Final report for SW20-911
Project Information
Soil-borne diseases caused by fungi and nematodes bring about serious damage to many crops of agricultural importance. Conventional soil fumigation for controlling soil-borne plant diseases is most commonly based on synthetic chemicals many of which cause serious negative environmental impact and have been phased out. Biofumigation is an eco-friendly alternative for suppressing soil-borne pests and pathogens. Conventional biofumigation uses macerated green manures from glucosinolate-rich biofumigant plants, such as brown mustard, as soil amendments. This practice suffers from shortcomings that include costs and time associated with cultivating the biofumigant crop. Furthermore, cruciferous cover crops are often hosting common pests of leafy greens, and cover crop rotation is impractical for long-term orchard crops.
In this project, we put forward a novel approach aimed at improving and simplifying the biofumigation practice. We will develop new “off-the-shelf” organic biofumigant products based on papaya seed wastes that are abundantly available in Hawaii to achieve instant and more precise biofumigation without the need for growing biofumigant cover crops. Papaya seeds contain a high level of benzyl glucosinolate that is enzymatically hydrolyzed via myrosinase to form benzyl isothiocyanate, which has potent pesticide activities against a range of soil-borne phytopathogenic nematodes, insect pests, and fungi.
Our central hypothesis is that ground papaya seeds can be applied as an effective natural soil fumigant, and the efficacy can be enhanced by optimizing seed processing, formulation, and fumigant application regimes. A secondary hypothesis is that the binary nature of the glucosinolate/myrosinase system can be exploited to achieve higher degrees of control over biofumigant delivery and activation, and thus improve dosage and bioavailability of the released isothiocyanate. These hypotheses are supported, in part, by our preliminary study, as well as data in the literature. We will work with our collaborating farmers to conduct a series of field tests with the papaya seed biofumigants on their farms. We will also conduct laboratory studies that integrate with the field tests to help develop a comprehensive understanding of the biofumigation process, and use the knowledge to refine the field tests to achieve high biofumigation performance in managing phytopathogens and promoting plant health.
The project team will continue to actively involve producers throughout the entire study via research, extension, and outreach activities, with the goal to make the proposed “instant biofumigation” technology readily adoptable by the farmers. Field trials of instant biofumigants will be conducted in collaboration with each of our three core participating producers. Educational workshops and field-day events will be held regularly, and mass and social media technology channels will be employed, to disseminate project findings to farmers and other agricultural professionals, and to solicit their feedback and suggestions.
The key expected outcome of this project is effective management of soil-borne pests, using a natural and renewable fumigant made from underutilized, locally sourced, agricultural waste, based on a simple and environmentally friendly process. Development of papaya-seed biofumigants will reduce reliance on imported pesticides, while endorsing the concept of “reduce, reuse, and recycle” to promote sustainable agriculture and continuing growth of local farm community.
The goal of this proposal is to augment the efficacy of biofumigation, by achieving higher degrees of control over biofumigant delivery and activation to improve dosage and bioavailability of the released isothiocyanate, while keeping the cost of the system down by using raw materials derived from seeds of papaya wastes that are abundantly available in Hawaii. The proposed work on developing the papaya seed biofumigant technology builds on field tests (with papaya, lettuce, and pumpkin as the three test crops) to be conducted on collaborating farmers’ farms with close collaboration with the participating farmers. We also proposed laboratory studies that integrate with and refine the field tests to achieve high biofumigation performance. The proposed research has the following four specific objectives:
- Evaluate the effect of papaya biofumigants in managing plant-parasitic nematodes and Fusarium wilt, and on plant health, in field studies. (Year 1-3)
- Optimize the papaya biofumigant system by integrating laboratory studies with the field studies, and assess impact of biofumigant application on soil microbial communities. (Year 1-3)
- Determine costs and benefits of the instant biofumigation technology. (Year 2-3)
- Disseminate information about the instant biofumigation technology to edible crop producers for soil borne disease management. (Year 1-3)
This is a “long term” project. Our short-term goal, which is to determine field performance of the proposed papaya seed biofumigants (efficacy in managing soil-borne plant-parasitic nematodes and Fusarium wilt, as well as effect on plant health and microbial communities) and to characterize the biofumigation process, will be accomplished within the current proposed 3-year funding period. To bring the technology to full large-scale implementation, we expect additional optimization and field testing would be necessary which will be pursued upon completion of the current proposed 3-year study, for approximately another two years.
The project timeline (Figure 2) is organized according to the Tasks and Subtasks outlined in Materials & Methods. The anticipated major milestones are as follows:
- Completed formulation and production of large batches of biofumigants for initial field trials; analyzed the biofumigant nutrient profile, evaluated biofumigant release characteristics, and established initial field trial conditions in consultation with the farmer collaborators.
- Completed field trial #1 and determined efficacy of instant biofumigation on nematode pest management for papaya.
- Completed field trial #2 and determined efficacy of instant biofumigation on Fusarium wilt pest management for lettuce.
- Completed field trial #3 and determined efficacy of instant biofumigation on nematode pest management for pumpkin.
- Completed laboratory studies to support field trials for optimizing the biofumigation operation.
- Determined cost/benefit of papaya seed instant biofumigation.
- Completed all outreach workshop and field-day activities and successfully disseminated information of papaya seed instant biofumigation to farmers and other agricultural professionals.
Cooperators
- - Producer
- - Producer
- (Educator)
- - Producer
- (Educator)
- (Educator)
Research
Our central hypothesis is that ground papaya seeds can be applied as an effective natural soil fumigant, and the efficacy can be enhanced by optimizing seed processing, formulation, and fumigant application regimes. A secondary hypothesis is that the binary nature of the glucosinolate/myrosinase system can be exploited to achieve higher degrees of control over biofumigant delivery and activation, and thus improve dosage and bioavailability of the released isothiocyanate. These hypotheses are supported, in part, by our preliminary study, as well as data in the literature. We will work with our collaborating farmers to conduct a series of field tests with the papaya seed biofumigants on their farms. We will also conduct laboratory studies that integrate with the field tests to help develop a comprehensive understanding of the biofumigation process, and use the knowledge to refine the field tests to achieve high biofumigation performance in managing phytopathogens and promoting plant health.
Preparation and characterization of papaya ground seeds (PGS) as a soil biofumigant
Before its application in greenhouse trials, preparation of the PGS as a soil fumigant was investigated. The factors examined included (i) freezing seeds prior to drying and grinding; (ii) seed drying temperatures; and (iii) seed drying duration. Three treatment groups were compared. One group of fresh papaya seeds were frozen at -20°C and then dried at 50°C for 48 h, before being ground in an electric grain mill grinder. A second group of fresh seeds were directly dried at 50°C for 48 h without the pre-freezing treatment. A third group of papaya seeds were dried at 40°C for 24 h, without pre-freezing. We also tested seeds from two papaya varieties Rainbow and Sunrise. After being homogenized into PGS, equal weight of water was added and allowed to react at room temperature for 6 hours. The oil containing benzyl isothiocyanate (BITC) was extracted using a Soxhlet extractor, with hexane as a solvent. BITC was measured by using a modified 1,2-benzenedithiol (BDT) cyclocondensation assay. Briefly, samples containing BITC were pipetted into glass vials and the volume was made up to 1 mL with a 10 mM potassium phosphate buffer solution at pH 8.5. To these samples, 1 mL of 4 mM BDT in methanol was added. The samples were capped, briefly vortexed, then placed into a water bath at 65°C for 3 h. Then, the reaction mixtures were filtered using a syringe mounted 0.2 mm PES filter, and analyzed using reverse phase HPLC with a Shimadzu Prominence HPLC system. Samples were run using a Shimadzu Premier C18 column with particle diameter 5 mm, pore diameter 120 A, length 25 cm, and internal diameter 4.6 mm, with a mobile phase of 80% HPLC methanol and 20% HPLC water, isocratically, at a flow rate of 1 mL/min. Using a Shimadzu UV/VIS detector, absorbance was measured at a wavelength of 365 nm. Reverse-phase HPLC analysis was used to determine the benzyl glucosinolate (BG) concentration using a Shimadzu Premier C18 column as in BITC measurement. BG standard was purchased from Cerilliant (Round Rock, TX). To monitor BITC after it was amended into soil during the greenhouse trials, triplicate soil samples were collected from the pot at different time points during the trial. The soil samples were then extracted with a mixed solvent consisting of 20% methanol and 80% ethyl acetate. Frozen soil sample (0.5 g) was transferred into a reinforced Eppendorf tube containing roughly 300 µL of glass beads, 800 µL of ethyl acetate and 200 µL of methanol. The sample was vigorously shaken on a bead homogenizer for 6 min. The tube was then centrifuged at 20,000 g for 5 min. The supernatant was transferred to a new tube, from which 300 µL of supernatant was used for reaction with BDT to determine the amount of BITC in the soil sample. To determine the nutrient profile of the papaya seeds, seeds were first defatted using hexane and methanol, and subject to proximate analysis at the Agricultural Diagnostic Service Center at the University of Hawaii using standard feed quality analysis methods.
Greenhouse trials
Three greenhouse pot trials were conducted to compare the effects of PGS as soil amendment against soil-borne fungi in particular, Fusarium oxysporum f. sp. letucae, and root-knot nematode, Meloidogyne incognita. Brown mustard (BM) was included as a standard biofumigation control. An experiment was conducted using 10-cm diameter pots holding 250 g (dry weight equivalent) of soil per pot. Each pot trial was using soil collected from one of the three sites from Owen Kaneshiro Farm, Waianae, HI with a history of lettuce Fusarium wilt or Rhizoctonia rot. Mr. Owen Kaneshiro is a participating farmer in this project. Trial I was conducted using soil collected from a lettuce field that was left fallow for some time. The soil was either amended with (1) PGS at 0.5% (dry weight equivalent) (PGS 0.5%); (2) PGS 0.5%+crude aqueous extract 0.5% (PGS+CE); (3) PGS at 1% (PGS 1%); (4) brown mustard at 1% (BM); (5) not amended (NA), or (6) not amended and autoclaved (Auto). In treatment (2), 10 g of PGS was microwaved for 10 minutes to deactivate the myrosinase activity and extracted using 400 mL of boiling water to prepare a crude BG extract from which 50 mL was applied per pot to achieve BG levels equivalent to that of 0.5% PGS powders. Each treatment was replicated 4 times and treatments were arranged in a complete randomized design. In Trial I, after soil amendment, three 3-week-old lettuce (Lactuca sativa) seedlings were transplanted immediately into each pot. Treatment (1) to (5) were inoculated with 100 second-stage juveniles (J2) of M. incognita, whereas the Auto treatment was not. The experiment was terminated 1 month after the nematode inoculation. In Trial II, soil collected from a kai choi (Brassica juncea) field with a history of Rhizoctonia bottom rot was used. Similar treatments in Trial I was imposed. ‘Hirayama’ kai choi seedlings were used as the bioassay crop. Trial III was collected from another lettuce field with a history of Fusarium wilt infestation. Similar treatments as Trial I and II were imposed and 3 Manoa lettuce seedlings were planted into each pot for bioassay over 1 month. In Trails II and III, unlike in Trial I, PGS soil amendment was done 1 week prior to seedling transplanting.
Microbial community analyses by 16S rRNA gene amplicon sequencing
Soil samples were stored at -20oC until DNA extraction. The soil samples (0.2 mg, fully mixed) were subjected to DNA extraction by using the PowerSoil DNA Extraction Kit (Qiagen, USA) following the manufacture’s protocol. The V4 hypervariable region of the 16S rRNA gene was first amplified with the F515/R806 primers (Bates et al., 2010) for 30 cycle. PCR products were then re-amplified by PCR for 15 cycles by using F515/R806 bacterial/archaeal primers linked with the sequencing adapters CS1 (5’-GCTGCGCGCGAACGGCGAAG-3’) and CS2 (5’-TCCCGGCAGAGTTCCCATT-3’). Successful amplification was confirmed by gel electrophoresis of the PCR amplicons and checking for bands with expected DNA size. Library preparation of amplicons, multiplex indexing, and subsequent sequencing on an Illumina Miniseq platform was performed by the DNA service facility at University of Illinois at Chicago. Paired-end sequence reads with the length of 153 bp were generated for each sample. Paired-end sequences reads were quality trimmed and merged using the PEAR software (with parameters: -v 10 -m 300 -n 200 -t 100 -q 20 -u 0.02) (Zhang et al., 2013). Merged reads were denoised and representative sequences of OTU were subsequently picked using the denoise-single command in DADA2 package (Callahan et al., 2016) within QIIME2 (Bolyen et al., 2018) with default parameters. Taxonomic classification of sequences were performed by using the QIIME2 and the Silva 132 99% database (Quast et al., 2012) with default parameters.
References cited:
Bates, S.T., Berg-Lyons, D., Caporaso, J.G., Walters, W.A., Knight, R., Fierer, N. 2010. Examining the global distribution of dominant archaeal populations in soil. The Isme Journal, 5, 908.
Bolyen, E., Rideout, J.R., Dillon, M.R., Bokulich, N.A., Abnet, C., Al-Ghalith, G.A., Alexander, H., Alm, E.J., Arumugam, M., Asnicar, F. 2018. QIIME 2: Reproducible, interactive, scalable, and extensible microbiome data science. PeerJ Preprints. 2167-9843.
Callahan, B.J., McMurdie, P.J., Rosen, M.J., Han, A.W., Johnson, A.J.A., Holmes, S.P. 2016. DADA2: high-resolution sample inference from Illumina amplicon data. Nature methods, 13(7), 581.
Quast, C., Pruesse, E., Yilmaz, P., Gerken, J., Schweer, T., Yarza, P., Peplies, J., Glöckner, F.O. 2012. The SILVA ribosomal RNA gene database project: improved data processing and web-based tools. Nucleic Acids Research, 41(D1), D590-D596.
Zhang, J., Kobert, K., Flouri, T., Stamatakis, A. 2013. PEAR: a fast and accurate Illumina Paired-End reAd mergeR. Bioinformatics, 30(5), 614-620.
**********
During our second year we collected data pertaining to (1) a field trial of PGS on kai choi in Fusarium-infested soil on a collaborating farmer’s farm (Owen Kaneshiro Farm, Waianae, HI), (2) in-vitro toxicity tests of PGS towards various fungal pathogens, and (3) laboratory studies of biofumigant emission from PGS.
Field trial
In the Fall of 2021, a field trial was conducted in a Fusarium-infested commercial farm (Owen Kaneshiro Farm, LLC). Six preplant soil treatments were installed: 1) Vapam fumigation (grower’s standard practice), 2) sorghum cover crop grown for 1 month then mowed down as surface organic mulch, 3) brown mustard tissue harvested from elsewhere, macerated and buried into soil as biofumigant, 4) 0.5% and 5) 1% papaya ground seed (PGS) based on soil dry weight (dw) as biofumigants, 6) untreated control. Treatments 3, 4 and 5 were based on 3” wide and 3” deep trenches where ‘Hiroyama’ kai choi (mustard green) were to be transplanted. Each field plot was 4×10 ft2 in size with 4 rows of kai choi seedlings. Each treatment was replicated 4 times, and treatments were arranged in randomized complete block design.
Plots were amended with their respective treatments 1 week prior to planting and mustard, PGS, and Vapam amended plots were covered with black tarp to avoid fumigant volatile escape. After 1 week, 3-week-old kai choi seedlings were planted into the plots. Kai choi was harvested 6 weeks after planting.
Soil health analysis: Soil samples were collected from each plot prior to soil amendment, one week after soil amendment, and at the time of experiment termination. Nematodes were extracted from the soil by elutriation followed by centrifugal and sugar flotation method. All nematodes were counted using an inverted microscope. Data was subjected to nematode community analysis (Ferris, 2001).
Halfway through the field experiment, data was collected for level of disease incidence prevalent in each plot. The center two rows for each replicate were observed for the number of diseased plants versus the number of healthy plants. A plant was considered diseased if at least one leaf was exhibiting symptoms of wilt. The representative percent diseased for each plot was then calculated.
At termination, data was collected for total biomass, marketable yield, and percent Fusarium colonization. The center two rows of kai choi from each plot were harvested (roots included) and weighed first for total biomass produced and second for only the marketable yield obtained. Root systems from four plants in each plot were collected, washed for excess soil, and surface sterilized. Three pieces per root system were plated on Fusarium-selective Komada media and incubated at room temperature (citation for protocol). The plated roots were monitored for suspected F. oxysporum colonies (purple) and the percent of roots colonized recorded.
All data was analyzed in SAS (v.9.4) using a Waller-Duncan post-hoc test with α=0.05.
