were less clear cut. This ultimately may be a good indicator for the use of B. bassiana as part of an IPM program, as we chose these species because they are often incorporated into management programs. For S. cameroni and M. raptor, the difference between the control and the treatment groups was lower than the difference found in the flies, and S. endius had hardly any significant difference at all. Lecuona et al. 2007 also exposed S. endius to B. bassiana with very minimal effects for single dosing, so the interaction between B. bassiana and S. endius, in particular, may be an interesting area for future study. Overall, these lesser effects imply that both tactics could possibly be used together more effectively in an IPM program. This lesser effect is especially beneficial to M. raptor, the species that is more commonly released in poultry facilities (Kaufman et al. 2000). Muscidifurax raptor would be more likely to be exposed to the current commercial application technique of B. bassiana as it spends more time in the upper layers of the manure than the two Spalangia spp. (Geden 2002).
It was not shown that there are any further differences between strains in terms of their effects on parasitoids. Parasitoid mortality was measured over 14 d for all species. Given how different the two life histories of the groups are, further studies may be done with shorter observation ranges to measure fungal efficacy, to better reflect their shorter lifespans. The adult lifespan of both Spalangia genera tested is three weeks while Muscidifurax raptor's is only two, so a mortality measurement over 14 d may not be necessary and a smaller window of time may give better insights to the differences in effectiveness between strains for parasitoids if any exist.
While B. bassiana genotypes do display host specificity (Fargues and Remaudiere 1977, Maurer et al. 1997, Vestergaard et al. 2003), the strains isolated and found primarily in one host can be effective for a range of different species (Butt et al. 2009, Wraight et al. 2010). This suggests that perhaps instead of being well suited to the host, perhaps the specificity arises from the strain being well suited to the environment in which that host is normally found (Uma Devi et al. 2008). Future studies could replicate different conditions found in a poultry house, such as high ammonia levels and high temperatures, to see how they affect lethality in house flies. The increased temperature, in particular, would be an interesting variable to study. In the field house flies can exhibit a form of the fevering behavior seen in which results in lowering the virulence of the B. bassiana infection (Anderson et al. 2013a, Anderson et al. 2013b). Finding a strain that is particularly tolerant of high temperatures would be useful in developing a biological control tool.
Overall, the B. bassiana strains isolated from house flies killed greater numbers of flies than the negative control and GHA. In parasitoids, all strains had a more limited effect than was observed in the house flies, except for in S. endius, in which there was no effect. The susceptibility of these house flies to the treatments and the lack thereof in all parasitoid species is a good indicator of the usefulness of field-collected strains of B. bassiana and their use as a biological control tool. Given that the strains each demonstrated different traits in their infection of house flies, further research should be done to see the extent of each of these traits and if they could be useful for biological control (Gillespie and Claydon 1989, Hajek and St Leger 1994, Kershaw et al. 1999, Anderson et al. 2011).
Objective 4: To develop a novel auto-dissemination method microbial control of house flies.
Results
Sugar feeding trials with house flies were started in 2019. Erythritol and sucrose were used as sugars as xylitol is toxic to birds. Sugar is consumed at a higher rate than erythritol, but erythritol seems to have a lethal effect on flies.
A pattern emerged through the first trials where most treatments with erythritol and the B. bassiana isolates resulted in greater morality than the sucrose with B. bassiana isolates. This was not the case with PSU 5 and L90. Infectivity of house flies was over twice as high in the erythritol with B. bassiana over the sucrose with B. bassiana, however (Figure 2). This may suggest that there is some component of the erythritol that synergizes with the fungus for greater efficacy.
These trials were not completed as expected. The University required that our fly rearing room be moved on two separate occasions because of incompatibility with other building residents. To make these moves, we had to decrease our rearing scale. The second move occasion required renovations to the room which delayed increasing fly numbers. In addition, because of University policies related to COVID-19, laboratory experiments were significantly reduced throughout 2020 when the MSc student hired to complete this work was available. In addition to these challenges, the Beauveria samples collected proved challenging to rear and propagate and were difficult to combine with the sugars. This slowed the progress of additional replicates. Because of these operational and time constraints, only experiments that the methodology had been confirmed and the outcome positive were completed.
