Final report for GS15-152
Project Information
Project Abstract
The imminent depletion of the Ogallala Aquifer demands innovative cropping alternatives to prevent dramatic losses of income when water levels are insufficient for irrigated row-crop production in the Southern High Plains. Integrating winter cover crops with summer crops maximizes land productivity and system profitability by improving water infiltration, stabilizing soils, and increasing potential income channels. Even though the benefits of cover crops for nutrient retention and erosion control are well recognized, adoption has been slow because of concerns that cover crops withdraw soil water to the detriment of the summer crop. This small-plot experiment tested the interacting effects of irrigation and tillage management techniques with five cover-crop species on soil water depletion and productivity of the cover and subsequent summer forage crop. The overall aim was to compare the success of residue management schemes in reducing irrigation needs for the water-limited Southern High Plains region. The overall recommendation that culminated from this work is that West Texas producers should plant rye as a cover crop. Dryland rye produces modest yield, even in a dry year, but light irrigation should be used to ensure good forage yield if the winter grazing is desired. The impact of this project is two-fold: strengthen rural communities by ensuring the persistence of profitable agriculture in the region, and stabilizing the soil surface from excessive wind erosion and desiccation.
Statement of Problem, Rationale and Justification
The purpose of this project was to investigate five cover crop species as potential complements to a warm-season beef-stocker grazing system. Historically, producers in the Texas High Plains generate income from beef cattle, cotton, and grain crops. Agriculture in the region relies on supplemental irrigation from the Ogallala Aquifer, an aquifer stretching across eight states and underlying more than 170,000 square miles (HPWD, 2014). Unfortunately, the vitality of the Ogallala is threatened since extraction rates of around 12 inches per year greatly exceed the annual estimated recharge rate of 0.5 inch (HPWD, 2014). With strong gusting winds and limited precipitation (18 inches annually), it is not surprising that many soils are prone to severe erosion, especially during the winter months when most annual croplands are fallow. Integrating winter cover crops will allow producers to take advantage of otherwise fallow land by managing cover crop residue to stabilize and enhance soil resources.
Forage-based livestock systems have proven to be economical and resource-efficient options for the High Plains (Allen et al., 2012). Cover crops are just one possible alternative management strategy to better utilize depleting water supplies from the Ogallala Aquifer and sustain on-farm resources. Allen et al. (2012) reported moderate success in grazing winter cereals as part of an integrated crop-livestock system. Most cereals mature rapidly in the spring, making them difficult to manage as they tie up nutrients and deplete soil water if not properly managed. This trial compared five winter-annual forages harvested under four combinations of irrigation and tillage treatment.
While traditional cattle ranches once dominated Texas agriculture, the industry continually evolves to adapt to new technology and environmental conditions (Kelton, 2007). Fences, wells, and windmills were once considered novel ideas that revolutionized agriculture in the High Plains. Row crop production is now most prevalent, but the imminent disappearance of aquifer resources should encourage producers to seek innovative alternatives to safeguard against dramatic losses of income when pumping capacity is insufficient for irrigated crops. Forage-livestock systems are a viable option for the future of West Texas agriculture, and the inclusion of cover crops managed as surface residue could preserve the economic viability of High Plains agriculture.
The impact of this project is two-fold: strengthen rural communities by ensuring the persistence of agriculture in the region, and stabilizing the soil surface from excessive wind erosion and desiccation. Cattle production is crucial to the region’s agriculture community (Butler and Muir, 2012). This research can help rejuvenate the region’s ranching heritage but do so by promoting forage-based livestock systems that are potentially more productive and profitable than traditional rangelands, and more water-frugal when integrated into a row-crop dominant ecosystem. Texas plays a major role in agriculture, especially in the beef industry since the state had 12.4% of the nation’s cattle in 2014 (NCBA, 2015). Without the adoption of more sustainable, resource-efficient management practices, agriculture cannot persist on the Southern High Plains.
Project Relevance to Sustainable Agriculture
The region’s climate presents challenges for agricultural producers. Precipitation is limited (15-20 inches annually, 22% of evaporative demand). Gusting winds and intense rainfall accompany storms, which provide most of the region’s precipitation, but erode exposed soils. High rates of evaporation quickly diminish already limited soil water resources in this semi-arid climate.