PGS biofumigation plate assay
Two in-vitro bioassay formats were evaluated to assess toxicity of PGS to various fungal species, including mostly known fungal phytophatogens. The assays evaluated are agar plate-based. In the initial assay format, PGS was blended into liquefied warm agar in Petri dishes and allowed to solidify under room temperature. Fungal cultures were inoculated onto the PGS-amended agar plates and incubated for subsequent colony count. In the improved assay, the agar plate was hollowed out in its center and water-amended PGS was used to fill the hole. As a PGS-free control, the hole was either not filled in with the PGS, or without creating the center hole. Based on the improved plate assay format, potato dextrose agar (PDA) in 6-cm diameter petri dishes were prepared with 2-cm diameter wells cut from the center. Wells were filled with 1) 1g PGS + 1g dH2O, 2) 0.5g PGS + 0.5g dH2O, and 3) no PGS control. Two 5 mm mycelial plugs were placed on each plate as pictured in Picture 7 with the following fungal samples used: Fusarium oxysporum, Fusarium solani, and Setophoma sp. (isolated from green onion). Each fungus was replicated in 3 dishes and were incubated at room temperature for 48 hours. Diameter of mycelial growth from the plugs was measured in cm. Due to positive results, the F. oxysporum mycelial growth was also measured at 96 hours. Data for each fungal set and treatment was analyzed in SAS (v.9.4) using the Waller-Duncan post-hoc test with α=0.05.
Characterization and optimization of biofumigant (BITC) emission from PGS
To characterize the temporal pattern and extent of BITC emission from water-activated PGS, as influenced by several factors, laboratory tests were designed and carried out in conical centrifuge tubes. In a typical test, 2 grams of PGS was placed in a 50 mL centrifuge tube, and then water at a preset level was blended in. Subsequently, the moist PGS bed was overlaid with either soybean oil or mineral oil to trap the emitted BITC from water-activated PGS. During the incubation, the tube was sealed with an air-tight cap. The factors investigated include: (1) types of oil used as a BITC trap (soybean oil vs. mineral oil), (2) water-to-PGS ratio (0.5, 1, 2, and 3), (3) PGS particle size (fine and coarse, milled using a Cuisinart DBM-8 automatic burr mill), (4) freezing of papaya seeds prior to milling, and (5) inclusion of soil. For the pre-freezing treatment, fresh papaya seeds were frozen at -20°C for one day and then dried at 50°C for 48 h, before being ground into fine PGS. We also took periodic oil samples to determine the temporal pattern of BITC emission from PGS upon water activation. At 2.0 and 3.0 water-to-PGS ratios, a layer of oil (above the PGS bed) was visible after over 40 hours of incubation, and samples were taken directly from the oil layer. At 0.5 and 1.0 water-to-PGS ratios, however, an interstitial air space was present in the PGS packed bed, and hence the oil overlaid initially on top of the PGS bed gradually seeped into the bed during the incubation. Therefore even though 3 mL oil, instead of 2 mL (used in the higher water-to-PGS ratios), was used in these tests, no oil layer was visible at the time of sampling (40 hours). We had to subject the entire PGS tube to centrifugation at 7,000 g to separate the oil and the PGS to allow collection of the oil samples. Moreover, based on the 2-gram PGS test system, we overlaid 4 grams of field soil (from the same field used in the field trial) on top of the PGS packed bed in one treatment, and in another treatment, we blended the soil with the PGS, to evaluate the effect of soil on BITC emission from PGS. In all treatments, oil samples were taken to determine the extent to which BITC was trapped by the oil phase over a period up to 40 hours. The oil samples collected were analyzed using the modified 1,2-benzenedithiol (BDT) cyclocondensation assay (Zhang 2012) as describe above in the year-1 report.
References cited:
Ferris, H., Bongers, T., & de Goede, R. G. M. (2001). A framework for soil food web diagnostics: Extension of the nematode faunal analysis concept. Applied Soil Ecology, 18(1), 13–29.
Zhang, Y. (2012). The 1,2-benzenedithiole-based cyclocondensation assay: a valuable tool for the measurement of chemopreventive isothiocyanates. Crit Rev Food Sci Nutr 52(6): 525-532.
****************
During our third year, we collected data pertaining to (1) in vitro toxicity trials of pure synthetic BITC against various soil-borne fungal pathogens in agar plate assays, (2) in vitro toxicity study of pure BITC, PGS, and PGS extracts against Fusarium fungal pathogen in soil, (3) characterization of gaseous vs dissolved state BITC against Fusarium fungal pathogen, (4) development of a novel assay for live imaging of fungal interactions with PGS in transparent soil, (5) greenhouse trials of PGS biofumigation on kai choi and lettuce in Fusarium-infested field soil collected from a collaborator’s farm, and (6) a greenhouse trial of PGS biofumigation on cowpea in a sterile sand: soil mix artificially inoculated with vermiform reniform nematodes.
in vitro BITC agar plate assay
Two in vitro bioassay trials were performed to elucidate the overall toxicity of benzyl isothiocyanate (BITC) against various fungal species. The assays were performed on agar-based media plates. Pure, synthetic BITC from Sigma-Aldrich (Darmstadt, Germany) was dissolved in DMSO then added to sterile, ½ strength potato dextrose agar (PDA) at either 0.5 mM final concentration or (2) 0.1 mM final concentration. ½ PDA with no added BITC was used as a control. As pictured in Figure 18, one 5 mm mycelial plug was placed on each plate, with the following fungal samples used: Fusarium oxysporum, Fusarium solani, Rhizoctonia solani, and Setophoma sp. Each fungus was replicated on 3 plates per treatment (0.5 mM BITC, 0.1 mM BITC, no BITC control), and incubated at room temperature (RT). After 48 hours, the diameter of mycelial growth from agar plugs was measured in mm. Data for each fungal set and treatment was analyzed in SAS (v.9.4) using the Waller-Duncan post-hoc test with α=0.05.
Kai choi/Lettuce Greenhouse Trials
Kai choi and lettuce greenhouse trials were performed similarly to those performed in Year 1, with the following changes: Crude extract (CE) and brown mustard (BM) treatments were not included, pots were not inoculated with Meloidogyne incognita juveniles, and all trials were transplanted 1 week after soil amendment with 2-week-old kai choi and lettuce seedlings. Additionally, percent roots colonized by Rhizoctonia solani on kai choi was evaluated similarly to Fusarium root colonization, but with the use of a R. solani selective media (Ko and Hora, 1971).
A 4×2 (amendment × leachate) factorial designed experiment was conducted to examine if activating the targeted pathogen with root leachate could improve the biofumigation effects of BITC. For the second factor, 72 hours prior to soil amendment, soil was treated with either (1) 80 mL of kai choi or lettuce root leachate per 250 g soil (indicated by a ‘+’) or (2) 80 mL of water per 250g soil (indicated by a ‘-‘) to compare results between pots treated with root leachate versus pots treated with a water control. Each leachate (+ or -) and amendment (PGS 0.5%, PGS 1%, NA, or Auto) treatment combination (PGS 1%+, PGS 1%-, etc.) was replicated 4 times.
Root leachate was prepared by placing surface cleaned kai choi or lettuce roots in a blender, adding enough water to just cover the roots, then blending on high speed until emulsified. The leachate was then diluted to 1000 mL total volume.
Cowpea Greenhouse Trial
One greenhouse pot trial was conducted to examine the effects of PGS soil amendment against the reniform nematode, Rotylenchulus reniformis. The trial was conducted in 10-cm diameter pots with 300 g sand: soil mix per pot. The sand: soil was amended with either (1) PGS at 0.5% (dry weight equivalent) (PGS 0.5%), (2) PGS at 1% (dry weight equivalent) (PGS 1%), (3) not amended (NA), or (4) not amended and autoclaved (Auto). Each treatment was replicated 4 times and treatments were arranged in complete randomized design. At the time of amendment, treatments (1) to (3) were inoculated with 200 vermiform reniform nematodes per pot. One week after amendment, three 5-day-old ‘Black Eye #5’ cowpea (Vigna unguiculata) seedlings were transferred into each pot. The experiment was terminated 2 months after inoculation. Data was collected for total biomass, overall disease incidence, and the number of female reniform nematodes present on cowpea roots.
Characterization of BITC vaporization
BITC’s vapor pressure is much lower than that of allyl isothiocyanate (AITC) which is the active biofumigant in mustard greens. Laboratory tests were conducted to determine if gaseous BITC can be generated efficiently via vaporization at 30-35C. The synthetic BITC was prepared in water containing 0.01% vs. 1% DMSO, or in soybean oil (to simulate papaya seed oil), in sealed vials. Headspace samples were analyzed for BITC using gas chromatography. Similarly, PGS placed in a sealed reaction vial was activated with water and the headspace samples were collected and analyzed using GC.
Measurement of BITC using gas chromatography
Headspace samples were drawn from the reaction vials using a syringe and quickly transferred to hexane in a GC sample vial. GC analysis of BITC was developed using an Agilent 8860 GC system equipped with a Flame Ionization detector (FID) and an autosampler model 7650A. The column used was an Equity-1 capillary GC column (30 m L× 0.32 mm ID, df 2mm). GC detector temperature was set at 300 C. Column temperature was programmed from 80 C (held for 5 min), ramping to 200 C at 10 C/min, and then to 220 (held for 10 min) at rate of 1 C/min. Hydrogen (Peak Scientific, Precision Hydrogen, 99.99999%) was used as carrier gas at a flow rate 30 mL/min. Nitrogen (Peak Scientific, Genius) was used as the makeup gas at a flow rate of 20 mL/min. Samples were injected with 2 mL with a split ratio of 10:1 and a split flow of 7.4 mL/min.
In-vitro F. oxysporum plate assays to analyze/optimize BITC transmission from PGS
Based on the agar-based plate assays developed in year-2, in which an agar plate was hollowed out in its center and water-amended PGS was used to fill the hole, additional assay plates were set up to investigate PGS application on inhibiting growth of F. oxysporum. Factors investigated include PGS amendment rates (PGS:agar ratios), PGS/water incubation time prior to fungal inoculation, water:PGS ratios (to test optimal level of water needed to activate PGS for BITC production), and solid partition to separate PGS from surrounding agar (i.e., no direct physical contact between PGS and the surrounding agar, to differentiate anti-fungal efficacy of gaseous vs dissolved state BITC produced by PGS). Another series of plate assay were set up by replacing the agar with autoclaved soil saturated with water, and by placing F. oxysporum agar plugs (cut from fully grown Fusarium plates) on top of the soil. This test was designed to determine whether BITC released from the PGS (placed at the center of the plate) can transmit through the surrounding soil to reach the fungal plug and inhibit its growth. PGS-free plates were used as control.
Preparation of PGS extracts
In year one, we tested BG extracts derived from PGS, here we prepared crude aqueous PGS extracts containing BITC. To optimize the extraction conditions, we examined effects of Tween80 surfactant concentration in the aqueous extractant, ratios of PGS/water extractant, and extraction temperature and duration.
In-vitro tests of BITC, PGS, and PGS extracts against Fusarium oxysporum in improved soil-based assays
Autoclaved fine soil (2 g) was added into a mortar, and BITC, PGS, and PGS extract, respectively, was added to the soil and ground thoroughly using a pestle. For BITC, it was tested at 10, 20, and 30 mg/L. PGS was added at 0, 20, 40 and 60 mg, respectively. Extracts were prepared from 2% and 3% PGS, respectively. To prepare the F. oxysporum mycelia suspension, 10 mL of sterile water was added to a fresh plate of Fusarium oxysporum grown on PDA, followed by sonicating the aqueous suspension for 30 s to disperse the mycelia. One mL of fungal suspension was then added to the mortar and blended with the aforementioned soil/PGS powders for 5 min. Subsequently, the resulting soil paste was transferred to a sterilized test tube and incubated at room temperature for 2 days. The content was then rinsed with 20 mL of sterile water and transferred to a 250-mL baffled flask which was shaken at room temperature for 3 h on an orbital shaker, to flush out any residual fungus. Finally, 20 mL of suspension sample was inoculated on a Fusarium selective medium plate (Komada 1976) and incubated at 28°C for several days to monitor the growth. Each treatment was duplicated.
Imaging PGS-fungus interactions in a transparent soil system
Transparent soil was made by sieving cryolite particles to obtain particles with sizes less than 40 micron, followed by suspending the particles in the refractive index matching solution of Ludox TMA (O’Callaghan et al. 2018; Sharma et al. 2020). The transparent soil was mixed with F. oxysporum mycelia (stained with calcofluor white fluorescent brightener 28) and loaded into a polydimethylsiloxane (PDMS) microfluidic chip for imaging using a confocal microscope (Leica Stellaris 5, Germany).
References cited:
Kriti Sharma, Márton Palatinszky, Georgi Nikolov, David Berry, Elizabeth A Shank (2020) Transparent soil microcosms for live-cell imaging and non-destructive stable isotope probing of soil microorganisms eLife 9:e56275; https://doi.org/10.7554/eLife.56275
Ko, W.-H. and Hora, F. K. (1971). A Selective Medium for the Quantitative Determination of Rhizoctonia solani in Soil. Phytopathology, 61(6), 707. https://doi.org/10.1094/Phyto-61-707
Komada, H. (1976) A new selective medium for isolating Fusarium from natural soil. Proceedings of the American Phytopathological Society, 3, 221.
O’Callaghan, F.E., Braga, R.A., Neilson, R. et al. New live screening of plant-nematode interactions in the rhizosphere. Sci Rep 8, 1440 (2018). https://doi.org/10.1038/s41598-017-18797-7
****************
During our final reporting period, we collected data pertaining to (1) in vitro toxicity trial of PGS against pure R. solani, (2) two greenhouse trials of PGS biofumigation on kai choi in Fusarium-infested field soil collected from a collaborator’s farm, (3) one greenhouse trial of PGS biofumigation on cowpea in sterile sand:soil mix artificially inoculated with vermiform reniform nematodes, (4) one field trial comparing PGS+CE and sorghum biofumigation on kai choi in Fusarium-infested soil on a collaborating farmer’s farm (Owen Kaneshiro Farm, Waianae, HI), (5) refined toxicity tests in soil-based assays for Fusarium sp., and (6) imaging of F. oxysporum response to PGS treatment in a transparent soil system.
In addition to the above data, we also (1) designed and validated a real-time, quantitative PCR (RT-qPCR) assay for detection of R. solani in Hawaii’s clay-like soil, (2) isolated and sequenced four Fusarium commune isolates from diseased kai choi roots with Fusarium wilt-like symptoms, and (3) designed a preliminary RT-qPCR assay for detection of F. commune in Hawaii soils.
Rhizoctonia solani qPCR development
Reference material
Pure culture mycelium DNA used in qPCR reactions was extracted using a modified CTAB method: 50-100 mg mycelium tissue was combined with and 500 uL of 2% CTAB solution (20 mM EDTA, 0.1 M Tris-HCl pH 8.0, 1.4 M NaCl, 2% CTAB, and 1% β-Mercaptoethanol) in a 1.5 mL centrifuge tube containing ceramic beads, and tissue was disrupted using a bead homogenizer. Samples were then incubated at 65°C for 30 minutes in a hot water bath, followed by centrifugation at 12,000 x g for 5 minutes. The resulting supernatant was mixed with an equal volume of phenol:chloroform:isoamylalcohol (P:C:I; 25:24:1), vortexed, and centrifuged again at 12,000 x g for 5 minutes. The supernatant was then combined with one volume of cold isopropanol, mixed by inversion, and centrifuged at 12,000 x g for 5 minutes. After discarding the supernatant, the DNA pellet was washed twice with 500 uL of cold, 70% ethanol (EtOH). The pellet was then air-dried at room temperature for 1 hour before being eluted in 50 uL of Tris-EDTA (TE) buffer solution for downstream applications. DNA extracted from soil was obtained using the E.Z.N.A. Soil DNA kit from Omega Bio-Tek (Norcross, GA, U.S.A.).
Primer/probe design
Primers RsAG2-1F (5’-ATCTCTTGGCTCTCGCATCG-3’) and RsAG2-1R (5’-AACAGGCATGCTCCAAGGAA-3’), and the flurogenic probe used for Rhizoctonia solani targeted the internal transcribed spacer 1-2 (ITS 1-2) region (DeShields, 2018). Probe RsAG2-1Pro was designed to be labeled with flurophore reporter (FAM) and Black Hole Quencher-1 (BHQ) as 5’ reporter and 3’ quencher respectively (5’-[FAM]ATCTTTGAACGCACCTTGCG[BHQ]-3’).
qPCR assays
qPCR reactions were completed using the CFX96 Touch Real-Time PCR Detection System (Bio-Rad, Hercules, CA). Reactions were run with the following program protocol: (1) denaturation at 95 °C for 15 seconds, (2) primer annealing at 60 °C for 30 seconds, and (3) repeat reaction cycle 39 times for a total of 40 cycles. Each reaction tube contained 10 µL of SsoAdvanced Universal Probes Supermix (Bio-Rad), 0.6 µL of 10 mM forward primer, 0.6 µL of 10mM reverse primer, 0.3 µL of 10 mM fluorescent probe, 6.5 µL of sterile water, and 2 µL of template DNA, for a total reaction volume of 20 µL.
Fusarium commune qPCR development
Fusarium isolate PCR amplification and sequencing
Suspected Fusarium spp. isolated from diseased kai choi roots were sent in for Sanger sequencing to the University of Hawai`i Advanced Studies in Genomics, Proteomics and Bioinformatics (ASGPB) facility, where sequencing was performed using an Applied Biosystems 3730XL DNA Analyzer (Thermo Fischer Scientific, Waltham, MA, U.S.A.). In preparation for sequencing, DNA was extracted from pure culture mycelium by smashing a small amount of mycelia in 100 uL of sterile ddH2O, then boiling the mixture for 10 minutes. Samples were then centrifuged at 13,000 rpm for 1 minute, and the supernatant was used downstream as DNA template in PCR amplification.