Regardless, these initial results were promising and new research on non-nutritive sugars has shown even more promise as a bait control for flies. These combinations should be explored in the future.
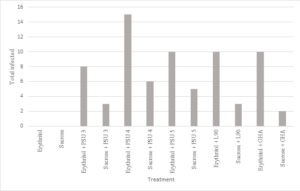
Objective 1 references
Acharya, N., E. G. Rajotte, N. E. Jenkins, and M. B. Thomas. 2015a. Potential for biocontrol of house flies, Musca domestica, using fungal biopesticides. Biocontrol Sci. Technol. 25(5): 513-524.
Acharya, N., E. G. Rajotte, N. E. Jenkins, and M. B. Thomas. 2015b. Influence of biotic and abiotic factors on the persistence of a Beauveria bassiana biopesticide in laboratory and high-rise poultry house settings. Biocontrol Sci. Technol. 25(11): 1317-1332.
Acharya, N., R. A. Seliga, E. G. Rajotte, N. E. Jenkins, and M. B. Thomas. 2015. Persistence and efficacy of a Beauveria bassiana biopesticide against the house fly, Musca domestica, on typical structural substrates of poultry houses. Biocontrol Sci. Technol. 25(6): 697-715.
Anderson, R. D., A. S. Bell, S. Blanford, K. P. Paaijmans, and M. B. Thomas. 2011. Comparative growth kinetics and virulence of four different isolates of entomopathogenic fungi in the house fly (Musca domestica L.). J. Invertebr. Pathol. 107(3): 179-184.
Barin, A., Arabkhazaeli, F., Rahbari, S., and Madani, S. A. 2010. The housefly, Musca domestica, as a possible mechanical vector of Newcastle disease virus in the laboratory and field. Medical and Veterinary Entomology. 24(1): 88-90.
Bruce, N., and Decker, C. 1949. House fly resistance to chemicals. The American journal of tropical medicine and hygiene. 1(3):395-403.
Calibeo-Hayes, D., Denning, S. S., Stringham, S. M., Guy, J. S., Smith, L. G., and Watson, D. W. .2003. Mechanical transmission of turkey coronavirus by domestic houseflies (Musca domestica Linnaeaus). Avian Diseases. 47(1): 149-153.
Daoust, R. A., and R. M. Pereirn. 1986. Stability of Entomopathogenic Fungi Beauveria bassiana and Metarhizium anisopliae on Beetle-attracting Tubers and Cowpea Foliage in Brazil. Environ. Entomol.15(6): 1237-1243.
Freeman, J. C., D. H. Ross, and J. G. Scott. 2019. Insecticide resistance monitoring of house fly populations from the United States. Pestic. Biochem. Physiol. 158: 61–68.
García-Munguía, C., F. Reyes-Villanueva, M. A. Rodriguez-Perez, H. Cortez-Madrigal, M. Acosta-Ramos, L. A. Ibarra-Juárez, M. A. Velázquez-Machuca, J. T. Silva-García, M. Rebollar-Plata, and A. M. Garcia-Munguia. 2015. Autodissemination of Metarhizium anisopliae and Beauveria bassiana in Musca domestica L. Results in Less Oviposition and Short Gonotrophic Cycle. Southwest. Entomol. 40: 519–529.
Gillespie, A. T., and E. Crawford. 1986. Effect of water activity on conidial germina- tion and mycelial growth of Beauve- ria bassiana, Metarhizium anisopliae, Paecilomyces spp. and Verticillium le- canii. In Fundam. Ap- Plied Asp. Invertebr. Pathol. 254.
Goettel, M. S., and D. G. Inglis. 1997. Fungi: Hyphomycetes, Man. Tech. Insect Pathol. (pp. 213-249). Academic Press.
Hajek, A. E. 1999. Ecology of terrestrial fungal entomopathogens. Adv. Microb. Ecol. (pp. 193-249). Springer, Boston, MA.
Holderman, C. J., L. A. Wood, C. J. Geden, and P. E. Kaufman. 2017. Discovery, development, and evaluation of a horn fly-isolated (Diptera: Muscidae) Beauveria bassiana (Hypocreales: Cordyciptaceae) Strain from Florida, USA. J. Insect Sci. 17(2).