Environmental and economic advantages of cover crops are widely reported (Clark, 2012; NRCS-Texas, 2013; Idowu and Grover, 2014). Reducing soil erosion, increasing water infiltration, and supplying organic matter and nutrients to successive crops are the most relevant benefits for the High Plains. Grass species are more adept in scavenging nutrients and produce larger volumes of residue that break down slowly, compared with broadleaf-type species (Clark, 2012). Legumes fix atmospheric nitrogen (N), some of which carries over to subsequent crops, and the legume residue has a lower carbon-to-N ratio, allowing N to be released more readily than from grasses (Clark, 2012). Although less common, the biofumigation and biodrilling potentials of brassica species are an attractive option for winter-annual ground covers (Clark, 2012). The five species selected for this trial were developed for or found to be adaptable to the Southern High Plains. The target uses of these cover crops serve the dual roles of stabilization and enhancement of soil plus their use as a grazeable spring forage. Teff response is evaluated as a summer-annual forage and as an indicator species for responses by other summer cash crops.
Even though the benefits of cover crops are well recognized, adoption has been minimal. Erratic weather patterns and unpredictable market conditions make producers hesitant to plant crops that may not generate immediate economic benefits (Butler and Muir, 2012; Idowu and Grover, 2014). There is a dogma among landholders in the Southern High Plains that cover crops extract too much water from the soil profile, hindering productivity of the successive cash crop. Managing the cover as a residue that improves soil productivity for longer-term benefits can help negate concerns.
Idowu and Grover (2014) proposed utilizing no- or minimal-tillage practices with cover crops to minimize concerns. Minimizing soil disturbance and maintaining ground coverage can improve soil health and promote water infiltration while reducing evaporative losses. Unger and Vigil (1998) reported that significant declines in soil water storage reduced subsequent crop productivity, but acknowledged that water use by cover crops could be counterbalanced by benefits of no- or reduced-tillage systems. This research investigated the interacting effects of irrigation and tillage with five cover crops on soil water depletion and productivity of the cover and subsequent forage crop to identify the most successful cover crop practices in the drought-prone Southern High Plains.
The research will provide knowledge to spark future development of a more sustainable agricultural landscape in West Texas. Producers will receive information that facilitates their decision-making on how to fit cover crops into their crop and livestock systems.
Literature Cited
Allen, V.G., C.P. Brown, R. Kellison, P. Green, C.J. Zilverberg, P. Johnson, J. Weinheimer, T. Wheeler, E. Segarra, V. Acosta-Martinez, T.M. Zobeck, and J.C. Conkwright. 2012. Integrating cotton and beef production in the Texas Southern High Plains: I. Water use and measures of productivity. Agronomy Journal 104:1625-1642.
Butler, T.J., and J.P. Muir. 2012. Perspective on forage legume systems for the tallgrass and mixed-grass prairies of the Southern Great Plains of Texas and Oklahoma. Crop Science 52:1971-1979.
Clark, A. (Ed.). 2007. Managing cover crops profitability, 3rd Edition. Sustainable Agriculture Network, Beltsville, MD.
HPWD. 2014. Water planning. High Plains Underground Water District. http://www.hpwd.org/water-planning/ (online, accessed 5 July 2014).
Idowu, J., and K. Grover. 2014. Principles of cover cropping for arid and semi-arid farming systems. Guide A-150. New Mexico State University Cooperative Extension Service. Las Cruces, NM.
Kelton, E. 2006-2007. Ranching in a changing land. In: Texas Almanac- The Source For All Things Texan Since 1857. http://www.texasalmanac.com/topics/agriculture/ranching-changing-land (online, accessed 11 Feb 2015).
Li, Y., V.G. Allen, J. Chen, F. Hou, C.P. Brown, and P. Green. 2013. Allelopathic influence of a wheat or rye cover crop on growth and yield of no-till cotton. Agronomy Journal 105:1581-1587.
NCBA. 2015. Beef industry statistics. National Cattlemen’s Beef Association. http://www.beefusa.org/beefindustrystatistics.aspx (accessed 11 Feb 2015).
NRCS-Texas. 2013. Winter cover crop species adapted to North-Central West Texas and Southwestern Oklahoma. Plant Materials Technical Note No: TX-PM-13-01. USDA, Knox City, TX.
Unger, P.W. and M.F. Vigil. 1998. Cover crop effects on soil water relationships. Journal of Soil and Water Conservation 53:200-207.
- Compare persistence and productivity of five winter cover crop species under four water and tillage treatment combinations for ability to conserve soil water and promote growth of summer forage.