PCR amplification was performed using previously designed primers, FusariumEF1 (5’-ATGGGTAAGGARGACAAGAC-3’) and FusariumEF2 (5’- GGARGTACCAGTSATCATG ), for the Fusarium translation elongation factor 1a (TEF-1a) region (O’Donnell et al., 2010). Reactions were run using the following protocol: (1) denaturation at 98 °C for 10 seconds, (2) primer annealing at 57 °C for 15 seconds, (3) extension at 72 °C for 1 minute, and (3) repeat reaction cycle 34 times for a total of 35 cycles. Each reaction tube contained 10 uL of 5X Phusion High-Fidelity buffer (New England Biolabs, Ipswich, MA, U.S.A.), 2uL of 10 mM forward primer, 2 uL of 10mM reverse primer, 1 uL of 10 mM dNTPs, 0.5 uL of Phusion High-Fidelity DNA polymerase (New England Biolabs), 33.5 uL of sterile ddH2O, and 1 uL of template DNA, for a total reaction volume of 50 uL.
PCR product cleanup was performed using the ExoSAP-IT PCR Product Cleanup reagent from Thermo Fischer Scientific. Reaction tubes contained 5 uL PCR product and 2 uL ExoSAP-IT reagent for 7 uL total volume. Tubes were incubated at 37 °C for 15 minutes, followed by 80 °C for 15 minutes. The total volume of cleaned PCR product was combined with 0.5 uL of 10 mM primers and 3.5 uL ddH2O for sequencing. Sequences were aligned using Geneious (Biomatters, Inc., Boston, MA, U.S.A.) for trimming and building of a consensus sequence.
SYBR Green qPCR Primer design
Primers used were previously designed and validated for use in fluorescent dye-based qPCR, targeting the mitochondrial small subunit (mtSSU) rDNA region: FO1 (5’- GCCATAGGTCAGATAACCAGTT-3’) and FO2 (5’- TCACTACTGGTGTCAGAAACGG-3’) (Zhu et al., 2016).
qPCR assays
qPCR reactions were completed using the CFX96 Touch Real-Time PCR Detection System (Bio-Rad, Hercules, CA). Reactions were run with the following steps: (1) denaturation at 95 °C for 15 seconds, (2) primer annealing at 58 °C for 20 seconds, and (3) extension at 72 °C for 20s, then 77 °C for 5s, and (5) repeating the reaction cycle 39 times for a total of 40 cycles. Melting curve data was obtained after reaction runs by cooling to 65 °C for 5s and heating to 95 °C at 0.5 °C increments, with collection of fluorescent data. Each reaction tube contained 12.5 µL of SsoAdvanced Universal SYBR Green Supermix (Bio-Rad), 3.25 µL of 0.1 mM forward primer, 3.25 µL of 0.1 mM reverse primer and 1 µL of template DNA, for a total reaction volume of 20 µL.
Kai choi (+/- surfactant) greenhouse experiment
One kai choi greenhouse experiment completed in the final reporting period was performed similarly to kai choi and lettuce greenhouse trials from year three. However, instead of leachate, pots were either treated with 80 mL of 0.1% Tween80 solution or 80 mL water during the amendment stage.
Sorghum and PGS+CE kai choi field experiment
Sorghum was planted on July 23, 2023, at a rate of 50 lbs/acre in 6 × 13 sq. ft. Experimental plots were arranged in a randomized complete block design (RCBD) with four replications. Initial soil samples were collected on July 26, 2023. Sorghum was terminated on September 25, 2023, followed by soil sampling on September 28, 2023. Kai Choi was then planted on October 18, 2023. Papaya crude extract was prepared by boiling papaya seed grounds in a solution of 0.1% Tween80 for 30 minutes. Papaya extract was applied on October 19, 2023. Final soil samples were collected on November 6, 2023, for analysis and comparison with initial samples. The disease rating was conducted using a scale ranging from 0 to 4. On this scale, a rating of 0 indicates plants that are completely healthy, showing no signs of disease. Rating from 1-4 indicates increasingly severe symptoms of wilting and disease in the plants. Fresh plant weight was also measured at the end of the experiment.
Preparation of alginate hydrogel-based transparent soil
Alginate hydrogel particles were prepared by emulsification coupled with external gelation. First, 1% alginate aqueous solution was mixed with soybean oil containing 3% SPAN 80 and emulsified for 1 min. The emulsion was sonicated for 10 min in an ice bath, and calcium chloride solution was added dropwise to crosslink the alginate gel particles. Afterwards, the gel particle suspension was centrifuged to remove the supernatant, followed by rinsing the particles with isopropanol to remove oil residues. The alginate hydrogel particles were then suspended in water and used as a transparent soil model (Ma et al., 2019).
Imaging fungal response to PGS treatment in transparent soil using a new procedure with improved microfluidic chambers
A two-layer polydimethylsiloxane (PDMS) microfluidic chamber was fabricated using 3D printed molds and bonded to a glass slide via plasma treatment. This microchamber allows improved direct imaging of fungal-PGS interactions in transparent soil with a confocal microscope. Fusarium oxysporum hyphae were mixed with hydrogel transparent soil (as an aqueous suspension) with or without amended PGS and the mixture was injected into the microchamber through the microfluidic channels. The system was incubated for one day to allow the PGS biofumigation to take effect. This was followed by replacing water in the microchamber with the liquid Komada medium via perfusion, and the fungal cells were allowed to grow for 2 days, and subsequently subject to in-situ fluorescent staining and de-staining prior to confocal microscopy imaging.
References cited:
DeShields, J.B., & Kiwamu, T. (2018). Rhizoctonia solani AG2-1 primers and probe. Unpublished work.
Ma, L., Shi, Y., Siemianowski, O., Yuan, B., Egner, T.K., Mirnezami, S.V., et al. (2019). Hydrogel-based transparent soils for root phenotyping in vivo. Proceedings of the National Academy of Sciences 116(22), 11063-11068. doi: doi:10.1073/pnas.1820334116.
O’Donnell, K., Sutton, D. A., Rinaldi, M. G., Sarver, B. A. J., Balajee, S. A., Schroers, H.-J., Summerbell, R. C., Robert, V. A. R. G., Crous, P. W., Zhang, N., Aoki, T., Jung, K., Park, J., Lee, Y.-H., Kang, S., Park, B., & Geiser, D. M. (2010). Internet-Accessible DNA Sequence Database for Identifying Fusaria from Human and Animal Infections. Journal of Clinical Microbiology, 48(10), 3708–3718. https://doi.org/10.1128/JCM.00989-10
Zhu, Z.X., Zheng, L., Hsiang, T., Yang, G.L., Zhao, D.L., Lv, B., Chen, Y.F. and Huang, J.B., (2016). Detection and quantification of Fusarium commune in host tissue and infested soil using real‐time PCR. Plant Pathology, 65(2), pp.218-226.
This project started in summer 2020. During this first report period, due to the COVID restrictions and financial challenges facing our farmer collaborators as a result of COVID, we are unable to initiate the proposed field trials on participating farmers’ farms. However, we are able to work with one of our collaborating farmers, Mr. Owen Kaneshiro of the Owen Kaneshiro Farm, Waianae, HI. Using soil collected from various fields on the Kaneshiro farm (picture 1), and through discussions with our collaborating farmer partner, we designed and conducted greenhouse studies to determine the efficacy of the papaya seed biofumigant against Fusarium oxysporum f. sp. letucae and root-knot nematode, Meloidogyne incognita, under different biofumigant formulations and application regimes, using lettuce and kai choi as test crops. Laboratory studies have also been conducted to optimize the preparation of the PGS as biofumigants, and to investigate the impact of PGS application on soil bacterial/archaeal communities.
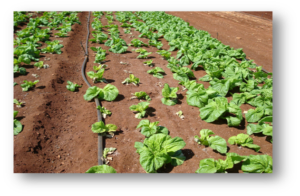
Papaya seeds contain a high level of BG that can be enzymatically hydrolyzed via myrosinase in aqueous solution to form BITC which has potent pesticide activities against a range of soil-borne phytopathogenic nematodes, insect pests, and fungi. The binary glucosinolate/myrosinase system requires activation by disrupting the cellular compartments that naturally separate the glucosinote from the myrosinase, and by the inclusion of water to trigger the enzymatic hydrolysis. In our previous study (Han et al., 2018) we noted a significantly elevated level of BITC in the oil extracted from papaya seeds that were previously frozen compared with that found in oil extracted from non-frozen seeds. Since BITC is the active ingredient of the papaya seed biofumigant, we tested whether it was necessary to pre-freeze the seeds to obtain high BITC in the PGS. To that end, prior to the greenhouse pot trials, we investigated processing conditions for preparing the PGS and measured BG and BITC contents in the PGS. The factors examined included (i) freezing seeds prior to drying and grinding; (ii) seed drying temperatures; and (iii) seed drying duration. One group of fresh seeds were frozen at -20°C and then dried at 50°C for 48 h, before being ground in an electric grain mill grinder (S1). A second group of papaya seeds were dried at 50C for 48 h without a prior frozen treatment (S2), and a third group of seeds were oven dried at 40°C for 24 h without a prior frozen treatment (S3). The resulting PGS samples were reacted with an equal weight of water at room temperature for 6h to synthesize BITC, and seeds oil containing BITC was finally extracted with hexane and analyzed using the cyclocondensation assay and HPLC quantification as described in the Materials/Methods section. The results are shown in Fig. 1. Samples S1 and S2 gave essentially the same amount of BITC after activated with water, indicating freezing of papaya seeds prior to drying and grinding into PGS is unnecessary. BITC is lower in sample S3 than the other two treatment groups (p< 0.05). Drying the seeds for 24 hours at 40°C did not remove sufficient moisture from the seeds, and the resulting PGS formed small clumps which may have reduced the enzymatic conversion of BG to BITC. Overall, our results indicate that directly drying the papaya seeds at 50°C for 48 hrs without prior freezing is adequate to generate PGS with reproducible BITC content. Further, activation of PGS with equal volume of water for 6 hours at room temperature is sufficient to convert most of the BG to BITC.
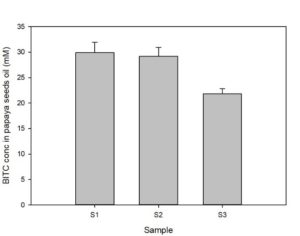
We also measured the BG and BITC contents in seeds of two common papaya varieties Rainbow and Sunrise, and tested whether seed BITC synthesis is affected by dry storage. The BG content was averaged about 20-25 µmole/g dry seeds regardless the papaya varieties. As shown in Figure 2, the BITC generation did not vary considerably between the two varieties. These results also demonstrate that BITC generation from seeds freshly harvested from fruits is similar to that from seeds dried and stored in an air-tight Ziploc bag for 1 month.
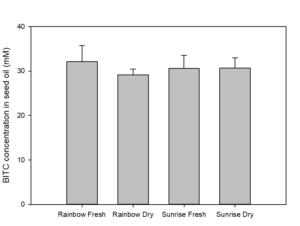
Based upon these results, PGS for subsequent greenhouse trials was prepared by drying papaya seeds at 50°C for 2 days, milled into powders, and stored in Ziploc bags at room temperature prior to use in the trials. We also analyzed the nutrient profile of the defatted PGS. The proximate analysis result is presented in Table 1.
Table 1. Nutrient profile of defatted PGS.
In greenhouse Trial I, phytotoxicity of lettuce seedlings occurred in all treatments receiving PGS and resulted in plant growth similar to the NA (Picture 2; Fig. 3). However, BM was able to suppress M. incognita and resulted in lettuce growth similar to the autoclaved treatment (without M. incognita inoculation and no fungal infestation) (Fig. 3).
In Trial II, PGS was amended to the soil one week prior to the seedling transplanting. While the canopy width of kai choi was increased by BM compared to the NA control, the shoot and root weights of kai choi in BM amended soil were not different from NA control (Fig 4 B, C). All PGS treatments (PGS 0.5%, PGC 1%, PGC+CE) did not pose phytotoxicity on kai choi as the canopy width, shoot and root weight were all not different from the NA control (Fig. 4 A, B, C). Unfortunately, all PGS treatments and brown mustard (BM) amendment did not reduce the plant disease index using a scale of 1-4 (Fig. 4 D). However, all biofumigation (PGS and BM) significantly (P ≤ 0.05) reduced the number of root pieces infected by F. oxysporum (Fig. 4 E) and the number of M. incognita penetrated into kai choi roots (Fig. 4 F). No Rhizoctonia hyphae was observed on the Komada medium. Initial attempts to monitor the fate of BITC in the PGS-amended soil during the trial produced highly varied results and no clear conclusion can yet be drawn. Uniformity in soil sampling is identified as a potential factor that needs further investigation. It is also noted that PGS has a relatively high oil content (about 25% per dry seed weight), while BITC is hydrophobic, and hence BITC is likely partitioning to the oil phase, and its release may be affected by gradual seed oil biodegradation in the soil over time. These issues will be further investigated to achieve a more effective PGS application regime as a soil biofumigant.
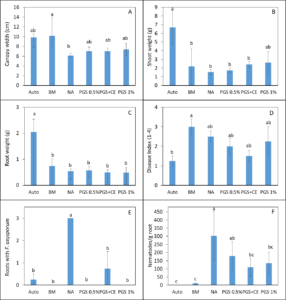
Trial III was collected from another lettuce field with a history of Fusarium wilt infestation. Same treatments as Trial II were imposed and 3 Manoa lettuce seedlings were planted into each pot for bioassay over 1 month. Lettuce plants responded with positive growth from the NA control when planted in soil amended with BM or 1% PGS (P ≤ 0.05, Fig. 5 A). Only BM resulted in wider canopy width and total shoot biomass higher than NA (Fig. 5 B, C). It is also promising in this trial that biofumigation treatments resulted in a lower plant disease index compared to the NA control (P ≤ 0.05; Fig. 5D). Deviate from the results in Trial II, PGS 0.5% and PGS 1% were the only treatments that suppressed the recovery of kai choi roots with F. oxysporum on the Komada medium (P ≤ 0.05, Fig 5E) much like the autoclaved soil. Whereas, root gall index (1-5 scale) on lettuce was significantly suppressed by all biofumigation treatment including PGS+CE compared to the NA control (P ≤ 0.05, Fig 5F). From this trial, PGS showed promise as a potential sustainable biofumigant.
To study the effect of PGS treatment on soil microbial diversity, twelve soil samples, triplicate samples from four different treatments (Table 2), were collected from greenhouse Trial 1 and then subjected to 16S rRNA gene amplicon sequencing. Bioinformatics analysis identified a total of 510 bacterial/archaeal genera across the twelve samples. The 40 most abundant genera, which were those operational taxonomic units (OTUs) with relative abundance no less than 1% in at least one sample, were summarized in Figure 6.
Table 2. Sample ID and treatment conditions for twelve samples subjected to sequencing
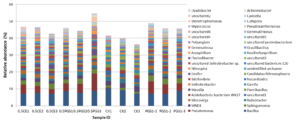
Analysis of the alpha diversity, including the observed OTUs and Shannon index, were shown in Figure 7A and 7B, respectively. No significant difference among the four treatments were observed with all p-value of Kruskal-Wallis tests higher than 0.1.
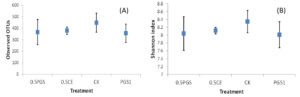
Analysis of beta diversity, including the Jaccard distance and unweighted UniFrac distance, were shown in Figure 8A and 8B, respectively. Different treatments showed significant microbial community dissimilarity, with p-value of 0.021 in PERMANOVA test of unweighted UniFrac distance. Additionally, all the three treatment (0.5 CE, 0.5 PGS and PGS1) showed significant microbial community dissimilarity than the control treatment (CK), with p-value of 0.10, 0.09 and 0.10 in pair-wise PERMANOVA test, respectively.
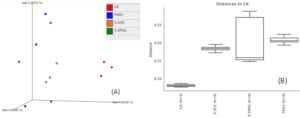
Based on the taxonomic classification of 16S rRNA gene, eight previously-reported plant growth promoting bacteria (Genus/Species) were identified in the soil samples, shown in Figure 9. Different treatments resulted in significant difference in the abundance of plant growth promoting bacteria (ANOVA test, p=0.022), shown in Figure 10. All the PGS treatments (0.5 CE, 0.5 PGS and PGS1) showed significant higher total relative abundances of the eight plant growth promoting bacteria than that in the control treatment (CK), with p-value of 0.002, 0.054 and 0.013 in pair-wise ANOVA test, respectively.
References cited:
Han, Z., Park, A., Su, W.W. 2018. Valorization of papaya fruit waste through low-cost fractionation and microbial conversion of both juice and seed lipids. RSC Advances, 8(49), 27963-27972.