Inglis, G. D., M. S. Goettel, and D. L. Johnson. 1995. Influence of ultraviolet light protectants on persistence of the entomopathogenic fungus, Beauveria bassiana. Biol. Control. 5(4): 581-590.
Karanja, L. W., N. A. Phiri, G. I. Oduor, B. Vuellemin, and M. Sorokin. 2004. Effect of Different Solid Substrates on Mass Production of Beauveria bassiana and Metarhizium Anisopliae Entomopathogens. 789–797.
Kaufman, P. E., C. Reasor, D. A. Rutz, J. K. Ketzis, and J. J. Arends. 2005. Evaluation of Beauveria bassiana applications against adult house fly, Musca domestica, in commercial caged-layer poultry facilities in New York state. Biol. Control. 33: 360–367.
Kaufman, P. E., J. G. Scott, and D. A. Rutz. 2001. Monitoring insecticide resistance in house flies (Diptera: Muscidae) from New York dairies. Pest Manag. Sci. 57(6): 514-521.
Keiding, J., and H. Van Deurs. 1949. D.D.T.-resistance in house-flies in Denmark. Nature. 964-965.
Latifian, M., B. Rad, and M. Amani. 2014. Mass production of entomopathogenic fungi Metarhizium anisopliae by using agricultural products based on liquid-solid diphasic method for date palm pest control. Int. J. Farming Allied Sci. 3: 368–372.
Machtinger, E. T., E. N. I. Weeks, and C. J. Geden. 2016. Oviposition deterrence and immature survival of filth flies (Diptera: Muscidae) when exposed to commercial fungal products. J. Insect Sci. 16(1): 54.
Mascarin, G. M., and S. T. Jaronski. 2016. The production and uses of Beauveria bassiana as a microbial insecticide. World J. Microbiol. Biotechnol. 32(11): 177.
Maurer, P., Y. Couteaudier, P. A. Girard, P. D. Bridge, and G. Riba. 1997. Genetic diversity of Beauveria bassiana and relatedness to host insect range. Mycol. Res. 101(2): 159-164.
Meyling, N. V., and J. Eilenberg. 2007. Ecology of the entomopathogenic fungi Beauveria bassiana and Metarhizium anisopliae in temperate agroecosystems: Potential for conservation biological control. Biol. Control. 43(2): 145-155.
Mian, L. S., H. Maag, and J. V. Tacal. 2002. Isolation of Salmonella from muscoid flies at commercial animal establishments in San Bernardino County, California. J. Vector Ecol. 27(1): 82-85.
Rehner, S. A., and E. P. Buckley. 2003. Isolation and characterization of microsatellite loci from the entomopathogenic fungus Beauveria bassiana (Ascomycota: Hypocreales). Mol. Ecol. Notes. 3(3): 409-411.
Scott, J. G. 2017. Evolution of resistance to pyrethroid insecticides in Musca domestica. Pest Manag. Sci. 73(4): 716-722
Scott, J. G., C. A. Leichter, F. D. Rinkevihc, S. A. Harris, C. Su, L. C. Aberegg, R. Moon, C. J. Geden, A. C. Gerry, D. B. Taylor, R. L. Byford, W. Watson, G. Johnson, D. Boxler, and L. Zurek. 2013. Insecticide resistance in house flies from the United States: Resistance levels and frequency of pyrethroid resistance alleles. Pestic. Biochem. Physiol. 107(3): 377-384
Shimazu, M. 1992. Pathogenicity of some entomogenous fungi of the adult housefly, Musca domestica (Diptera: Muscidae). Japanese Journal of Applied Entomology and Zoology. 36(3): 202-203.
Siri, A., A. C. Scorsetti, V. E. Dikgolz, and C. C. L. López Lastra. 2005. Natural infections caused by the fungus Bauveria bassiana as a pathogen of Musca domesticain the neotropic. BioControl. 50(6): 937-940
Skovgård, H., and T. Steenberg. 2002. Activity of pupal parasitoids of the stable fly Stomoxys calcitrans and prevalence of entomopathogenic fungi in the stable fly and the house fly Musca domestica in Denmark. BioControl. 47(1): 45-60.