- Compare residual effects of cover crops and winter management strategies on the productivity and nutrient status of a subsequent no-till, irrigated summer teff hay crop. Potential effects include nitrogen supplied by legumes, allelopathy from wheat and rye, and depletion of ground water resources.
- Compile research conclusions into fact sheets readily available to producers through the TAWC outreach program and to be demonstrated at Texas Tech Research Field Days.
Cooperators
Research
Site Description: Research was conducted at the Texas Tech Forage-Livestock Research Station located in New Deal, TX in northeast Lubbock County (33°45' N, 101°47' W; 993 m elevation). The research site is nearly level (0-1% slopes) with Pullman clay-loam soils containing a thick layer of CaCO3 (caliche) at 2-4 feet depth. Average monthly rainfall is 2.5 inches and potential evapotranspiration is 7 inches per month. The experimental area is equipped with an underground drip irrigation system with tapes on 40-inch centers, approximately 14 inches deep with injection emitters every 2 feet set to deliver 0.39 gallons per hour at 12.8 psi. A turbine water meter (Master Meter WNT-01, Fort Worth, TX) will track amount of irrigation applied. Irrigation was applied by hand, with a portable water tank, during winter months when the drip lines were drained to prevent freezing.
Experimental Design: A 50 foot x 180 foot area was allocated to this 2 x 2 x 6 factorial design, small-plot experiment, with three replicate blocks. Two irrigation treatments, dryland and irrigated, were applied across all blocks as a strip. The criterion for irrigation was to supplement rainfall up to no more than 1 inch per month of total water (rain + irrigation) delivery. The design within each block was a 2 x 6 factorial arranged in perpendicular strips, with two tillage treatments (minimal and no-till, arranged east-west) and six cover crop treatments (five species + fallow control; arranged north-south). Minimum tillage consisted of lightly disking no deeper than 2 inches once-over the field to break the surface crust. All plots were minimally tilled in Year 1 to ensure termination of the established perennial grasses. Cover crop treatments were arranged as strips, perpendicularly across tillage x irrigation combinations. Treatments compared rye (cv. Maton II), wheat (cv. TAM204), burr medic (cv. Armadillo), hairy vetch (cv. Vallana), rape-kale (cv. Bayou) and an unplanted fallow. Treatment assignments were not rerandomized in Year 2 to investigate cumulative effects. Inclusion of a winter fallow enabled treatment effects to be resolved from potential allelopathic effects of rye and wheat (Li et al., 2013). Rape-kale stands were monitored for fungal diseases caused by potential Leptosphaeria maculans buildup in the soil. The irrigation-by-tillage comparison tested the interactive nature of soil disturbance and water supply on productivity and N uptake of the five cover species and summer hay crop. Teff (cv. Tiffany) was planted after cover-crop termination in May of each year to measure the response to cover crop treatments. All teff plots received similar amounts of irrigation during May-August such that irrigation supplemented rainfall up to 5 inches per month of total water (rain + irrigation) delivery.
Project Set-Up: A tractor-mounted power-tiller was used to prepare seedbeds in early September before cover crops were planted in Year 1. Cover crops were drilled in 7-inch row spacing using a Tye drill in mid-September (Table 1). In Year 1, one access tube for a Dynamax PR2 Profile Probe System (Dynamax Inc., Houston, TX) soil moisture sensor was installed in each subplot in the central block (block 2). Teff was drilled the following May (two-three weeks post-termination of covers to minimize allelopathic effects [Clark, 2012]). This process was repeated in Year 2 except that the minimum-till blocks were lightly disked.
Data Collection:
Time trends in crop cover were monitored photographically by collecting images of three, random, 1 ft x 3 ft quadrat locations in each subplot before harvest using an Olympus TG-820 digital camera (Olympus Optical Co., London, UK) mounted on a PVC monopod at 5 feet high. All images were processed in batches (species x sampling date) using unique macros developed for ImageJ® software (version Fiji; imagej.nih.gov).
Canopy height, morphological growth stage, and extent of nodulation (for burr medic and hairy vetch, on a 1-5 visual scale) were recorded in five locations within each plot before harvest. Forage mass of the cover crops was determined from three, 1 ft x 3 ft hand samples clipped to a 3-inch stubble height. A flail harvester with 40-inch cutting head was used to harvest an 8-foot strip to 3-inch stubble height from each teff plot in Year 1. Plot weight was recorded before collecting a 500 g subsample to determine dry weight and N concentration. Due to mechanical problems in Year 2, the teff forage mass was determined by hand clipping one square foot sample from each plot. Unsampled biomass was removed from all harvested experimental areas to maintain vegetative growth.