**********
During our second year we collected data pertaining to (1) a field trial of PGS on kai choi in Fusarium-infested soil on a collaborating farmer’s farm (Owen Kaneshiro Farm, Waianae, HI), (2) in-vitro toxicity tests of PGS towards various fungal pathogens, and (3) laboratory studies of biofumigant (BITC) emission from PGS to develop better practices for more efficient use of PGS in the field implementation of PGS-based biofumigation.
Field Trial
A. Biomass and Marketable Yield
Total biomass and marketable yield were measured to determine overall effectiveness of the PGS treatments at preserving the crop for market and maintaining healthy plant growth (Picture 4). All treatments were significantly less in mass for both the total biomass and marketable yield than the conventional Vapam-treated plots within the experiment. However, this included the mustard treatment, which showed no significant difference from the PGS treatments as well (Fig. 11). Considering mustard was an effective biofumigation crop (Waisen et al., 2019) previously tested in the same farm and the positive effects of PGS in our greenhouse pot trials, the current field results suggested that trench application was not an effective approach to deliver PGS or macerated brown mustard.
B. Disease Incidence
Diseased plants were counted to determine the overall effectiveness of PGS and other tested amendments in reducing Fusarium wilt disease incidence in a field infested with F. oxysporum (Picture 5). Of the six treatments, Vapam had the least amount of disease, significantly lower than all other treatments. Both PGS 0.5% and PGS 1% were significantly higher in disease incidence, but comparable to the brown mustard treated plots (Fig. 12). The Sorghum planted plots (not till into the soil) had less diseased than the PGS-treated plots, but not different from the control and mustard. These results suggested that both trench application of PGS and macerated brown mustard and no-till cover cropping of sorghum were not effective biofumigation approaches against the targeted soil-borne pathogens. Future study will examine more effective and feasible biofumigation methods using PGS.
C. Fusarium colonization of kai choi roots
To observe whether or not PGS was able to suppress F. oxysporum and prevent root colonization, kai choi roots collected from the field experiment were sterilized and plated on Fusarium-selective media, then observed for any Fusarium-like mycelial growth protruding from the plated roots (Picture 6).
Control, mustard, Vapam, and 1% PGS treatment groups were all significantly lower in Fusarium growth than the 0.5% PGS and Sorghum treatment groups (Fig. 13). Most surprisingly, there is very little difference between the control (negative) treatment group and the Vapam (positive) treatment group. This is likely due to certain strains of F. oxysporum being known to colonize plant roots as avirulent endophytes in various plants, so we can’t be sure if what emerged from roots on the Komada media are pathogenic or avirulent, symbiotic F. oxysporum strains. In order to verify findings or produce more accurate results, we will develop a qPCR assay specific to the F. oxysporum forma specialis that is pathogenic in kai choi.
Picture 6. Surface-sterilized kai choi root pieces plated on Fusarium-selective Komada media (left) and same kai choi root pieces on Komada media with suspected Fusarium growth (right).
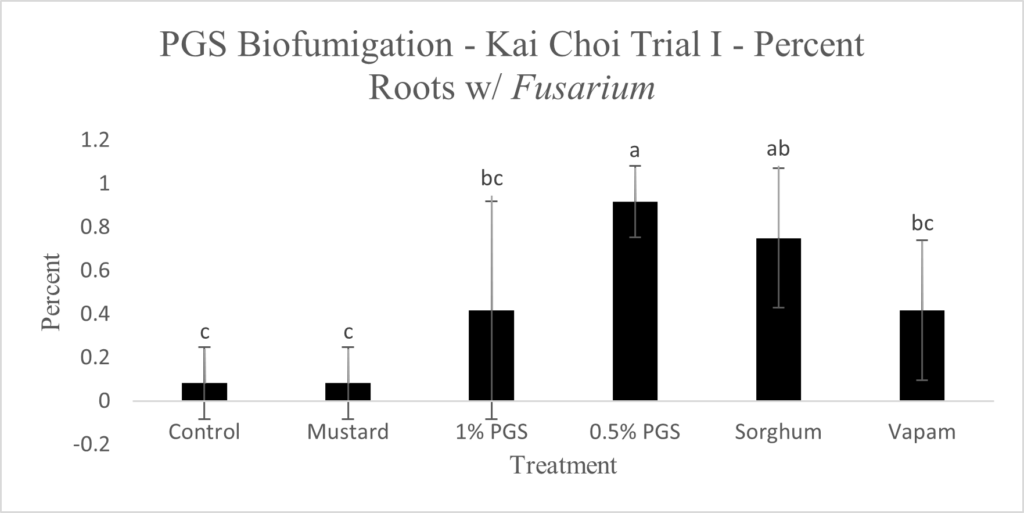
D. Nematode community as soil health indicators
A primary reason for assessing alternative biofumigant methods is due to the harm that Vapam inflicts on the natural soil ecosystem when used. To make this comparison and measure the soil health of PGS-treated plots versus Vapam-treated plots, free-living nematodes were extracted and counted. Total free-living nematode counts were significantly higher in the Sorghum-treated plots compared to all other treated plots. The control, mustard, 1% PGS, 0.5% PGS, and Vapam treated plots were all comparable in overall nematode counts (Fig. 14).
Most treatment groups were comparable in species richness (number of different species present) measures, however the Vapam plots were significantly less species rich (Fig. 15). This is likely due to the that Vapam indiscriminately kills everything in the soil that it is used in (nematodes, fungi, beneficial microbes, etc.), thus creating a uniformity in the species present in the soil as the species that are able to bounce back quickly. It’s unlikely in these conditions to observe and record and long generational/large nematodes in the soil samples. So, despite not seeing a large difference among the PGS, sorghum, mustard, and control treated plots, there is already an observable increase in species richness in the soil not treated with Vapam. This is an indicator that in one crop rotation without the synthetic fumigant, the soil is becoming healthier.
PGS biofumigation plate assay
In our initial plate assay design, fungal cultures were inoculated onto the PGS-amended agar plates (PGS was blended in and encapsulated in the agar) and incubated for subsequent colony count. We encountered microbial contamination problems with this assay format. Some of the papaya seed indigenous microbes apparently survived the seed drying and milling processes and grew in the PGS-amended agar plates. Subsequently, we developed an alternative plate assay, in which the agar plate was hollowed out in its center and water-amended PGS was used to fill the hole. As a PGS-free control, the hole was either not filled in with the PGS, or without creating the center hole. This assay format worked well, showing little signs of contamination from the indigenous papaya seed microbes, and was adopted for subsequent in-vitro bioassays. Of the three fungi tested, the F. oxysporum plate assay showed greatest overall significant difference in growth, reducing the mycelial growth to almost zero compared to the control group (Picture 7, top left; Fig.16). Thus, it appears that PGS biofumigation is highly effective against F. oxysporum in vitro. However, both F. solani and Setophoma sp. had no significant difference in growth between the control and two treatments groups, with the PGS biofumigation appearing to have no effect on the mycelial growth (Picture 7, top right and bottom; Fig. 17). For Setophoma sp., there was a slight difference between the control group and the group treated with 0.5g PGS + 0.5g water, but this was not easily observed (Picture 7, bottom; Fig. 17).
Emission of BITC from PGS
In intact papaya seeds, cellular membranes separate glucosinolate (the substrate) from myrosinase (the enzyme), and hence efficient breakdown of the membrane barriers is essential to promote the enzymatic hydrolysis upon water addition. One of the simplest approach to break down the membranes is to mill the dried seeds into powders, called “PGS” herein. This method is inexpensive, though it may not completely degrade all membrane barriers in the seeds. In our prior study (Han et al. 2018), we could detect ample amounts of BITC after extracting water-activated PGS using organic solvents. The result indicated that high levels of BITC could indeed be produced by PGS upon water activation. However, since organic solvents were used to extract the BITC, we do not know the extent BITC can be released by itself from the PGS without extraction. In year 2, we designed and evaluated a series of laboratory experimental tests, to gain a better understanding about the temporal pattern and extent of BITC emission from water-activated PGS, and to investigate how this process is affected by PGS water content, PGS particle size, freezing pretreatment, and inclusion of soil. These fundamental characterizations are essential to develop better practices for more efficient implementation of PGS-based biofumigation in the field. Because currently we do not have a gas chromatography system for measure BITC in the vapor phase, we had to develop an alternative assay platform based on measurement using liquid chromatography. In this regard, since BITC is a hydrophobic compound that shows good solubility in plant oil (triacylglycerol) and mineral oil (alkanes), we tested commercial soybean oil and mineral oil as a liquid trap for BITC emitted from PGS, and the oil was then analyzed for BITC using HPLC. The transport of BITC from water-activated PGS to the vapor phase surrounding the PGS involves multiple steps. First, the addition of water to the PGS activates the myrosinase enzyme which catalyzes the hydrolysis of benzyl glucosinolate (BG) in an aqueous phase. Second, the BITC thus generated is hydrophobic, and hence it tends to partition into the seed fat, or emit to the vapor phase (interstitial air space) followed by eventual release into the headspace above the PGS bed. It may also be absorbed onto PGS particle surfaces as it diffuses through the bed of PGS.
In our tests, we typically use 2 g of dried PGS in each test. We first examined the BITC trapping efficiency by soybean oil vs mineral oil. A water:PGS ratio of 3 was used so that a thin layer of water is viable above the PGS bed which was overlaid with 2 mL of oil. The tube was incubated under room temperature for 40 hours before the oil samples were taken and analyzed using HPLC. As shown in Fig. 18, mineral oil appeared to be a more effective BITC trap than the soybean oil. More importantly, this result provided direct proof that not only BITC can be generated by PGS upon water activation, but also it can be released out of the PGS matrix and migrate into the headspace. Next, we investigated the temporal pattern of BITC release from PGS. Using mineral oil as the trap, and with 2 grams of PGS plus 6 grams of water, the BITC continued to partition into the oil trap over the 32-hr testing period, as shown in Figure 19. We also investigated the effect of soil on BITC release. Sorption of released BITC onto soil surfaces could potentially reduce the efficacy of BITC. In one treatment, 2 g of PGS was mixed into 4 g of soil and placed in a 50 mL centrifuge tube, followed by adding sufficient water until a thin layer of water was viable above the soil/PGS bed. In the other treatment, 2 g of PGS was placed in the bottom of a 50-mL centrifuge tube, overlaid with 4 g of soil, followed by adding water until a thin layer of water was viable above the soil/PGS bed. In both treatments, 2 mL of mineral oil was overlaid on the water layer. As shown in Figure 20, by dispersing PGS in soil, much higher BITC emission was detected, which could have implications on how to apply the PGS in soil in the field. Because in these tests, the interstitial space in the PGS bed was filled with water, BITC needs to migrate through this aqueous phase before it can partition into the oil trap on the top. Typically, PGS-amended soil in the field is not waterlogged, the hydrophobic BITC is likely to diffuse more readily in the air space between soil particles and hence greater and faster BITC release is expected in the field. In summary, our results provide direct proofs of BITC release from water-activated PGS, and that the release process likely lasted for several days. The vapor pressure of BITC is two orders lower than that of allyl-isothiocyanate (AITC, active biofumigant in mustard), and hence slower release than AITC is expected.
Water plays an important role in the conversion of BG to BITC in PGS. On the one hand, water is necessary for the myrosinase to function. However, too much water may rinse out BG (which is water soluble), and may cause BITC degradation. Too much water is also harmful to the crops. We examined BITC release at four different water:PGS ratios (0.5, 1, 2, and 3) using the oil-trap assay. At a 2:1 water:PGS ratio, a higher BITC production was noted (36.05±12.65 mM) compared to our initial treatment (3:1 water:PGS; 22.51±0.43 mM). At further reduced water:PGS ratios of 1 and 0.5, the BITC production was even higher (120.14±1.80 mM and 108.58±36.71 mM, respectively). However, we likely cannot judge the water effect solely on these data. As the water content was decreased, oil on the top seeped into the PGS bed after several hours of incubation. Therefore, some of the oil was in direct contact with the PGS matrix, and BITC likely partitioned into this oil phase once it was produced from BG hydrolysis. This result however suggests addition of some oil (such as vegetable or mineral oil) to the PGS when it is amended to the soil might be beneficial in modulating the release characteristics of BITC. Partition of BITC in an oil phase can help stabilizing the hydrophobic biofumigant and prolong its release from the PGS. This aspect will be investigated further during the next report period.
To investigate two additional potentially important factors, i.e., PGS particle size and seed freezing, we slightly modified the assay. We blended 2 g PGS with 2 g of water and 2 g of mineral oil in a 50 mL centrifuge tube and incubated for 18 hours. The tube was then centrifuged, and the oil sampled for BITC measurement. As shown in Figure 21, PGS with finer particle size generated more BITC than coarser PGS. Freezing treatment of papaya seeds prior to drying and grinding into PGS also improved BITC release from PGS (Figure 22). Finer PGS particles likely promoted contact between BG and myrosinase by breaking down tissue/membrane barriers in the papaya seeds and also increased interfacial area for better mass transport. By freezing fresh seeds, further degradation of internal tissues in papaya seeds may occur which can augment breakdown of cellular membrane barriers and promote BITC production.
References cited:
Waisen, P., B. S. Sipes, and K.-H. Wang. 2019. Potential of biofumigant cover crops as open-end trap crops against root-knot and reniform nematodes. Nematropica 49:254-264.
***********************
During our third year, we collected data pertaining to (1) in vitro toxicity trials of pure synthetic BITC against various soil-borne fungal pathogens in agar plate assays, (2) in vitro toxicity study of pure BITC, PGS, and PGS extracts against Fusarium fungal pathogen in soil, (3) characterization of gaseous vs dissolved state BITC against Fusarium fungal pathogen, (4) development of a novel assay for live imaging of fungal interactions with PGS in transparent soil, (5) greenhouse trials of PGS biofumigation on kai choi and lettuce in Fusarium-infested field soil collected from a collaborator’s farm, and (6) a greenhouse trial of PGS biofumigation on cowpea in a sterile sand: soil mix artificially inoculated with vermiform reniform nematodes.
BITC in vitro agar plate assays
In order to confirm that BITC released from PGS was the compound involved in suppressing plant-pathogens of interest, synthetic pure BITC was combined with ½ PDA media for two of in vitro experiments with pure fungal isolates. This eliminated any interference of additional microbes or compounds present in the PGS. In both in vitro trials, all four fungal species (F. oxysporum, F. solani, R. solani, and Setophoma sp.) showed significant reduction in growth in both 0.1 mM and 0.5 mM BITC concentration treatments compared to the control (Fig. 23 and 24). Additionally, 0.5 mM BITC plates had significantly lower mycelial growth than the 0.1 mM BITC plates (Fig. 24), with growth completely halted in Trial 1 (Fig. 24A). These results demonstrate that pure BITC is able to suppress a range of fungal pathogens when combined in vitro in agar media, however the level of suppression in this bioassay is known only up to the 48hour time point. Subsequently, we conducted further in vitro testing using an alternative soil-based assay for F. oxysporum, examining an extended range of BITC concentrations and growth periods. The results are presented in the section “In vitro soil-based assays.”
In vitro soil-based assays
Agar-based in vitro plate bioassays were predominantly used in our study to probe the inhibitory effect of BITC on soil-borne fungal pathogens. While it is a convenient assay platform, it does have limitations. Agar is a hydrophilic material, whereas soil surface properties are quite different. It is known that soil biofumigant may be adsorbed onto soil surfaces as it is transmitted through the soil matrix. Therefore, antifungal efficacy revealed in agar-based assays may not completely represent the actual biofumigant performance in soil. We evaluated several alternative in vitro assay formats in which autoclaved field soil (collected from the site the first field trial was conducted) was incorporated. Initially, our approach simply replaced the agar with water-saturated soil, in otherwise the same assay format as the agar/PGS plate assay described above. By placing fungal agar plugs above the soil, we wanted to compare mycelia growth on the plug by varying the PGS amendment rates. In the agar assay, BITC was clearly released from the PGS placed in the center of the agar plate and diffused through the agar to reach the inoculated fungus and inhibit its growth. Unfortunately, we were unable to get consistent results with the soil-based plate assay. Subsequently, we developed an alternative assay format that offered a simple and reproducible way to semi-quantitatively evaluate the effect of PGS, PGS extracts, and BITC, in inhibiting F. oxysporum mycelial growth in soil. We also invested surfactant treatment of soil on the biofumigant activity. The alternative assay is described in the Research Method section above, under “In-vitro tests of BITC, PGS, and PGS extracts against Fusarium oxysporum in improved soil-based assays.” First, we tested the PGS amendment rates at 0, 1, 2, and 3%, respectively. From Figure 25, significant growth inhibition of F. oxysporum mycelia can be seen at 2% and 3% PGS amendments into the soil. In a typical field soil environment, unlike the selective media used here, nutrients are much scarcer, and hence the extent of fungal inhibition offered by 2% PGS is likely sufficient to suppress the growth of any residual F. oxysporum cells that are not killed by the BITC released by PGS mixed into the soil. The brown patches appeared on the selective agar plates in Figure 25 were from the soil used in the assay. While a very simple assay, it offered consistent and reproducible results. Therefore, we used this assay format to further test the effect of pure BITC and PGS extracts, and soil treatment with a surfactant called Forte (J R Simplot Company, Lathrop, CA). The commercial soil surfactant Forte can alter soil surface properties by alleviating soil water repellency, and possibly reduce soil adsorption of hydrophobic BITC released from PGS. As shown in Figures 26 and 27, respectively, BITC and PGS extracts both performed better with Forte-treated soil than with untreated soil. The effect is especially profound with PGS extracts (Figure 27). Using the improved soil-based assay, pure BITC was shown to be highly effective at 30 mg/L which is equivalent to about 0.2 mM (Figure 26). This is slightly higher than the 0.1 mM BITC revealed from the agar-based assay, although with 0.1 mM BITC, fungal suppression was also not so effective in one of the two trails as shown in Figure 24B. Surprisingly, PGS extracts performed worse than PGS at 2% and 3%, using untreated soil (cf. Figure 25 vs. Figure 27, day 2), although PGS extract performance was improved considerably when used with Forte-treated soil (Figure 27). In future experiments, we will improve the preparation of the initial mycelia suspension for inoculation to enable quantification of fungal growth upon PGS treatments. We will also apply this assay to examine additional soil-borne fungal pathogens, besides F. oxysporum.