Steenberg, T., J. B. Jespersen, K. M. Jensen, B. O. Nielsen, and R. A. Humber. 2001. Entomopathogenic fungi in flies associated with pastured cattle in Denmark. J. Invertebr. Pathol. 77(3): 186-197
Steinkraus, D. C., C. J. Geden, D. A. Rutz, and J. P. Kramer. 1990. First Report of the Natural Occurrence of Beauveria bassiana (Moniliales: Moniliaceae) in Musca domestica (Diptera: Muscidae). J. Med. Entomol.
Thomas, G.D., Skoda, S.R. (Eds.). 1993. Rural flies in the urban environment. University of Nebraska, Agric. Res. Div. Res. Bull. 317 ,97pp
Walstad, J. D., R. F. Anderson, and W. J. Stambaugh. 1970. Effects of environmental conditions on two species of muscardine fungi (Beauveria bassiana and Metarrhizium anisopliae). J. Invertebr. Pathol. 16(2): 221-226.
Wanaratana, S., Amonsin, A., Chaisingh, A., Panyim, S., Sasipreeyajan, J., and Pakpinyo, S. 2013. Experimental assessment of houseflies as vectors in Avian Influenza Subtype H5N1 transmission in Chickens. Avian Diseases. 57(2): 266-272.
Watson, D. W., C. J. Geden, S. J. Long, and D. A. Rutz. 1995. Efficacy of Beauveria bassiana for controlling the house fly and stable fly (Diptera: Muscidae). Biol. Control. 5(3): 405-411.
Watson, D. W., Mullens, B. A., and Petersen, J. J. 1993. Behavioral fever response of Musca domestica (Diptera: Muscidae) to infection by Entomophthora muscae (Zygomycetes: Entomophthorales). Journal of Invertebrate Pathology. 61(1): 10-16.
Weeks, E. N. I., E. T. Machtinger, S. A. Gezan, P. E. Kaufman, and C. J. Geden. 2017. Effects of four commercial fungal formulations on mortality and sporulation in house flies (Musca domestica) and stable flies (Stomoxys calcitrans). Med. Vet. Entomol. 31: 15–22.
Objective 2 and 3 references
Acharya, N., E. G. Rajotte, N. E. Jenkins, and M. B. Thomas. 2015a. Potential for biocontrol of house flies, Musca domestica, using fungal biopesticides. Biocontrol Sci. Technol. 25(5): 513-524.
Acharya, N., E. G. Rajotte, N. E. Jenkins, and M. B. Thomas. 2015b. Influence of biotic and abiotic factors on the persistence of a Beauveria bassiana biopesticide in laboratory and high-rise poultry house settings. Biocontrol Sci. Technol. 25(11): 1317-1332.
Acharya, N., R. A. Seliga, E. G. Rajotte, N. E. Jenkins, and M. B. Thomas. 2015. Persistence and efficacy of a Beauveria bassiana biopesticide against the house fly, Musca domestica, on typical structural substrates of poultry houses. Biocontrol Sci. Technol. 25(6): 697-715.
Anderson, R. D., A. S. Bell, S. Blanford, K. P. Paaijmans, and M. B. Thomas. 2011. Comparative growth kinetics and virulence of four different isolates of entomopathogenic fungi in the house fly (Musca domestica L.). J. Invertebr. Pathol. 107(3): 179-184
Anderson, R. D., S. Blanford, N. E. Jenkins, and M. B. Thomas. 2013. Discriminating Fever Behavior in House Flies. PLoS One. 8(4): e62269
Anderson, R. D., S. Blanford, and M. B. Thomas. 2013. House flies delay fungal infection by fevering: At a cost. Ecol. Entomol. 38(1), 1-10.
Barin, A., Arabkhazaeli, F., Rahbari, S., and Madani, S. A. 2010. The housefly, Musca domestica, as a possible mechanical vector of Newcastle disease virus in the laboratory and field. Medical and Veterinary Entomology. 24(1): 88-90.
Bryant, E. H., and L. M. Meffert. 1998. Quantitative Genetic Estimates of Morphometric Variation in Wild-Caught and Laboratory-Reared Houseflies. Evolution (N. Y). 52(2), 626-630.