Volumetric soil water content was monitored weekly throughout the experiment (except in time of equipment malfunctions or inclement weather) with the Profile Probe at 4, 8, 12, 16, 24, and 40 inch depths. Three soil samples were randomly collected from each irrigation x tillage subplot in each block each September. Around May 1, similar samples were collected in blocks 1 and 3 after cover crops have been terminated. Water content in all subsubplots in block 2 was determined using the Profile Probe.
Treatment comparisons were made with mixed-model analysis of variance, with additional analyses within season where appropriate. The irrigation-strip design across blocks disallows statistical testing of the main irrigation effect; however, all interactions with tillage and cover crop treatments were tested.
Irrigation and Rainfall:
As illustrated in Figure 1, there was a significant interaction between cover crop species and year for irrigation applied from September to April (P = 0.02). Although there does not appear to be a clear trend in irrigation required by the different crops, more irrigation was applied during this period in Year 2 since rainfall was lower than in Year 1 (Table 2). The teff received 11.69 inches of irrigation in Year 1 and 8.97 inches in Year 2 to supplement summer rainfall (Table 2).
Table 2. Seasonal rainfall total for Lubbock, TX.
Cover crop yield:
Greater cumulative yields were observed in Year 1 than Year 2, and the forage species responded differently within each year (P < 0.01; Fig. 2). In Year 1, the greatest cumulative yields were observed in rape-kale (irrigation or dryland management) and irrigated rye, follow by dryland rye. The remaining forages produced negligible yields. In Year 2, the greatest cumulative yields were produced by irrigated rye, followed by irrigated hairy vetch and rye, then wheat (both irrigation regimes) and dryland hairy vetch. The burr medic and rape-kale stands failed in Year 2. These differences are further illustrated in Figure 3.
Timing and volume of precipitation events were critical to the success of the crops in each year. Even though burr medic did produce a decent ground cover in Year 1, the crop never accumulated significant yields that could be harvested. Hairy vetch performed much greater in Year 2, which may indicate a high level of dormant seed in the material that was planted in Year 1. It was surprising that rape-kale performed so well in Year 1 then failed in Year 2. Although the crop germinated, it did not persist under the colder, drier weather found throughout the fall and early winter in Year 2. Irrigated rye was consistently the highest yielding crop in both years of the study, while dryland rye followed closely behind. The wheat crop produced measurable forage mass in both years but it never competed with the irrigated rye. The wheat crop was suppressed in Year 1 by grazing from wild animals and delayed regrowth after an extended period under snow (over 7 days). There was no indication of allelopathy in the rye or wheat crops for this study.
Cover crop crude protein:
Crude protein concentration of the cover crops did not differ by harvest, forage, or their interaction in Year 1 (P > 0.32; Fig. 4). However, there was a significant interaction between harvest and forage in Year 2 (P = 0.02; Fig. 4). Not surprisingly, hairy vetch (only harvested at the second harvest) had the greatest crude protein concentration. Crude protein was greater in Harvest 1 than Harvest 2 for both small grains, but the decline was greater in rye. The wheat was at a similar average Feekes growth stage for both harvests (8.5 vs. 9.0), while the rye was much more mature at the second harvest (4.3 vs. 9.0).
Ground cover:
Greater ground cover was observed in Year 1 than Year 2 (Fig. 5; P > 0.01). Likewise, greater cover was seen in Harvest 1 than Harvest 2 (Fig. 5; P > 0.01). This was most likely a result of the greater cover from the rape-kale that was only harvested in Year 1 as the stand failed in Year 2 (Fig. 5; P > 0.01). Rape-kale has wide, large leaves which translates to a higher leaf are index (LAI; 2.13) than rye (0.66) or wheat (0.19) (P > 0.01).
Nodulation:
The interaction of tillage regime and forage species affected the visual nodulation score (P = 0.01; Fig. 6 and Fig. 7). Nodulation was much greater in hairy vetch than burr medic. Tillage reduced nodulation in hairy vetch, but yielded no significant effect on burr medic. The harsh climate in West Texas provides a challenging environment for Rhizobium bacteria. Different inoculants should be evaluated for burr medic to attempt to improve nodulation.