Kai choi greenhouse biofumgation trial
A. Disease Index
Overall disease index was assessed based on a pre-determined kai choi disease scale of 1 to 4, with 1 being healthy and 4 being most diseased (Fig. 28). In the kai choi greenhouse trial, the treatments with the highest disease index ratings were the non-amended (NA) treatment group (both with [+] and without [-] leachate were comparable) and the autoclaved soil (Auto) treatment group, which was comparable to NA (+ and -) (Fig. 29B). As the disease index rating does not discriminate between biotic and abiotic disease symptoms, the high rate of disease in the Auto treatment group was likely due to a lack of nutrients in the autoclaved field soil. To reduce abiotic disease symptoms in autoclaved reps, synthetic fertilizer will be included in the treatment moving forward.
The 0.5% PGS (+) and (-) and the 1% PGS (+) treatments were comparable to each other, however these 3 treatments showed overall reduced disease symptoms when compared to all NA disease index ratings. Additionally, while 1% PGS (-) results were comparable to the NA (+) treatment results, the disease index rating in 1% PGS (-) was significantly less than the disease index rating observed in the NA (-) treatment group (Fig. 29B). Overall, both the 0.5% and 1% PGS amendment groups significantly reduced disease incidence regardless of root leachate drenching used or not. There was also no significant difference in disease incidence between the two PGS concentrations, indicating that the 0.5% PGS amendment rate may be sufficient in trials moving forward. However, additional kai choi greenhouse trials are needed to confirm this hypothesis.
B. Biomass
Overall biomass of combined kai choi shoots and roots was measured in grams (g). Auto treated pots had the lowest biomass, which matches up with the respective disease index ratings. Similar to results seen with the disease ratings, individual PGS treatments were not significantly affected by the use of root leachate or not [i.e. 0.5% PGS (+) did not differ from 0.5% PGS (-) and 1% PGS (+) did not differ from 1% PGS (-)]. However, contrasting with the disease index results, the 1% PGS amendment rate outperformed the 0.5% PGS rate in overall biomass. While the two concentrations were comparable to each other, the 1% PGS treatment had significantly higher mean biomass than the NA treatment group, whereas the 0.5% PGS biomass was comparable to that of the NA treatment group (Fig. 29A).
Interestingly, in terms of biomass, the NA (+) group performed significantly better than the NA (-) group, indicating potential benefits of adding the kai choi root leachate alone (Fig. 29A). As kai choi is a member of the Brassica spp. (Brassica juncea subsp. integrifolia), a genus known for volatile-producing plant species, it is possible that the root leachate contains additional volatiles or compounds toxic to Fusarium spp. and R. solani.
C. Fusarium and Rhizoctonia root colonization
Kai choi root pieces plated on Komada (Fusarium) and KH (Rhizoctonia) selective media were observed periodically over 12 days to check for Fusarium spp. and R. solani mycelial growth. There was a small amount of R. solani growth observed in the Auto treated reps, but due to some Rhizoctonia existing as saprophytic species within plant roots, there is potential for non-pathogenic Rhizoctonia spp. to emerge on the selective media. Otherwise, in this trial, no statistically significant differences between treatments were observed (Fig. 29C-D). This is likely due to a small data set size (4 reps per treatment, with only 3 root pieces plated per rep), so any major variability within treatments may have a larger effect on results. Based on this, no clear conclusions can be derived from root colonization data. For future trials, root pieces will be plated onto selective media per plant growing in each potted rep. Additionally, qPCR assays will be designed and used to quantify levels of Fusarium and Rhizoctonia in the kai choi roots and soil.
Cowpea greenhouse biofumigation trial
A. Disease Index
Disease index was measured based on a pre-determined cowpea disease scale of 1 to 4 (Fig. 30). Based on this scale, the Auto treatment was found to have the greatest overall disease incidence, whereas NA treated pots had a significantly lower mean disease rating compared to the Auto and PGS treatments, which was the opposite of expected results (Fig. 31A).
B. Root and shoot biomass
Cowpea root and shoot biomass was measured separately in grams (g). The NA treated group had significantly greater biomass in both the shoots and roots of the plants, whereas the 1% PGS treatment was significantly the lowest in shoot and root mass, aside from the Auto treatment in shoot mass, in which it was comparable (Fig. 31B-C). These results align with those observed in the cowpea disease index data.
There were confounding factors in this trial. Treatments planted in plastic pots appeared to retain excess moisture, leading to plant roots rotting early in the trial, a lack of uptake in water and nutrients, and premature plant death due to causes unrelated to nematode inoculation and infection. Treatments planted in terracotta pots did not have this issue. For future trials, only terracotta type pots will be used. Additionally, as the sand: soil mixture is autoclaved and without added nutrients in the Auto and NA treatment groups, these pots will be treated with a synthetic NPK fertilizer moving forward to reduce the lack of nutrients as a potential cause of plant death.
C. Number of female reniform nematodes infecting roots
The number of R. reniformis females present per 1 g of roots were counted under a dissecting microscope after acid fusion staining. Infection observed overall was highly variable, with a low number of females quantified in the healthier, NA treatment reps. While it appears that the 1% PGS amended pots had a much higher number of female nematodes present, it was still found to be statistically comparable to the NA treatment (Fig. 31D). This is likely due to large outliers within the 1% PGS data set. Despite outliers, there was generally low infection of roots by reniform females. With questionable health of the plants, it is unclear if infection was low due to bad (rotting) roots or poor transfer of nematodes to the pots during inoculation.
Lettuce greenhouse biofumigation trial
Overall results obtained from this trial displayed no significant interaction between pots that were treated with lettuce root leachate, versus those that were not. All lettuce reps for each treatment group were therefore pooled (n=8) regardless of leachate treatment or not.
A. Disease Index
Disease was measured based on a pre-determined lettuce disease scale of 1 to 4 (Fig. 32). No significant difference was observed between treatments, with the NA treated group having a slightly higher disease rating. However, in general the disease index remained at a relatively constant mid-range across treatments (between 2-2.5 average) (Fig. 33A).
B. Biomass
Lettuce root and shoot mass were measured separately in grams (g). Root biomass was significantly higher in the 0.5% PGS amended reps compared to the NA treatment, however 1% PGS treated reps had significantly lower root mass than NA (Fig. 33C). Similar results were seen in the shoot biomass means, where the 0.5% PGS treatment had had significantly higher biomass, but the 1% PGS treated pots were comparable to the NA treatment (Fig. 33B). Based on these results, rather than being related to disease or lack thereof causing differences in biomass, it is speculated that PGS has a green manure effect at lower concentrations, but phytotoxic effects if PGS concentrations used for amendment are too high. This is supported by the positive growth results seen in the 0.5% PGS data compared to the negative, deleterious effects seen within reps treated with 1% PGS.
In previous, unpublished work, it was determined that PGS contains a high level of nitrogen (N), at 4.14% overall content. While N fertilizer is beneficial to plants, an excess in N is phytotoxic. All-purpose, synthetic store-bought NPK fertilizer commonly contains N at 24% of its overall content, and manufacturers recommend a total application of approximately 15g per 9.3 m2. Of this total application, 3.6 g total is N applied over the 9.3 m2 area (Miracle-Gro, The Scotts Company LLC., Marysville, OH). At a 1% PGS amendment rate, 25 g PGS is applied to an approximately 0.01 m2 area pot, with a total of 1.035 g N applied to a considerably smaller area of soil. Based on this information, it is likely that the N content present in 1% PGS compared to 0.5% PGS amendment is too high and having negative effects on overall plant health.
C. Fusarium root colonization
No significance between treatments was observed from data obtained, likely due to overall low infection rates occurring. The mean number of root pieces demonstrating Fusarium growth on selective media was equal to or less than 0.5 for all treatment groups (Fig. 33D). Field soil collected and used in this trial was obtained from a commercial lettuce and leafy green farm that reported Fusarium wilt disease issues in previous years, however when discussing the problem with the project team, the grower originally described the disease as being an issue during several cropping cycles, but not all.
Characterization of vapor-phase BITC transmission
In biofumigation using mustard greens, the active biofumigant allyl-isothiocyanate (AITC) is transmitted primarily via the vapor phase. However, the active biofumigant compound in PGS, i.e., BITC, is much less volatile. To characterize to what extents BITC is transmitted via vapor vs dissolved state, we set up a series of tests with either pure BIT or PGS placed in air-tight tubes and the gas phase samples were taken and analyzed with gas chromatography. However, we were unable to detect BITC. We can not rule out that some gaseous BITC was still formed but our sampling and analytical method was not sensitive enough to pick up the signal. That said, this result suggests that the vapor-phase transmission of BIT may be low. We then did a simple experiment in which we placed PGS in a small peri dish which was placed inside of a regular sized petri dish at its center and PDA agar was poured afterwards to surround the PGS in the center. F. oxysporum agar plugs were inoculated onto the agar portion of the plate as in typical agar-plat assays. With this simple design, we were able to create a solid partition between PGS and the surrounding agar, and still allow gaseous BITC to be released from the top surface of PGS. As seen in Figure 34 (note the photos were taken from the back side of the petri dish), PGS without solid partition was capable of inhibiting fungal growth, while a much lower level of inhibition was observed with the solid partition, though still a little better than the control without any PGS. These results strongly suggest that the BITC released from PGS after water activation is transmitted mainly in its dissolved state, instead of its gaseous state. A similar observation has been reported in the literature comparing AITC and BITC as potential antimicrobial agents (Hareyama et al. 2022).
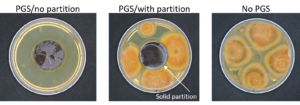
Probing PGS interaction with soil-borne fungi using a novel transparent soil system
In the aforementioned soil-based assay systems, PGS and fungal mycelia were mixed into the soil and incubated for a few days before subsequent analysis based on plating on a selective medium. Therefore, it is not possible to examine what exactly happened during the soil incubation. In recent years, emergence of the transparent soil technology has offered a novel approach for live imaging and analysis of soil microcosms. Here we adopted a transparent soil system that consists of cryolite particles immersed in a Ludox TMA (colloidal silica suspension) solution. By matching the refractive index, the cryolite particles become almost transparent in Ludox TMA, whereas in water, the cryolite suspension looked murky (Figure 35). This transparent soil system was mixed with a dilute F. oxysporum mycelial suspension (stained with a fluorescent dye) and loaded into a PDMS microfluidic chip. The Fusarium fungal mycelia at different depths within the transparent soil matrix could be clearly visualized using a confocal laser scanning microscope (Figure 35). In further studies, this chip will be connected to a fluidic system to introduce BITC, PGS extract, and/or nutrients, and be examined in real time using an inverted or confocal microscope system. Such live imaging analysis will provide highly valuable real-time characterization of fungal inactivation in response to PGS or BITC treatment in a simulated soil environment, and enable development of more efficient PGS application methods.
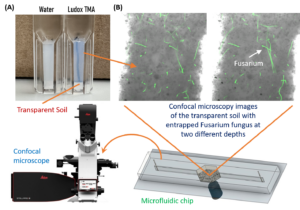
References cited:
Hareyama, Yohei, Mitsunori Tarao, Koki Toyota, Tomohiro Furukawa, Yoshiharu Fujii, and Masayo Kushiro. 2022. "Effects of Four Isothiocyanates in Dissolved and Gaseous States on the Growth and Aflatoxin Production of Aspergillus flavus In Vitro" Toxins 14, no. 11: 756. https://doi.org/10.3390/toxins14110756
***********************
Final reporting period results:
Rhizoctonia solani qPCR
In order to accurately measure levels of R. solani in agricultural fields and soil, and diseased kai choi root tissue suspected to be Rhizoctonia-infested, a real-time quantitative PCR assay was designed for use as a quantitative research tool for greenhouse and field experiments.
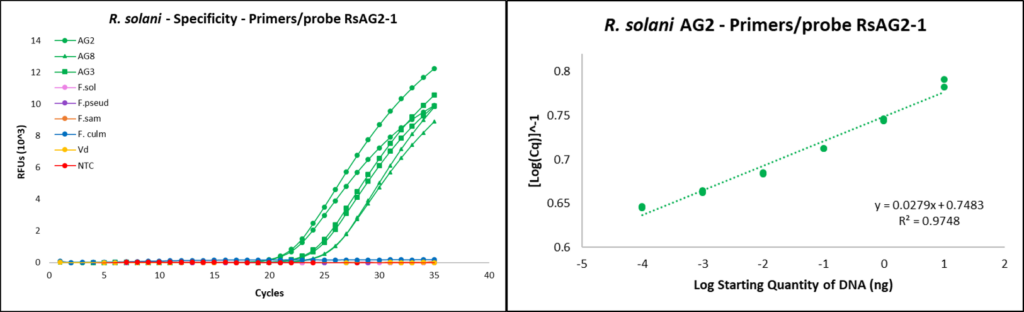
A. R. solani qPCR validation
To validate that the primers/probe used for the detection of R. solani would amplify only the targeted pathogen, R. solani pure culture isolates from anastomosis groups (AGs) 2, 3, and 8 were included as positive controls, and non-target pure culture isolates Fusarium solani, F. pseudograminearum, F. sambucinum, F. culmorum, and Verticillium dahliae were included as negative controls. Nuclease-free water was used as a no template control. To assess the overall sensitivity of the primers/probe, a 10-fold serial dilution of R. solani AG-2 was performed.
It was successfully demonstrated that the assay targeted and amplified various R. solani AGs while avoiding amplification of non-specific targets (Fig. 36, left). Additionally, the assay showed consistent detection sensitivity down to 0.1 pg of DNA (Fig. 36, right). Both assessments show high sensitivity and specificity of the assay, highlighting its potential as an accurate and sensitive detection tool for R. solani in future experiments. However, DNA samples extracted from soil samples suspected to be infested with R. solani were tested, and very low amplification occurred, indicating that R. solani may not be the primary pathogen causing disease in kai choi (not shown).
Fusarium commune qPCR
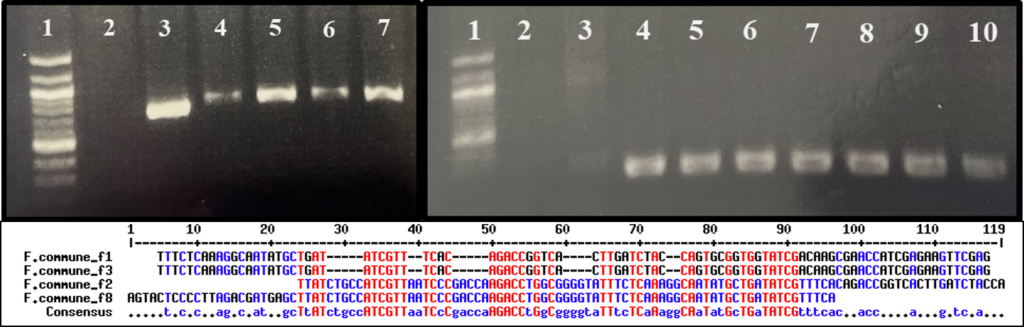
A. Fusarium isolate sequencing
In order to determine the causal pathogen of disease in kai choi, after low levels of R. solani was detected in diseased field soil samples, Fusarium single-spore isolates were obtained from diseased kai choi roots and sequenced. Of the sequenced isolates, after BLAST analysis, four Fusarium isolates returned with a 100% match for Fusarium commune, a known species involved in the Fusarium wilt and root rot disease complex. Based on the obtained sequence data, it was suspected that F. commune was a causal pathogen for disease in the kai choi plants rather than R. solani (Fig. 37).