Burgess, E. R., Johnson, D. M., and Geden, C. J. 2018. Mortality of the house fly (Diptera: Muscidae) after exposure to combinations of Beauveria bassiana (Hypocreales: Clavicipitaceae) With the Polyol Sweeteners Erythritol and Xylitol. Journal of Medical Entomology. 55(5):1237-1244.
Butt, T. M., Jackson, C. W., & Magan, N. 2009. Introduction - fungal biological control agents: progress, problems and potential. In Fungi as biocontrol agents: progress, problems and potential. (T. M. Butt, C. Jackson, and N. Magan, eds.), pp. 1–8. CABI Publishing, New York.
Butt, T. M., Wraight, S. P., Galaini-Wraight, S., Humber, R. A., Roberts, D. W., & Soper, R. S..1988. Humoral encapsulation of the fungus Erynia radicans (Entomophthorales) by the potato leafhopper, Empoasca fabae (Homoptera: Cicadellidae). Journal of Invertebrate Pathology. 52(1): 49-56.
Calibeo-Hayes, D., Denning, S. S., Stringham, S. M., Guy, J. S., Smith, L. G., and Watson, D. W. .2003. Mechanical transmission of turkey coronavirus by domestic houseflies (Musca domestica Linnaeaus). Avian Diseases. 47(1): 149-153.
Dubovskiy, I. M., M. M. A. Whitten, O. N. Yaroslavtseva, C. Greig, V. Y. Kryukov, E. V. Grizanova, K. Mukherjee, A. Vilcinskas, V. V. Glupov, and T. M. Butt. 2013. Can Insects Develop Resistance to Insect Pathogenic Fungi? PLoS One. 4 (2013): e60248.
Fargues, J., & Remaudiere, G. 1977. Considerations on the specificity of entomopathogenic fungi. Mycopathologia. 62(1): 31-37
Geden, C. J. 2002. Effect of Habitat Depth on Host Location by Five Species of Parasitoids (Hymenoptera: Pteromalidae, Chalcididae) of House Flies (Diptera: Muscidae) in Three Types of Substrates. Environ. Entomol. 31(2): 411-417.
Geden, C. J., Rutz, D. A., and Steinkraus, D. C. 1995. Virulence of different isolates and formulations of Beauveria bassiana for house flies and the parasitoid Muscidifurax raptor. Biological Control. 5(4): 615-621.
Gillespie, A. T., & Claydon, N. 1989. The use of entomogenous fungi for pest control and the role of toxins in pathogenesis. Pesticide Science. 27(2): 203-215.
Gillespie, J. P., Bailey, A. M., Cobb, B., & Vilcinskas, A. 2000. Fungi as elicitors of insect immune responses. Archives of Insect Biochemistry and Physiology. 44(2): 49-68.
Hajek, A. E., & St Leger, R. J. 1994. Interactions between fungal pathogens and insect hosts. Annual Review of Entomology. 39(1): 293-322.
Hasaballah, A. I., M. A. Fouda, M. I. Hassan, and G. M. Omar. 2017. Pathogenicity of Beauveria bassiana and Metarhizium anisopliae on the adult housefly, Musca domestica L. Egyptian Academic Journal of Biological Sciences. A, Entomology. 10: 79-86.
Johnson, D. M., Weeks, E. N. I., LoVullo, E. D., Shirk, P. D., & Geden, C. J. 2019. Mortality effects of three bacterial pathogens and Beauveria bassiana when topically applied or injected into house flies (Diptera: Muscidae). Journal of Medical Entomology. 56(3): 774-783.
Kaplan, E. L., & Meier, P. 1958. Nonparametric estimation from incomplete observations. Journal of the American Statistical Association. 53(282): 457-481.
Kaufman, P., Rutz, D., & Pitts, C. 2000. Pest management recommendations for poultry.Retrieved from https://ecommons.cornell.edu/handle/1813/42385 .New York State IPM Program.
Kershaw, M. J., Moorhouse, E. R., Bateman, R., Reynolds, S. E., & Charnley, A. K. 1999. The Role of destruxins in the pathogenicity of Metarhizium anisopliae for three species of insect. Journal of Invertebrate Pathology. 74(3): 213-223.