Teff yield:
In Year 1, competition from rape-kale decreased teff cumulative yields compared to the other winter cover crops (P < 0.01; Fig. 8 and Fig. 9). Strong northern winds prevented chemical termination of the cover crops in the first year (cotton was planted directly south of the experimental area). Close mowing did not sufficiently terminate the rape-kale, which continued to grow throughout the first summer harvest period. Only the winter tillage regime impacted the teff cumulative yield in Year 2 (P < 0.01; Fig. 8). This was most likely a consequence of greater water loss in the tilled plots in the top foot of soil (Fig. 13).
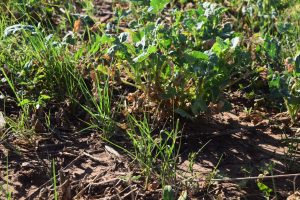
Teff crude protein:
Teff crude protein concentration was significantly affected by year and harvest (P < 0.01; Fig. 10). Scheduling prevented timely hay harvests in Year 2, consequently resulting in more mature forage that was lower in protein. Late season rains delayed the second harvest in both years, again resulting in lower crude protein content due to forage maturity.
Gravimetric water content:
Gravimetric water content (GWC; 0-10” deep) was affected by time of year (P < 0.01). These measurements only represent a single point in time and were only collected from irrigation x tillage subplots. A more detailed evaluation of water use in winter management schemes was made with volumetric water content. Still, GWC increased from September 2015 to May 2016. The GWC decreased during the same time period of the second year but this was most likely a consequence of heavy rainfall before sampling in September 2016 and dry Spring 2017.
Volumetric Water Content:
The winter irrigated plots has significantly greater volumetric water content (VWC) than the winter dryland plots at the top four depths from 4 to 24 inches (P < 0.01; Fig. 12). Likewise, tilling the plots before planting the winter crop reduced VWC at the top four depths (P < 0.02; Fig. 13). Winter cover crops only affected VWC at the 4 and 24 inch depths (P < 0.02; Fig. 14). The legume plots maintained a higher VWC than the rape-kale and winter fallow plots at 4 inches. Rye and wheat were intermediate to, though not different from, these two groups. At 24 inches deep, the wheat plots were the lowest but not significantly lower than rye or hairy vetch. The results are interesting but there does not appear to be a logical reason for the results. Increasing the sampling locations to another block would help explain these findings. Most importantly, the use of a winter cover crop did not significantly reduce the soil VWC at any depth. In fact, VWC was actually increased at the 4 inch depth (P < 0.02; Fig. 15).
Educational & Outreach Activities
Participation Summary:
Preliminary results from this project were presented at the following seminars and professional meetings:
- 2017 American Forage and Grassland Council Annual Meeting (January 2017)
- Texas Tech University, Department of Plant and Soil Science Seminar Series (February 2017)
- PhD Defense Seminar for Lisa Baxter (Texas Tech University; March 2017)
- Southern SARE Young Scholar Enhancement Poster (September 2017)
- To multiple state and international tour groups at the New Deal Research Farm
Final results from this project will be compiled into a manuscript for Crop, Forage, and Turfgrass Management. An educational bulletin will be compiled in Fall 2017 to be provided through the Texas Alliance for Water Conservation (TAWC) for producers in West Texas.
Project Outcomes
Final outcomes from this research include:
- Winter cover crops did not require more irrigation than the winter fallow.
- Cumulative yields from the winter cover crops were most consistent for rye. As expected, irrigated rye yielded greater than dryland rye in both trial years.
- Hairy vetch had the greatest crude protein concentration in Year 2.
- Ground cover was greater in Year 1 when rainfall was higher. Rape-kale had greater ground cover than the small grains.
- Visual nodulation scores were greatest in no-till hairy vetch.
- Competition from rape-kale reduced cumulative teff yields in Year 1.
- Switching to no-till reduced soil water loss and increase cumulative teff yields in Year 2.
- Crude protein of the subsequent teff crop depended more on timely harvests than winter management schemes.
- Most importantly, use of a winter cover crop did not decrease soil water reserves.
Future research should delve more deeply into the water use of these winter management strategies and the use of cover crop mixtures (specifically rye with hairy vetch).
The use of winter annual cover crops are very promising for this region. We can minimize producer concerns about water use by winter cover crops and the impact on the subsequent cash crop.
Overall recommendation: West Texas producers should plant rye as a cover crop. Dryland rye produces a modest yield, even in a dry year, but light irrigation should be used to ensure yield if the winter grazing is desired.