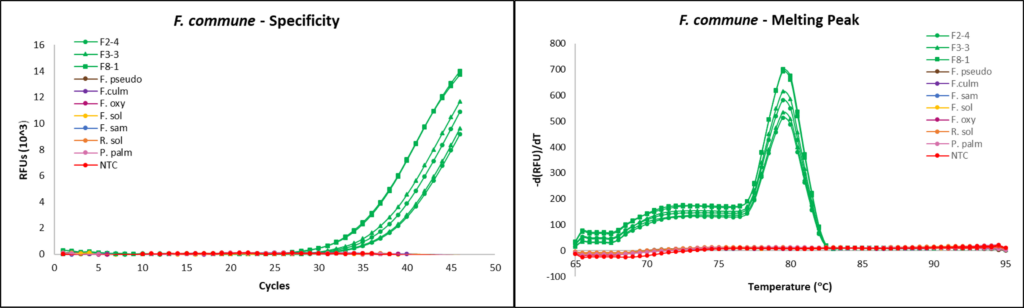
B. F. commune qPCR development
To test for and accurately measure levels of F. commune in agricultural soil and diseased kai choi root tissue alongside R. solani, a preliminary, SYBR green-based RT-qPCR assay was designed for use. To validate that primers were specific to amplifying only F. commune and not other Fusarium species, three sequenced Fusarium isolates with matches for F. commune were included. Non-target, pure culture isolates of Fusarium pseudograminearum, F. culmorum, F. oxysporum f.sp. cubense, F. solani, F. sambucinum, Rhizoctonia solani, and Phytophthora palmivora were included as negative controls, nad nuclease-free water was used as a no template control.
We were able to demonstrate that the designed assay could amplify several F. commune isolates, while also being specific enough to avoid amplifying non-target species, including other pathogenic Fusarium (Fig. 38). Additionally, melting curves for the amplified targets reached their peaks at the same temperature, further confirming all sequenced F. commune isolates used in this assay are of the same species (Fig. 38).
However, initial amplification results showed late amplification of pure F. commune isolates. qPCR assays frequently face low or no amplification signals during reactions due to residual inhibitors present in the DNA template, including phenols, detergents, proteases, or other residuals specific to source material or tissue. Thus, this is one factor that may be contributing to the late amplification of the F. commune template used.
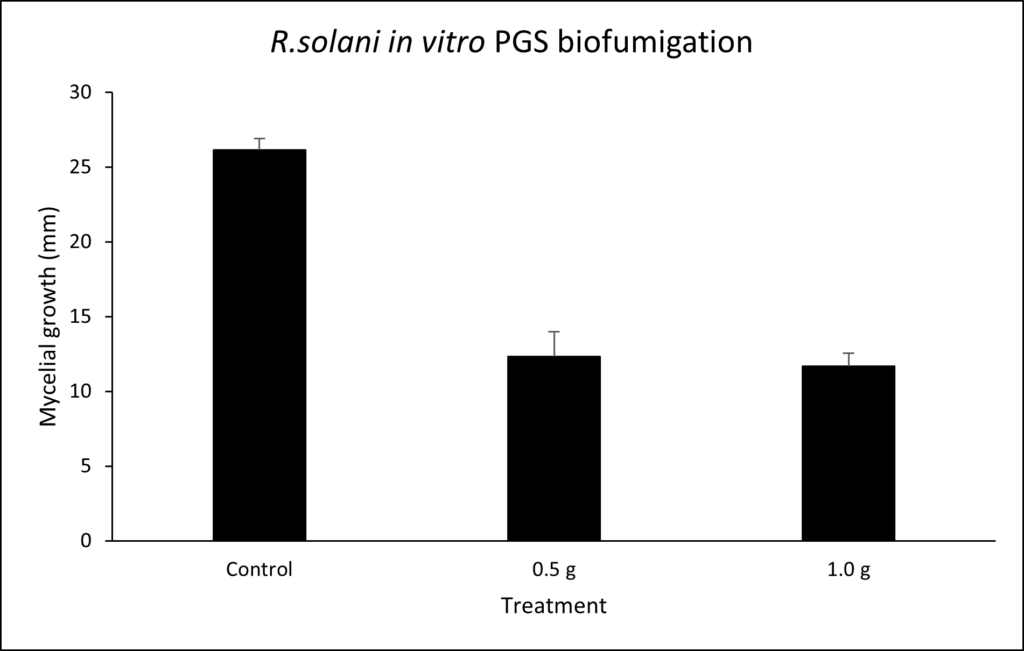
Rhizoctonia solani in vitro PGS biofumigation
To assess the toxicity of PGS biofumigation against pure R. solani, we conducted an additional in vitro PGS assay, performed similarly as the assay on other fungal species in year two of the project. After 48 hours of growth, R. solani mycelial growth was significantly lower in PGS-treated groups versus the untreated, control group. Both 0.5 g PGS and 1 g PGS performed comparably, indicating that lower concentrations of PGS are sufficient enough to slow R. solani growth. However, neither PGS concentration tested was able to completely stop growth (Fig. 39).
Kai choi greenhouse biofumigation (+/- leachate) trial II
Overall results obtained from this trial displayed no significant interaction between pots that were treated with kai choi root leachate, versus those that were not. All kai choi reps for each treatment group were therefore pooled (n=8) regardless of leachate treatment or not.
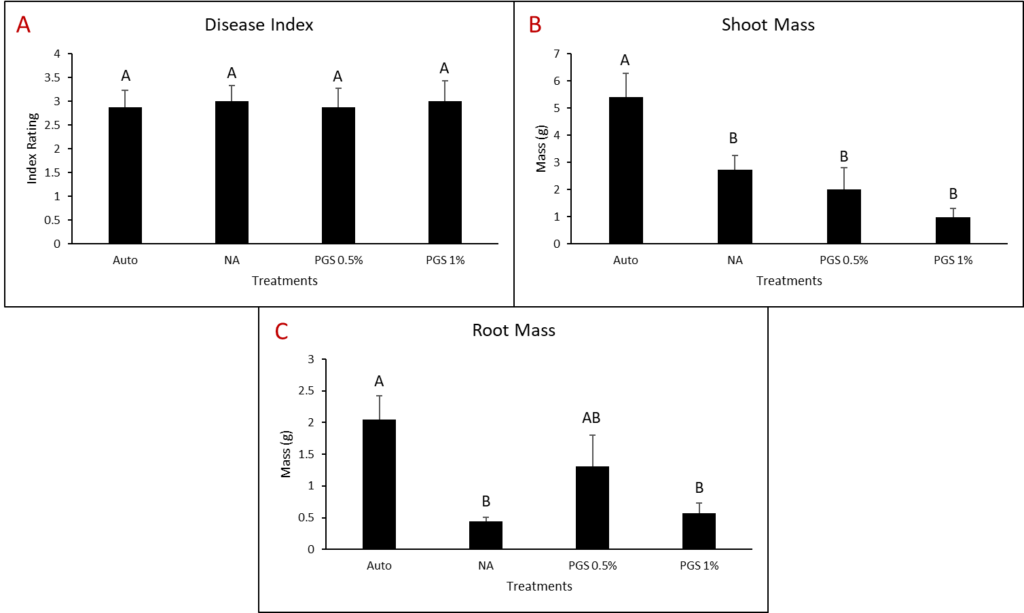
A. Disease Index
Disease index was measured based on a pre-determined kai choi disease scale of 1 to 4. Based on this scale, there were no differences observed between the treatment groups, including autoclaved soil pots (Fig. 40A). However, due to high heat conditions present in the greenhouse environment at the time of growing, there were complications with preventing water-related wilting in the plants. This is likely the reason that autoclaved treated plants had a visual disease rating that was comparable to the non-amended treatment group, despite being expected to have minimal disease symptoms.
B. Shoot & Root Mass
The autoclave group had the greatest shoot mass despite showing wilting symptoms. All other treatments had significantly less shoot mass, with a decreasing numerical trend shown with increased PGS concentration (Fig. 40B). As we saw this occur with an increase in the amount of PGS used, which subsequently increased the level of BITC produced, it is suspected that phytotoxicity is occurring as it did in early greenhouse trials, due to transplanting kai choi seedlings 48 hours after PGS amendment in this trial rather than 7 days after in previous trials.
However, contrary to the data observed in the shoot mass, the root mass of pots treated with 0.5% PGS were comparable to the autoclaved treatment. The non-amended treatment had the lowest root mass, with 1% PGS having a numerically higher root mass (Fig. 40C). These observed results may indicate that despite initial phytotoxicity, roots in the PGS-treated pots were recovering.
Cowpea greenhouse biofumigation trial II
A. Disease Index
Disease index was measured based on a pre-determined cowpea disease scale of 1 to 4 (Fig. 41). Based on this scale, disease observed was comparable in all treatments, with the non-amended treatment being the numerical lowest (Fig. 42, left). Due to the autoclaved treatment group showing some signs of disease, it is suspected that some abiotic disease symptoms were present.
B. Nematodes per gram of root
After acid fuchsin staining roots, the number of vermiform and female reniform nematodes (RN) and egg masses were counted under a dissecting microscope. There was a comparable number of vermiform nematodes and egg masses observed in the PGS-treated versus non-amended pots. However, PGS-treated pots had a lower number of female RN present in roots (Fig. 42, right). This suggests that while there is a larger number of vermiform nematodes present, the BITC produced by PGS appears to disrupt the RN lifecycle and suppress reproduction. Additionally, there was an overall numerical decrease in the number of vermiform nematodes observed in the 1% PGS treated pots compared to the non-amended pots, indicating that the 1% PGS treatment has some suppressive effect on the RN overall (Fig. 42, right).
Kai choi greenhouse biofumigation (+/- surfactant) trial I
In order to assess the effectiveness of using a detergent/surfactant to improve BITC molecule movement through the soil, a kai choi PGS biofumigation trial was performed, in which pots were either treated with 80 mL of a 0.1% Tween80 solution during amendment or 80 mL of water.
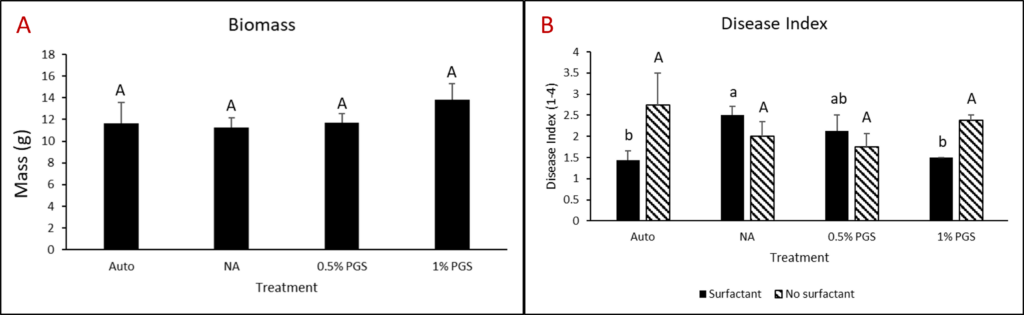
A. Biomass
No significant differences were observed between pots treated or not treated with the 0.1% Tween80 solution. All kai choi reps for each treatment group, when assessing biomass, were therefore pooled (n=8) regardless of surfactant treated or not. Overall, biomass observed was not significantly different, however the 1% PGS treatment group was observed to have numerically higher biomass than all other treatments, suggesting the beginnings of a beneficial trend (Fig. 43A).
B. Disease Index
There were some effects of applying versus not applying surfactant in observed disease index ratings. In the 1% PGS treated group, disease index was significantly reduced compared to the non-amended group, and comparable to the autoclaved treatment group (Fig. 43B). Additionally, there was a slight numerical decrease in observed disease index in the 0.5% PGS group, suggesting that the use of surfactant in the watering during amendment may aid in the dispersal of BITC through the soil, especially when using larger concentrations of PGS (Fig. 43B).
Kai choi sorghum and PGS+CE biofumigation field trial
Due to poor results in the field trial performed during year two of this project, and in vitro and greenhouse results that showed the potential for a surfactant to aid in dispersing BITC through the soil, a kai choi field trial was conducted to examine the efficacy of a PGS crude extract (PGS+CE), containing the Tween80 surfactant, on suppressing Fusarium wilt disease in a commercial kai choi field.
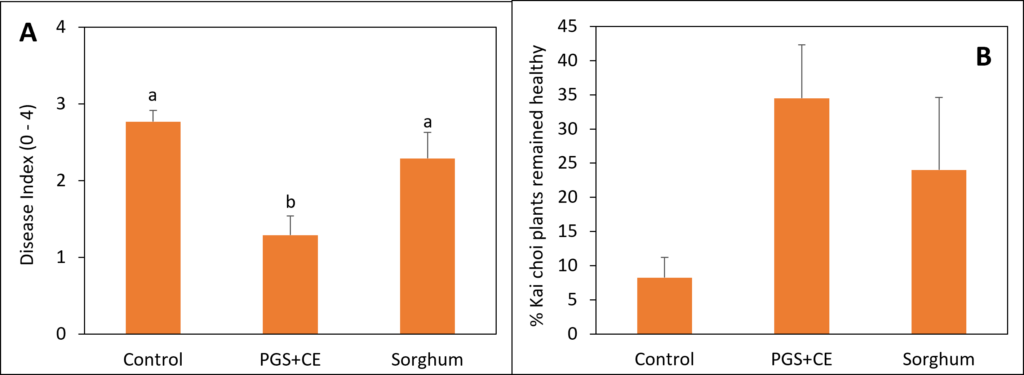
A. Disease Index
PGS+CE treated plots showed significantly reduced disease compared to both the untreated, control plots and plots amended with sorghum (Fig. 44A). These results demonstrated that PGS+CE was effective in suppressing disease compared to sorghum amendment and plots that went untreated.
B. Percent healthy plants
As expected, untreated plots had the lowest percentage of healthy plants remaining, where the PGS+CE treated plots showed a greatly increased percentage of healthy kai choi plants, having the highest number of healthy plants compared to both the untreated and sorghum amended groups (Fig. 44B). Additionally, data obtained from the year two kai choi field trial for disease incidence, in which PGS alone was applied, showed the PGS treatment groups as having the highest levels of disease compared to sorghum amended and untreated plots (Fig.12). This further supports the use of PGS+CE as a more effective application method over the use of PGS alone.
Further characterization of fungal growth upon PGS treatments in a soil-based assay
We improved the preparation of the initial mycelia suspension for inoculation to enable quantification of fungal growth upon PGS treatments in the in vitro soil-based assay. We conducted the assay to examine F. solani, in addition to F. oxysporum. The initial mycelia inoculum size in soil was set at about 5-7 x 105 cfu per gram of soil, which is high compared to typical pathogen levels in infested field soil. Even under such a condition, PGS amendment rate at 0.5% was shown to completely suppress the growth of the two Fusarium sp. (Figure 45) This PGS threshold is lower than what was reported in our report last year, and it is mainly because the inoculum size used in last year’s experiment was about an order higher. For F. solani and F. oxysporum, PGS in soil is highly inhibitory.
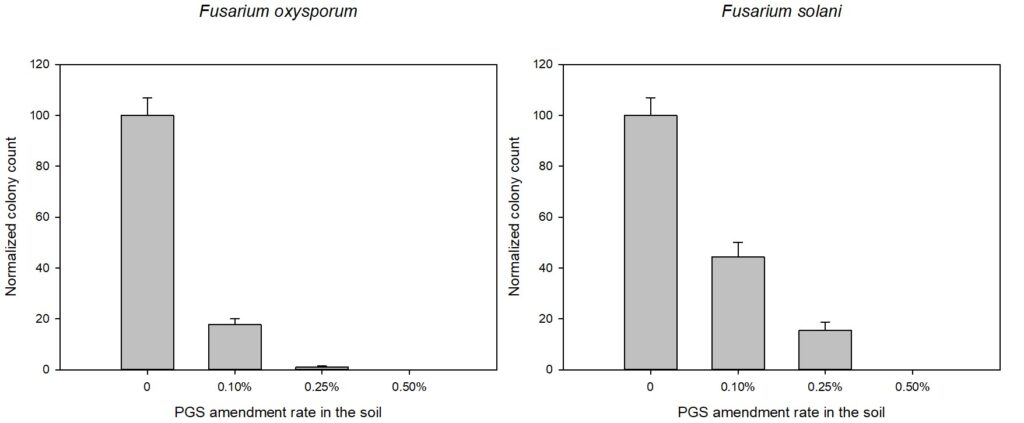
Direct imaging of Fusarium oxysporum growth inhibition in response to PGS amendment in a transparent soil system
In our last report, we described a transparent soil system that consists of cryolite particles immersed in a Ludox TMA (colloidal silica suspension) solution. By matching the refractive index, the cryolite particles become almost transparent in Ludox TMA, at modest cryolite particle concentrations. Unfortunately, under higher concentrations that mimic natural soil, the system becomes less transparent. Therefore, we evaluated an alternative transparent soil model made of alginate hydrogel particles in water (Ma et al. 2019). This system is simple to set up and remains transparent at high particle packing densities. By loading the transparent soil inoculated with F. oxysporum into a PDMS microfluidic device which allows the fungus to grow in a soil-like environment, we characterized the effect of 1% PGS amendment by direct imaging of the fungal growth in the microchip using confocal microscopy. The results in Figure 46 provided direct proof that PGS in the soil-like environment can indeed inhibit growth of F. oxysporum. The microfluidic chip coupled with hydrogel transparent soil and confocal microscopy offers a unique system for live cell imaging in a soil-like environment, and it can be used to examine additional aspects of PGS biofumigation, such as effect of PGS extract drenching and diffusion effect of BITC in the soil on fungal growth inhibition.