Lecuona, R., Crespo, D., & La Rossa, F. 2007. Populational parameters of Spalangia endius walker (Hymenoptera: Pteromalidae) on pupae of Musca domestica L. (Diptera: Muscidae) treated with two strains of Beauveria bassiana (Bals.) Vuil. (Deuteromycetes). Neotropical Entomology. 36(4): 537-541
Liu, H., and L. S. Bauer. 2008. Microbial control of emerald ash borer, Agrilus planipennis (Coleoptera: Buprestidae) with Beauveria bassiana strain GHA: Greenhouse and field trials. Biol. Control. 45(1): 124-132.
Ludwig, S. W., and R. D. Oetting. 2002. Efficacy of Beauveria bassiana Plus Insect Attractants for Enhanced Control of Frankliniella occidentalis (Thysanoptera: Thripidae) Florida Entomol. 85(1): 270-272.
Luz, C., & Batagin, I. 2005. Potential of oil-based formulations of Beauveria bassiana to control Triatoma infestans. Mycopathologia, 160(1): 51-62.
Machtinger, E. T., Weeks, E. N. I., and Geden, C. J. 2016. Oviposition deterrence and immature survival of filth flies (Diptera: Muscidae) when exposed to commercial fungal products. Journal of Insect Science. 16(1): 54.
Machtinger, E. T., Weeks, E. N. I., Geden, C. J., and Kaufman, P. E. 2016. House fly (Musca domestica) (Diptera: Muscidae) mortality after exposure to commercial fungal formulations in a sugar bait. Biocontrol Science and Technology. 26(10): 1444-1450
Malik, A., Singh, N., and Satya, S. 2007. House fly (Musca domestica): A review of control strategies for a challenging pest. Journal of Environmental Science and Health - Part B Pesticides, Food Contaminants, and Agricultural Wastes.42(4): 453-469.
Maurer, P., Couteaudier, Y., Girard, P. A., Bridge, P. D., & Riba, G. 1997. Genetic diversity of Beauveria bassiana and relatedness to host insect range. Mycological Research. 101(2): 159-164.
Mishra, S., P. Kumar, and A. Malik. 2015. The effect of Beauveria bassiana infection on cell mediated and humoral immune response in house fly, Musca domestica L. Environ. Sci. Pollut. Res. 22(19): 15171-15178.
Moore, T. C. 2018. Counting cells with hemocytometer. Protocols.Io. dx.doi.org/10.17504/protocols.io.nxsdfne
Shimazu, M. 1992. Pathogenicity of some entomogenous fungi of the adult housefly, Musca domestica (Diptera: Muscidae). Japanese Journal of Applied Entomology and Zoology. 36(3): 202-203.
Steinkraus, D. C., Geden, C. J., Rutz, D. A., and Kramer, J. P. 1990. First report of the natural occurrence of Beauveria bassiana (Moniliales: Moniliaceae) in Musca domestica (Diptera: Muscidae). Journal of Medical Entomology. 27(3): 309-312.
Trauer, U., and M. Hilker. 2013. Parental Legacy in Insects: Variation of Transgenerational Immune Priming during Offspring Development. PLoS One. 8(5), e63392.
Uma Devi, K., Padmavathi, J., Uma Maheswara Rao, C., Khan, A. A. P., & Mohan, M. C. 2008. A study of host specificity in the entomopathogenic fungus Beauveria bassiana (Hypocreales, Clavicipitaceae). Biocontrol Science and Technology. 18(10): 975-989.
Vestergaard, S., Cherry, A., Keller, S., & Goettel, M. 2003. Safety of Hyphomycete Fungi as Microbial Control Agents. In Environmental Impacts of Microbial Insecticides. (pp. 35-62). Springer, Dordrecht.
Viaud, M., Couteaudier, Y., Levis, C., & Riba, G. 1996. Genome organization in Beauveria bassiana: Electrophoretic karyotype, gene mapping, and telomeric fingerprint. Fungal Genetics and Biology. 20(3): 175-183.
Wanaratana, S., Amonsin, A., Chaisingh, A., Panyim, S., Sasipreeyajan, J., and Pakpinyo, S. 2013. Experimental assessment of houseflies as vectors in Avian Influenza Subtype H5N1 transmission in Chickens. Avian Diseases. 57(2): 266-272.