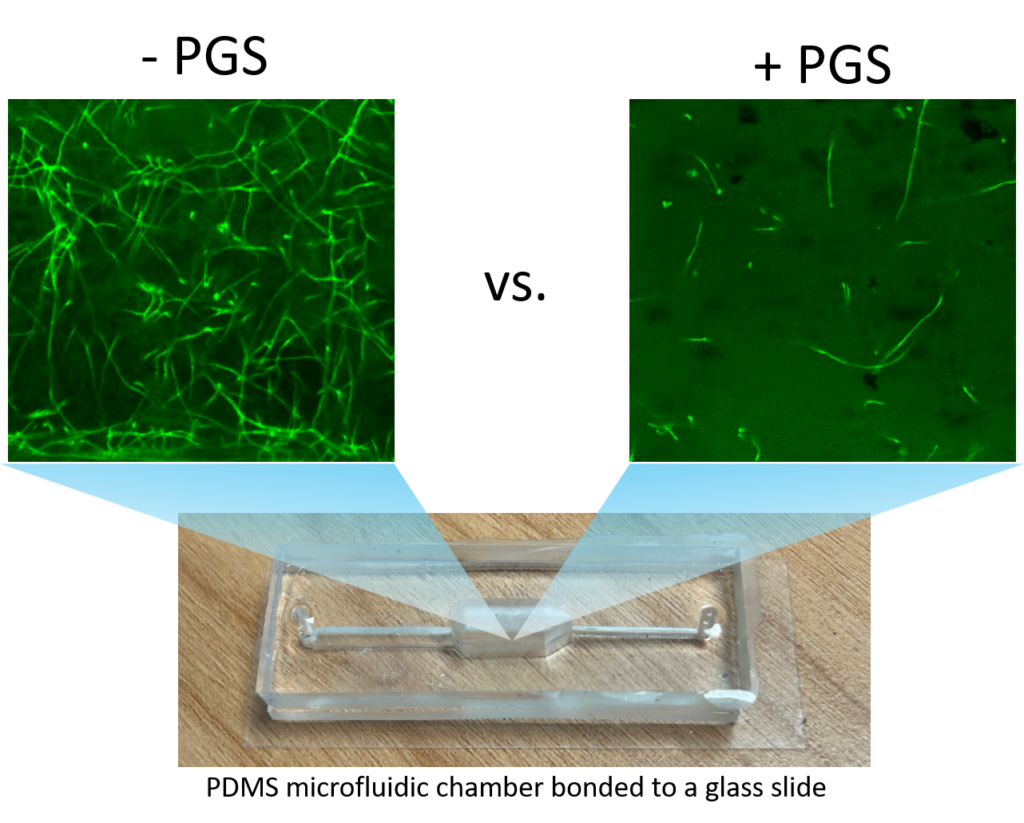
Research conclusions:
*************
Year-3 concluding remarks:
in vitro studies
Based on fungal growth on ½ Potato Dextrose Agar supplemented with 0.1 or 0.5 mM pure BITC, we were able to demonstrate that pure BITC was able to suppress various fungal pathogens, including F. oxysporum (the causal pathogen of Fusarium wilt) and R. solani (the causal pathogen of Rhizoctonia rot), especially at the higher concentration of 0.5 mM (74.6 mg/l). Further tests with F. oxysporum using PGS in soil-based in vitro tests clearly demonstrated inhibitory effects on mycelial growth at PGS amendment rates above 1%, and showing strong inhibition at or above 3%. Moreover, dissolved state rather than gaseous state BITC released from PGS is most responsible for the anti-fungal activity in soil. These findings are significant, because they provide direct evidence that BITC can be released from PGS and transmitted efficiently through soil (and not just in agar media) to reach the phytopathogen for inactivation. Because dissolved state BITC is likely most responsible for PGS’s anti-fungal activity, soil moisture content plays a role in PGS’s efficacy as a soil biofumigant. It is also better to disperse PGS in the soil rather than applying it as an agglomerate. Similar soil-based tests will be conducted to examine PGS effect on additional soil phytopathogens such as F. solani and R. solani. These simple in-vitro assays can inform approximate PGS dosage for field applications.
Kai choi greenhouse biofumigation trial
It was speculated PGS biofumigation was less effective in the field due to the presence of fungal survival structures (sclerotia of R. solani, chlamydospores of Fusarium) which are less prone to allelopathy of BITC. The hypothesis was that root exudates could signal fungal pathogens to exit the survival state, making the PGS more effective in suppressing the vulnerable stage of the fungi. Initial results showed no noteworthy improvement of PGS by root leachate against F. oxysporum but did show total suppression of R. solani colonization on kai choi roots when soil was amended at 1% PGS after receiving root leachate 2 days prior to soil amendment. The experiment needs to be repeated to verify this finding. On the other hand, nitrogen content in PGS contributed to some green manure effects on kai choi growth.
Lettuce greenhouse biofumigation trial
Overall Fusarium infection during this trial was low, rendering the test of PGS on lettuce inconclusive. However, shoot and root biomass were different among treatments despite the lack of disease symptoms and infection rate among treatments. Therefore, it is believed that outside of disease suppression, PGS can provide some green manure benefits at an optimal concentration but can also prove to be phytotoxic and stunt growth if the concentration is too high. This is suspected to be related to nitrogen toxicity from the high nitrogen content of PGS, but further experimentation is necessary to confirm this.
Additional trials are needed to obtain data from lettuce using soil with higher population densities of F. oxysporum, and to verify the green manure benefits vs phytotoxicity of PGS to lettuce.
Cowpea greenhouse biofumigation trial
Results from this trial was inconclusive due to premature death of plants unrelated to reniform nematodes. In order to elucidate the effectiveness of PGS amendment against reniform nematodes, additional cowpea greenhouse trials are needed. To assess the effectiveness of BITC and PGS biofumigation against nematodes in a controlled lab experiment, simple in vitro assays like those performed for F. oxysporum and R. solani can also be completed.
*********
Final report concluding remarks:
R. solani qPCR
The project team successfully developed and validated a real-time qPCR assay for the detection of R. solani in clay-like soils in Hawaii, but due to overall low detection levels of R. solani from soil samples collected from an infested field with high disease pressure, it was concluded that a different pathogen was the primary cause of infection and disease in the affected kai choi.
F. commune qPCR
Fusarium isolates obtained from diseased kai choi roots were successfully sequenced and identified as Fusarium commune. Thus, we suspected F. commune as the pathogen causing primary disease symptoms in kai choi, and designed a preliminary qPCR assay for the detection of F. commune.
However, in initial qPCR reaction runs for F. commune, amplification curves appeared late, which can be an issue for detection of lower pathogen concentrations in root or soil samples. Prior to using the designed qPCR assay as a quantification tool in other experiments, further optimization and validation of the thermal protocol, the reaction buffer concentrations, and the DNA extraction method is required.
R. solani in vitro assay
Based on our results, PGS biofumigation in an in vitro setting demonstrated effective suppression of R. solani growth, but did not completely stop mycelial growth. Therefore, while PGS has the potential as an alternative treatment against R. solani, more in vitro tests are needed to elucidate the concentrations of BITC, and subsequently PGS, required to completely halt R. solani growth.
Kai choi greenhouse biofumigation +/- leachate – Trial II
During this trial, the time between PGS amendment and seedling transplant was reduced from 7 days to 48 hours. However, due to a general downward trend observed in shoot mass, with both concentrations of PGS used having decreased mass compared to the untreated reps, it was determined that mild phytotoxicity was occurring in the plants. Based on this, we concluded that 48 hours post-amendment was still too short.
However, with an increase in root mass observed in PGS treated reps (especially with 0.5% PGS) compared to untreated reps, it was found that despite initial phytotoxicity, roots in the PGS treated reps were recovering, whereas the untreated reps continued to show low root mass due to disease.
Cowpea greenhouse biofumigation – trial II
Observed aboveground disease symptoms in this trial were not consistent with data collected on nematode counts in the roots, likely due to abiotic stress factors. When analyzing results obtained from nematode count data, 1% PGS treated reps showed low level suppression of vermiform nematode infection as well as a greater suppression of female RN infection on cowpea roots. Due to an overall low level of suppression, it is suspected that a higher concentration of PGS has potential for greater effect in suppressing RN. As the most significant decrease observed in nematodes infesting plant roots was in female RN counts, it is also believed that BITC was disrupting the RN lifecycle in some way, preventing vermiform RN from reaching female reproductive maturity. Therefore, PGS biofumigation has potential as an alternative control method against RN, but more understanding is needed on the mechanism of BITC suppression on RN in order to better design future greenhouse and field experiments for its application and use.
Kai choi greenhouse biofumigation +/- surfactant – Trial I
When assessing overall disease symptoms observed in kai choi plants, the use of a 0.1% Tween80 surfactant solution during PGS amendment showed to be effective in decreasing disease symptoms in reps using 1% PGS.
Due to BITC being a large, hydrophobic molecule, it was initially hypothesized that the use of a detergent or surfactant would aid in moving BITC through the soil. Based on preliminary data collected from this trial, it was determined that the combined use of the surfactant with a 1% PGS amendment has potential as an optimal method of PGS application, however additional trials demonstrating similar results need to be completed to further validate this assumption.
Sorghum & PGS+CE field trial
In this field trial, it was demonstrated that using PGS in a crude extract form increased disease suppression, subsequently increasing overall healthy, marketable kai choi yield. When compared to the previous kai choi field trial in year two of this project, where PGS only-treated plots had more incidence of disease than sorghum-treated plots, and were comparable to the untreated plots. There was significant improvement in kai choi health when using the PGS+CE application method.
It is believed that the use of 0.1% Tween80 as a surfactant in the CE preparation process, combined with the drenching method used when applying the extract, assists in uniform movement of the BITC molecules through field soil, as similarly concluded from the kai choi greenhouse trial conducted using 0.1% Tween80 when amending using dry PGS. We conclude that PGS performs better as a treatment against disease caused by Fusarium when used with a surfactant, especially in the production of crude extract.
The PGS biofumigant can be produced easily by simply drying the papaya seeds at 50°C for 2 days, followed by milling into fine powders. Dry seeds stored for over a month under room temperature can produce similar amounts of BITC upon milling into PGS, as those from fresh seeds. Seeds from Sunrise and Rainbow papaya generate similar amounts of BITC. The greenhouse pot experiments revealed that PGS at 0.5 or 1% amendment rate level could pose a phytotoxicity effect on young lettuce seedlings if transplanted immediately after soil amendment, but planting at 1 week after amendment avoided this problem. PGS at 1% rate was more consistent in suppressing F. oxysporum than PGS at 0.5%. Adding papaya seed crude extract (PGS+CE) did not improve either F. oxysporum or root-knot nematode suppression than using PGS alone. Further optimization of crude extract preparation is necessary. Overall, the results indicate that PGS would be safer to use as post-plant treatment or at least 1 week before seedling transplanting. Besides effects on the crop and the target pathogens, it is useful to probe the environmental impacts of PGS by examining its effect on soil microbial diversity. To this end, the 16S rRNA gene amplicon sequencing is shown to be a useful approach. Different PGS treatments (PGS+CE, PGS 0.5%, PGS 1%, and NA) resulted in significantly different microbial composition in soil, as indicated by the statistically different Beta diversity indices, but did not significantly affect the microbial richness, as indicated by the non-statistically different Alpha diversity indices. Eight plant growth promoting bacteria were identified in the soil samples. Different treatments resulted in significant difference in the abundances of these plant growth promoting bacteria. The abundance of plant growth promoting bacteria under the PGS+CE treatment was significantly lower than under other treatments. Interestingly, treatment with PGS at 1% amendment rate showed the highest abundance of plant growth promoting bacteria.
**********
Year 2 concluding remarks:
BITC emission from PGS
Laboratory tests in 50 mL sealed centrifuge tubes using a mineral oil layer to trap PGS-generated BITC allowed rapid comparison of a variety of factors for their effects on BITC release from PGS. Among the factors investigated, PGS particle size, water content, PGS distribution in soil, freezing of papaya seeds prior to drying/milling, and inclusion of vegetable oil in PGS amendment, all showed some degrees of influence on BITC release from PGS. We also showed that BITC release from PGS could last for several days. In year 3, these measurements will be further refined, including comparison of results with direct gas-phase BITC measurements using gas chromatography available in a new collaborator’s lab. Findings from these tests provide insights into developing better practices for more efficient use of PGS in the field implementation of PGS-based biofumigation.
PGS Biofumigation plate assays:
Based on results obtained, PGS biofumigation appears to work well against F. oxysporum in vitro, which is vital as it is the causal agent of Fusarium wilt in various plants and the primary concern for the kai choi fields of participating growers. With further optimization, in the greenhouse and in field trials, there is promise for PGS biofumigation to be recommended as a potential control method of Fusarium wilt. However, right now it’s still unclear whether or not this biofumigation method is effective against other fungal plant pathogens (as seen with F. solani and Setophoma sp.) so to test whether it is the PGS application method that is ineffective to other fungal species or the BITC compound released that is ineffective, another plate fumigation assay will be performed using pure BITC in place of PGS. Pure MITC, the active ingredient in Vapam, will also be tested in vitro to compare its strength in suppressing fungal pathogens to the strength of BITC.
Field biofumigation:
To troubleshoot the PGS amendment and optimize it for future field trials, there are various, small greenhouse experiments planned to determine how best to apply the PGS amendment in the field to get the best results. Most important limiting factor is insufficient amount of PGS can be prepared for entire bed preparation and resulted in uneven distribution of PGS beyond the root zone of kai choi. Other factors that could help to resolve this issue include how deep the PGS needs to be in the soil or how wet the soil needs to be in order to release and dissipate BITC into the surrounding area. Planned experiments to answer this question include basic pot experiments that will measure the amount of BITC captured when PGS is buried at various depths in potting conditions as well as an experiment that will measure volumetric soil moisture and the relative amount of BITC that is released linearly. It is also supposed that not enough PGS was used in the treated plots as well, as the field set up included digging trenches in the plots and only amending those locations. Since the sorghum and Vapam plots were prepared by the grower and employees involved, and the kai choi seedlings were also transplanted by farm employees, the research group was unable to oversee every part of the experimental plot preparation. The trenches were not clearly marked, so it is likely kai choi was not planted directly on PGS and it’s unclear the area that BITC can penetrate. Based on this, it may be beneficial to cover entire plots with the PGS amendment moving forward.
Research Outcomes
We completed a field trial in year 2. During the year-2 field trial, we observed early protection of kai choi seedlings from the fungal disease complex for at least 3 weeks after transplanting. Although the trench application of PGS used in our field trial could not effectively protect kai choi from fungal damages, our laboratory characterization of BITC emission from PGS revealed alternative application schemes of PGS that may improve the PGS efficacy, which will be evaluated further during year 3 in additional greenhouse and field trials. Some of the modifications will include reduction of PGS particle sizes and optimizing the duration of PGS incubation in soil prior to transplanting to maximize soil fumigation while minimizing toxicity to the crop plants. Additional improvements may come from optimizing the way PGS is dispersed in the soil, maintaining soil moisture level during biofumigation, and post plant drenching with refined aqueous PGS extract (to provide additional BG as myrosinase substrate). As we learned more about the characteristics and best application practices of papaya ground seeds as a natural soil fumigant, we will continue to work on outreach to disseminate our findings to the public and to promote adoption of this sustainable plant disease management practice.
During year 3 we completed one greenhouse trial each for kai choi, lettuce, and cowpea and in vitro studies testing pure BITC, PGS, and PGS-extracts against various soil-borne fungal plant pathogens in agar- and soil-based assays. The kai choi and lettuce trials showed positive results when using 0.5% PGS, but our in vitro soil assays revealed that between 1-2% PGS amendment was necessary to inhibit F. oxysporum. Additional soil-based tests will be conducted to examine PGS effect on F. solani and R. solani. These simple in-vitro assays can be used to set approximate PGS dosages for field applications to combat common soil-borne fungal pathogens. To determine the specific amendment rate to be carried over to our next field trial, we will perform one additional kai choi greenhouse trial and two additional lettuce greenhouse trials to test PGS amendment rates derived from in vitro soil tests (1-2%). To confirm that the collected field soil can cause disease in lettuce seedlings, we will also perform a simple disease assay with non-amended soil and autoclaved soil pots. Additional cowpea trials will be conducted to gather data on the effectiveness of PGS suppression against reniform nematodes, with changes made to lower watering rates compared to kai choi and lettuce in order to avoid root rot issues that were faced in the year 3 trial. In the meantime, Pluronic F-127 gel-based in vitro assays with pure BITC and PGS biofumigation will be performed using R.reniformis vermiform nematodes to determine the effectiveness of BITC and PGS in suppressing this nematode. As these trials are conducted, the PGS concentration that produces the best greenhouse results will be carried to be used in a final field experiment. As final results for this project are obtained, we will work to disseminate new findings in publications, as well as through other outreach avenues.