Watson, D. W., C. J. Geden, S. J. Long, and D. A. Rutz. 1995. Efficacy of Beauveria bassiana for controlling the house fly and stable fly (Diptera: Muscidae). Biol. Control. 5(3): 405-411.
Weeks, E. N. I., E. T. Machtinger, S. A. Gezan, P. E. Kaufman, and C. J. Geden. 2017. Effects of four commercial fungal formulations on mortality and sporulation in house flies (Musca domestica) and stable flies (Stomoxys calcitrans). Med. Vet. Entomol. 31: 15–22.
Wraight, S. P., Ramos, M. E., Avery, P. B., Jaronski, S. T., & Vandenberg, J. D. 2010. Comparative virulence of Beauveria bassiana isolates against lepidopteran pests of vegetable crops. Journal of Invertebrate Pathology. 103(3): 186-199.
- Beauveria bassiana can be collected in poultry facilities from house flies, but at very low levels.
- Strains of Beauveria bassiana collected from flies in poultry facilities may perform differently, even if collected from the same facility.
- Some strains of Beauveria bassiana were detrimental to some parasitoids, but S. endius was more resistant and may be more effective than other species if B. bassiana is used.
- Several house fly-specific strains of Beauveria bassiana could be adapted for use as biological control on poultry facilities and this should be pursued as there is a desperate need for organic-friendly options of fly control.
Education
The proposed educational solution to the problem of pest control on poultry facilities in Pennsylvania was to create a short-course in poultry pest management focused on biological control options and appropriate use for fly control, hosted by Penn State Extension. The outline of the online short course was created and included:
Introduction
Section 1: Damage caused by pest flies in poultry facilities
Section 2: Integrated Pest Management
Section 3: IPM step 1: Pest fly identification
Section 4: IPM step 2: Pest fly life cycles
Section 5: IPM step 3: Monitoring
Section 6: IPM step 4: Action thresholds
Section 7: IPM step 5: Deciding on control methods
Section 8: IPM Control: Cultural Control
Section 9: IPM Control: Physical and Mechanical Control
Section 10: IPM Control: Biological Control
Section 11: IPM Control: Chemical Control
Section 12: IPM: Putting it all together
Section 13: Extra module: Natural Resources professionals
Final thoughts
Resources
Glossary
Milestones
Establish monitoring and record-keeping - 20 producers will establish a fly monitoring program or continue a fly monitoring program and will maintain records on fly control expenditures.
20
38
August 31, 2019
Incomplete
A survey was distributed to course registrants to determine practices relate to fly control and IPM. Because of COVID-19, we could not visit farms to assist farmers with establishing monitoring procedures prior to the course. However, the survey demonstrated that greater than 50% of respondents reported that they have had fly problems in the past. 32% of respondents spend $50-$500 a year on fly control, and 27% spend $500-$10,000 on fly control. More than 50% of respondents use chemical control most of the time. 75% of respondents did not have an IPM plan, 50% do not monitor flies, and more than 50% were not confident they could control pest flies.
Pilot Testing - Ten producers will be given the pilot of the completed short-course and feedback will be used to update the course.
10
10
April 30, 2020
Completed
November 01, 2020
The short-course was pilot tested and corrections or clarifications were made before the course was finalized.
Recruitment - At least 500 poultry producers will receive direct mailings, emails, or social media information for registration. Advertisements will be placed with/in poultry organizations and magazines.
500
500
October 31, 2020
Completed
February 02, 2021
Announcements were made via social media, email, and post-cards sent to Penn State Extension contacts. At least 500 producers were reached based on email numbers, social media interactions, and mailings.
Pre-training engagement - At least 100 poultry producers will register and complete the short-course (prior to the emergence of most poultry pests). These participants will answer questionnaire questions regarding current practices and take a pre-test to analyze their current knowledge.
100
38
March 31, 2021
Completed
March 01, 2021
38 participants registered for the online course. This number may have been increased with additional time for enrollment. However, because of COVID-19 our production was shut down and that delayed the course development. Therefore, we had less time to advertise and have participants enroll.