************
During our final reporting period, we designed and validated a qPCR assay for the detection of R. solani in Hawaii agricultural soil, and designed and tested a preliminary qPCR assay for the detection of F. commune. However, in order to confirm F. commune as the causal pathogen of disease symptoms in commercial kai choi fields, the sequenced F. commune isolates need to be demonstrated in the greenhouse to re-infect healthy kai choi through Koch’s postulates. If confirmed to be the disease-causing pathogen, this would be a first report of F. commune causing disease in plants in Hawaii. Additionally, once optimization of the F. commune qPCR assay is complete, a sensitivity assay needs to be conducted to determine detection power, followed by an assay to detect F. commune in DNA samples extracted from soil and root tissue.
The R. solani in vitro assay demonstrated that PGS can suppress R. solani mycelial growth to an extent, but at concentrations of 0.5g and 1g PGS, it does not completely kill the mycelium and stop its growth. To determine the optimal PGS concentration necessary to completely stop the growth of R. solani mycelium, future in vitro experiments assessing longer growth periods and increments of higher PGS amounts need to be explored. Our kai choi greenhouse trials demonstrated that 1) PGS can still have mild phytotoxic effects at 48 hours post-amendment and 2) 1% PGS concentration is the most effective in suppressing disease in kai choi when a surfactant (Tween80) is mixed with water during amendment. To avoid phytotoxicity caused by BITC released from PGS, it’s recommended that seedling transplant remain at 7 days post-amendment. Otherwise, a greenhouse growth assay could be conducted to elucidate the shortest period between amendment and transplanting that can be used without causing phytotoxicity in kai choi seedlings. The subsequent kai choi field trial demonstrated that drenching field soil with a PGS crude extract was significantly more effective at suppressing disease caused by Fusarium than the previous method applied to field soil: dry PGS drenched with water only. Finally, our cowpea greenhouse trial showed that PGS biofumigation may be disrupting the reproductive lifecycle of reniform nematodes, however the mechanism behind this is not understood, so further studies are needed to make additional conclusions. In order to increase levels of RN suppression in cowpea, higher PGS concentrations need to be assessed. Additionally, future studies designing experiments to understand the mechanism behind BITC suppression on RN and how it disrupts RN reproduction and lifecycles may also provide insight when designing future PGS greenhouse and field experiments.
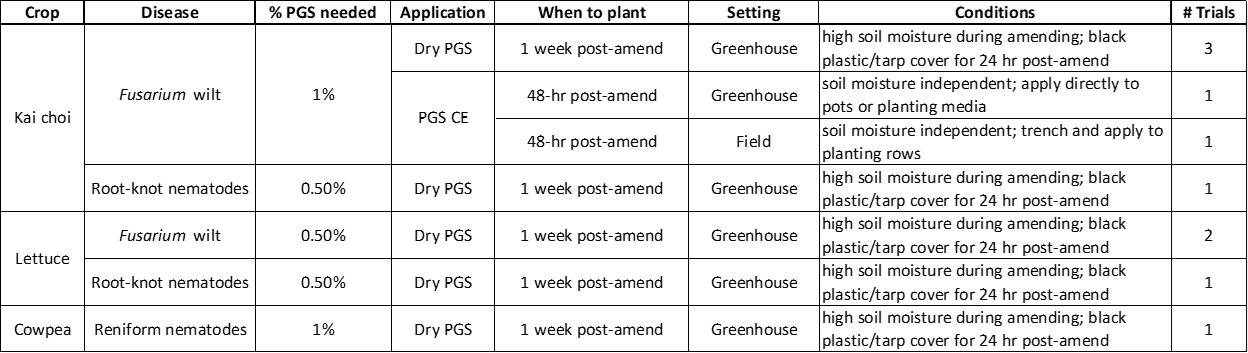
The overall research outcomes of this project…
As summarized in Table 3, one kai choi greenhouse trial and one lettuce greenhouse trial demonstrated PGS biofumigation as highly effective against root knot nematodes (RKN; Meloidogyne incognita), requiring a 0.5% PGS amendment rate to significantly reduce RKN infection. In two lettuce greenhouse trials, dry PGS amendment was also shown to be effective in suppressing Fusarium wilt disease symptoms when using a 0.5% PGS amendment rate. In one cowpea greenhouse trial, there was some effect seen in suppressing reniform nematodes (RN; Rotylenchulus reniformis). This required a rate of 1% PGS, but a higher amendment rate may be needed to see greater RN suppression. To successfully suppress Fusarium in kai choi, it was demonstrated in four greenhouse trials and two field trials that a 1% PGS amendment rate was necessary. The addition of using a surfactant solution when activating dry PGS or when preparing PGS crude extract for drenching showed the best results in suppressing disease.
Education and Outreach
Participation Summary:
During the first-year reporting period, the project is still in the research-data collection stage, so there is no outreach to report yet. However, as we learned more about the characteristics and efficacies of papaya ground seeds as a natural soil fumigant, we will begin to develop outreach activities in year 2 of the project to effectively disseminate the information and knowhow of the biofumigant technology to farmers, producers, and other agricultural professionals, and to solicit their feedback and suggestions. Given the challenges brought by the ongoing COVID pandemic, we needed to modify our original outreach plan that emphasizes on in-person educational workshops and field-day events, to incorporate a “hybrid” approach that relies also on webinars, online trainings, social media, web-conferencing, and other digital media. We plan to host virtual field day events and workshops which feature asynchronous (pre- recorded) and synchronous (live) presentations, interviews, and demonstrations on topics related to this project. For instance, live demonstrations of PGS biofumigant application will be conducted via Zoom or another virtual platform, and recorded, and technical presentations will be given to educate the public about science/technology, economics, as well as the sustainability aspects of the PGS biofumigant product.
Recording of these events will be disseminated via the biofumigation website, social media, and YouTube. Any face-to-face activities to be included in these outreach events will be planed carefully by following Federal, State, County, and University COVID guidelines. The field-day events and educational workshops will target farmers/producers, Cooperative Extension, USDA and state department of agriculture officials, and other agricultural professionals. We will partner with the Western SARE PDP program in Hawaii to recruit field-day participants, and extend technical information transfer to statewide producers, through its multi-agency network. This network brings together extension agents/specialists who work closely with agricultural producers, and other agricultural professionals in agencies such as USDA, Hawaii Farm Bureau Federation, Hawaii Organic Farmers Association, and University of Hawaii at Hilo, Hawaii State Department of Agriculture / Health, county agencies, industry collaborators, Hawaii State Department of Education K-12 programs and other essential public and private organizations. We will make a special effort to recruit and gain the support of new and existing farmers who suffer from production losses linked to plant-parasitic nematodes and other soil borne diseases.
Information and technology dissemination will also be achieved via conventional electronic and paper publications. Research findings will be published in peer-reviewed journals. Technical factsheets and tutorials, as well as other informational handouts and educational videos will be posted online via the internet newsletter of the Sustainable and Organic Agriculture Program (SOAP), Hānai’Ai, which has approximately 1,800 readers. Hard copies of the Hānai’Ai newsletters will be mailed to selected farmers who do not have regular access to internet. We will also partner with organizations which have existing relationships with culturally diverse communities to extend the new technology into the socially disadvantaged communities.
During our second year, the project team had given four guest lectures, one virtual IPM conference, one in-person field day, as described in the Educational & Outreach Activities section below.
One extension publication entitled “Repurposing Papaya: Examining the Potential of Instant Biofumigation using Papaya Seed Waste for Soil-borne Disease Management on Leafy Greens”, coauthored by Lauren Braley, Koon-Hui Wang, Joshua Silva, Tao Yan, Stuart Nakamoto, and Wei Wen Su, was submitted to the CTAHR Extension Publication Office, and it is currently in press. The publication number is PD-123.
Team meetings were held to develop an educational/outreach website on biofumigation, with emphasis on the sustainable PGS biofumigation technology that is being developed in this SARE project. A novel “citizen science” idea was proposed to allow the public to participate in the testing and development of the PGS technology. PGS can be made easily using household coffee grinders and papaya seeds are readily available. Moreover, PGS can be tested in participant’s backyards. The project team will develop simple PGS protocols and questions for distribution via website download, while citizen scientists can share their observations and results with the project team via the website for further data analysis. The findings will be disseminated also via the website. We believe this approach may encourage greater interests from the public in this sustainable practice in farming and even home gardening. Additional features of the website will include an online calculator to estimate PGS amounts for given soil amendment rates, and anonymous questionnaires and surveys.
During our third year, the project team had given two client presentations and one conference presentation, as described in the Educational & Outreach Activities section below. The extension publication entitled “Repurposing Papaya: Examining the Potential of Instant Biofumigation using Papaya Seed Waste for Soil-borne Disease Management on Leafy Greens”, coauthored by Lauren Braley, Koon-Hui Wang, Joshua Silva, Tao Yan, Stuart Nakamoto, and Wei Wen Su, has been published by the CTAHR Extension Publication Office.
*******
During our final reporting period, the project team gave two conference/symposium presentations and four guest lectures to GoFarm Hawaii’s new farmers. Additionally, one in-person field day was organized. All activities are described in the Educational and Outreach Activities section below. A whitepaper was developed to provide farmers and producers a technical guide for setting up farm-sized PGS production as a sustainable biofumigant.
- Multiple discussions on applying PGS in treating Fusarium wilt with a participating producer, Owen Kaneshiro of Owen Kaneshiro farm.
- Discussions with the county extension service Edible Crops agent Sharon Wages on bringing awareness of the papaya seed biofumigant technology to papaya industry practitioners on the Big Island of Hawaii which is the largest papaya growing area of the state.
- New working collaborations with USDA-ARS (US Pacific Basin Agricultural Research Center, PBARC; contact: Dr. Roxana Myers) on greenhouse/field trials to assess the effectiveness of the PGS biofumigant as a treatment at planting to suppress Coffee Root Knot Nematode, parallel to other treatments being tested.
During our second year, the team presented initial findings from the greenhouse study of instant biofumigation with papaya ground seeds (PGS) at a Virtual Soil Health and Sustainable IPM Mini Conference organized by co-PI Uyeda and Wang on Sep 28, 2021. There were 60 participants attended the conference. During a post conference survey, 7 farmers participated in the survey. 100% of the farmers expressed interest to try out PGS biofumigation to manage plant-parasitic nematode problem, however, only 50% of them expressed interest to try PGS biofumigation against soil-born fungal problem. The remaining 50% are interested in using biofumigation from cover crops such as brown mustard or sorghum or continue to use Vapam as a fumigant. Co-PI Wang presented the concept and initial results on PGS-based biofumigation through guest lectures to three cohorts of GoFarm Hawaii (https://gofarmhawaii.org/) which train beginning framers, in the topical area of “sustainable management of nematode and other pests in agroecosystems through cover cropping or biological derived products”. These guest lectures were delivered on: 1) Kauai- Farm Coach: Eric Hanssen (Dec 9, 2021); 2) Waimanalo - Farm Coach: Rachel Ladrig/Coordinator: Laura Ediger (Nov 10, 2021); and 3) Waialua, Oahu - Farm Coach: Dan Caroll (Dec 29, 2021). A total of 36 beginning farmers and 3 farm coaches were reached. Co-PI Wang was also invited as a guest speaker for the University of Hawaii Tropical Plant Pathology Graduate Student Seminar on February 11, 2022. She shared the findings of PGS instant biofumigation studies with 14 participants. A farmer approached our extension co-PI (Silva) and expressed interest to use PGS instant biofumigation for a new fungal disease on onion caused by Setophoma sp. Though our initial petri dish trial did not show significant biofumigant effects against this fungus, we will follow up with seedling treatment in the next progress period. On March 26 2022, an in-person field day visit was conducted by co-PI Wang and a graduate student with GoFarm Hawaii Waialua Cohort to discuss papaya production. During the discussion, the knowledge on PGS instant biofumigation approach was shared with the participating farmers while demonstrating papaya vegetative propagation to help beginning farmers that are interested to develop their own papaya selection. These farmers were fascinated by making use of papaya seeds to manage diseases on farms.
During the third year of this project, a graduate student under co-PI Wang attended the Society of Nematologists 61st Annual Conference in Anchorage, Alaska to share PGS biofumigation research findings with a broader, international community of researchers during the conference poster session. The project team also produced and disseminated documentation through the University of Hawai’i HānaiʻAi newsletter, which is regularly sent to a total of 2000 subscribers. Additionally, two client presentations were given by co-PI Wang on January 11, 2022 for NRCS employees (organized by Susan Kubo) and October 25, 2022 for farmer training in Kauai (organized by India Clark).
- Braley, L.E. and K.-H. Wang. Papaya ground seed as a biofumigant against soil-borne pathogens in Hawai’i. Society of Nematologists 61th Annual Conference, September 26-29, 2022, Anchorage, Alaska (Journal of Nematology 54: 16).
- Paudel, R., L. Braley, Joshua Silva and K.-H. Wang. 2022. Subbing sunn hemp with sorghum and papaya seeds in Fusarium HānaiʻAi 48: Newsletter December 2022. 8 pp. https://gms.ctahr.hawaii.edu/gs/handler/getmedia.ashx?moid=71763&dt=3&g=12
- Wang, K.-H. 2022. Soil health management approaches to help farmers reduce soil-borne disease problem in agroecosystems. NRCS STAC Partner Sharing Session, PIA. Jan 11, 2022 through Zoom. [Susan Kubo, 27 participants, NRCS employees].
- Wang, K.-H. 2022. Biofumigation Approaches (brassica, papaya seeds or sorghum) to help Farmers Reduce Soil-borne Disease Pathogen Problems in Agroecosystems. Oahu RC&D Farmer Soil Health Training in Kauai. Hui O Makaʻainana O Makana, Haea, Kauai. Oct 25, 2022. https://learn.oahurcd.org/training-sessions/course/soil-health-cohort-session-4-1(Organized by India Clark, 14 participants).
********
During the final reporting period of this project, a graduate student under co-PI Wang attended the Society of Nematologists 62nd Annual Meeting in Columbus, Ohio to share research findings on PGS biofumigation and its effect on plant-parasitic nematodes with a broader, international community of researchers during the conference poster session. Additionally, co-PI Wang’s graduate student also gave one oral presentation on PGS as a biofumigant tool and trigger for induced systemic resistance at the ARCS Foundation Inc., Honolulu chapter, scholar symposium, where fellow scholars from a wide range of disciplines were educated on the concept.
One in-person field day was organized and hosted by co-PI Wang and participating educator Jensen Uyeda at the Poamoho Experiment Station in Waialua, Oahu, highlighting the use of PGS biofumigation as a sustainable approach for plant-parasitic nematode management to 20 participating farmers. Co-PI Wang also presented the concept of and current results for PGS-based biofumigation during four additional GoFarm Hawaii guest lectures, where 76 new farmers were reached. Based on a survey given to GoFarm farmers after the lectures, in which multiple approaches to sustainable soil health management were offered, more than 80% of participants expressed interest in adopting some sort of biofumigation method. Though the use of PGS as a biofumigant was not the top choice for new farmers, quite a bit of awareness was brought to the farmers about PGS biofumigation as an option.
Citations:
Braley, L.B. and Wang, KH. Papaya ground seed as a biofumigant against root-knot and reniform nematodes in Hawai’i. Poster presentation at: Society of Nematologists 62nd Annual Meeting. 2023 July 12: Columbus, OH. (174 participants)
Braley, L.B. Papaya Ground Seed as a Biofumigant and Trigger for Induced Systemic Resistance against Soil-Borne Pathogens in Hawaii. Oral presentation at: ARCS Scholar Award Symposium for the Dr. Jacqueline Maly Award in Tropical Agriculture. 2023 April 15: Honolulu, HI.
Wang, K.-H. Soil health and soil-borne disease management. GoFarm at Waimanalo and Kauai. Farm Coach, Rachel Ladrig and Terry Lau, May 3, 2023 (20 participants).
Wang, K.-H. Soil health and soil-borne disease management. GoFarm at Hilo. Farm Coach, Danny Randerson. May 2, 2023 (12 participants).
Wang, K.-H. Cover crop and Nematode Management. GoFarm at Hilo, Farm Coach, Danny Randerson, Jun 19, 2023 (12 participants).
Wang, K.-H. Cover crop, nematode management and field sanitation. GoFarm at Waialua, Farm Coach, Dan Caroll, December 27, 2023 (12 participants).
Wang, K.-H., J. Uyeda, 2023. Sustainable nematode management. Aug 4, 2023. Poamoho Experiment Station, Waialua, HI (20 participants).
Education and Outreach Outcomes
We plan to hold additional client presentations and workshops to disseminate the technical know-how of PGS biofumigation to farmers and agriculture professionals.
- Development of a new approach to enable effective management of soil-borne pests by recycling/repurposing agricultural wastes
Information Products
- Repurposing Papaya: Examining the Potential of Instant Biofumigation using Papaya Seed Waste for Soil-borne Disease Management on Leafy Greens (Article/Newsletter/Blog)
- Papaya ground seed as a biofumigant against root-knot and reniform nematodes in Hawai’i (Conference/Presentation Material)
- Papaya ground seed as a biofumigant against soil-borne pathogens in Hawai’i (Conference/Presentation Material)
- Subbing Sunn Hemp with Sorghum in Fusarium Soils (Article/Newsletter/Blog)
- Scaling up to farm-sized production of papaya ground seeds (PGS) as a novel biofumigant for sustainable management of soil-borne plant pathogens – a whitepaper (Article/Newsletter/Blog)