Learning through the education program - 100 registrants will:
• Work through specific fly pest of poultry modules.
• Gain knowledge on biological information of fly pests of poultry (as described in the educational approach).
• Learn about monitoring methods, and action thresholds/economic thresholds.
• Learn about integrated pest management, and the ways to control fly pests of poultry.
• Develop an IPM plan for their farm for fly pests and learn about methods to evaluate that plan.
• Receive a certificate of completion.
100
38
March 31, 2021
Completed
June 01, 2021
38 participants completed the course on poultry pest management in the time alloted. For the purposes of this project, the course was free to take. This number may have been increased with additional time for enrollment. However, because of COVID-19 our production was shut down and that delayed the course development. Therefore, we had less time to advertise and have participants enroll.
Support to take follow-up action - 100 poultry producers will submit their IPM plans to the educators at the completion of the course. All 100 will have access to educators will be provided throughout the course.
100
38
March 31, 2021
Completed
June 16, 2021
38 participants created individual IPM plans and submitted them to the education collaborators for review. This number may have been increased with additional time for enrollment. However, because of COVID-19 our production was shut down and that delayed the course development. Therefore, we had less time to advertise and have participants enroll.
At least 100 producers will visit the “Bug Bytes” blog during the first project spring and summer and 200 the second project spring and summer.
100
September 30, 2022
Incomplete
After communication with Penn State Extension, it was determined that bug bytes could be distributed through the current social media and email lists associated with the Penn State Poultry Team. The PI (Machtinger) is now a part of this extension team and regularly meets with the group to promote timely reminders of pest control.
Verification of actions and resulting benefits - 75 producers will respond to verification survey to report actions taken to develop and execute an IPM plan and incorporate biological control for fly management.
75
28
September 30, 2021
Completed
December 01, 2021
The results of the post-course survey demonstrated that 61% felt the course helped them change how they managed flies. 31% decreased the amount they spent on fly control and 41.7% decreased the amount they spent on chemical control specifically. Only 16% of respondents said they used chemical control half the time or more. 88.3% reported that they have regularly updated their IPM plan and 72% reported they now monitor flies. 83% reported they felt somewhat confident or confident that they could manage flies on their facility after taking the course.
Milestone Activities and Participation Summary
Educational activities:
Participation Summary:
Learning Outcomes
Pre- and post- surveys were used associated with the fly management course. Of the respondents from the post-surveys, 61% felt the course helped them manage flies. Confidence in their ability to manage flies increased by 33%. IPM plan adoption increased by 63.3%. 41.7% of respondents decreased their use of chemical control methods and 16% reduced the frequency. Monitoring flies increased by 22%.
Performance Target Outcomes
Target #1
40
Adoption of an IPM plan for fly control.
640,000 birds
Savings of $200 in reduction of chemical application
38
Adoption of an IPM plan
340,000 birds
While we couldn't measure chemical expenditure directly because of COVID restrictions, chemical expenditures before the course and IPM plan adoption ranged from an average expenditure of $1,603-$3,996 per person to $270-$952 per person post-course.
We had 38 participants attending the synchronous course. This number may have been increased with additional time for enrollment. However, because of COVID-19 our production was shut down and that delayed the course development. Therefore, we had less time to advertise and have participants enroll. However, although we had half the number of participants we expected, they represented more than half of the number of birds. In order to pass the course to get a certificate, an IPM plan based on the course material was required. In this way, we could ensure that the participants would adopt an IPM plan for their facility. We also had a range of product types and farm sizes. Nearly 75% of the course takers responded to the post-survey. Feedback on the course was very positive, and the development of the course also resulted in 9 shorter "Learn Now' videos.
Information Products
- Managing Insecticide Resistance on Animal Facilities (Video)
- Integrated Pest Management (IPM) of Nuisance Flies on Animal Facilities (Video)
- Cultural Methods for Nuisance Fly Control in Poultry Facilities (Video)
- Monitoring Nuisance Flies in Poultry Facilities (Video)
- Setting Action Thresholds for Nuisance Flies in Poultry Facilities (Video)
- Understanding Nuisance Fly Life Cycles in Poultry Facilities (Video)
- Poultry Fly Management (Course or Curriculum)